Abstract
Background: Increased systemic oxidative stress is considered as an important risk factor for prostate cancer occurrence; however, the relationship between impaired redox homeostasis of prostate tissue and aging remains unclear.
Objective: In our study, we hypothesized that age-related deterioration of redox homeostasis in prostate tissue may be considered as a predisposing factor for prostate cancer occurrence.
Methods: Sprague–Dawley rats were divided into two groups as young control (5 months) and naturally aged (24 months). We investigated the levels of oxidant and antioxidant parameters in prostate tissue.
Results: Advanced oxidation protein products, protein carbonyl, non-protein thiol and lipid hydroperoxides levels of aged rats were significantly higher than in the young control rats (p < 0.01, p < 0.05, p < 0.001, p < 0.05, respectively). Additionally, antioxidant activity of Cu-Zn-superoxide dismutase in elderly group was significantly lower than young controls (p < 0.05).
Conclusions: We suggest that increased non-protein thiol levels found in aged rats may prevent further dissemination of oxidative protein damage. We also propose that the increased levels of oxidative protein damage markers and decreased Cu-Zn superoxide dismutase activity in aged prostate may be considered as a predisposing factor for prostate cancer. Further studies are warranted to clarify all these oxidative changes as initiation factors for prostate cancer in the association of aging with prostate cancer.
Introduction
It is observed that gradually higher proportion of the cancer-related deaths occur in the developing countries. Considering the range of cancer types in Turkey, lung cancer is the most common type of cancer among men and prostate cancer comes second with an incidence of 24.33 per 100 thousand [Citation1]. Besides socio-economic factors, one of the most prominent attributes of developing countries is the dissimilarity of their age-dependent demographic structure. In parallel with population growth, the reversal of population pyramid and the rapid increase of the population above 65 lead to various problems.
Reactive oxygen species (ROS) and reactive nitrogen species (RNS) are not only byproducts of the normal cellular metabolism, but they also play important roles in cell signaling [Citation2]. However, when the levels of ROS and RNS increase, cells are exposed to oxidative stresses, which activate a variety of mechanisms to allow them to cope with these changes [Citation2]. Enhanced extent of oxidative stress has also been linked to the pathogenesis of age-related diseases including prostate cancer [Citation3]. Aberrant ROS signaling may play an important role in the development and progression of this malignancy [Citation4]. Chronic increases in ROS over time are known to induce somatic mutations and neoplastic transformation [Citation4]. Aging represents a major risk factor for the occurrence of prostate cancer as well as benign prostate hypertrophy; however, redox regulation mechanisms which are responsible for this inter-relationship remain unclear. Increased oxidative damage to cellular macromolecules in prostate tissue has been observed in aging as well as during the development of the prostatic malignancy [Citation3,Citation4].
The objective of the current study was to examine the involvement of protein oxidation in the mechanisms of malignant transformation since the understanding of risk factors for prostate cancer has a practical importance for public health, genetic and nutritional education, and chemoprevention. In the present study, we hypothesized that age-related impairment of redox homeostasis in prostate tissue may be considered as a predisposing factor for prostate cancer occurrence. Hence, we investigated the prostatic tissue levels of oxidative damage parameters such as advanced oxidation protein products (AOPP), protein carbonyl groups (PCO), protein thiol groups (P-SH), lipid hydroperoxides (LHP), besides the antioxidant capacity biomarkers.
Materials and methods
Chemicals and apparatuses
Unless otherwise specified, the chemicals were of the highest analytical grade available. All chemicals and reagents were purchased from Merck (Darmstadt, Germany) and Sigma-Aldrich (St Louis, MO). Deionized water was used in the analytical procedures. All reagents were stored at +4 °C. The reagents were maintained in equilibrium at room temperature for 0.5 h before use. Centrifugation procedures for the analysis of various oxidative stress markers were performed at +4 °C with a Sigma 3–18 KS centrifuge (SIGMA Laborzentrifugen GmbH, Osterode am Harz, Germany). Oxidative damage biomarker profiles of prostate tissue were analyzed by spectrophotometric methods with Biotek Synergy™ H1 Hybrid Multi-Mode Microplate Reader (BioTek US, Winooski, VT).
Experimental animals
All protocols for experiments were approved by the Animal Care and Ethics Committee of Istanbul University. They were housed in a temperature controlled room (25 ± 5 °C) with 12-h light–dark cycles. All rats were fed with a normal laboratory diet nutrients rich pellets containing total energy as fat, protein and carbohydrates, and had free access to drinking water. The study was carried out with 18 male Sprague–Dawley rats. Experimental animals were examined into two groups. Group 1: Young control (YC) rats (n = 8; 5 months old), Group II: Naturally aged (NA) rats (n = 10; 24 months old).
Preparation of tissue homogenates and samples
Richie et al. had previously showed that the prostate tissue of 24-months-old rates as naturally aged rates are appropriate to investigate the age-related oxidative changes in prostate tissue [Citation3].The prostate tissue samples, which were obtained from the rats under deep anesthesia with intraperitoneally administered sodium pentothal, were washed in cooled 0.9% NaCl and placed on an ice-cold plate. The samples were then immediately frozen in liquid nitrogen until they were homogenized. Tissue samples were homogenized manually in homogenizing buffer (100 mM KH2PO4–K2HPO4, pH 7.4, plus 0.1% [w/v] digitonin) to made%10 homogenates.
Homogenates were centrifuged at 5000 × g for 10 min, and various analytes of the supernatant fraction were examined [Citation5].
The supernatant fractions of tissue homogenates were divided into aliquots (one for each assay) and immediately stored at −80 °C for the analysis of oxidative stress markers such as PCO, AOPP, P-SH, total thiol (T-SH), non-protein thiol (NP-SH), LHP, Cu-Zn-superoxide dismutase (Cu-Zn-SOD), and ferric reducing/antioxidant power (FRAP).
Analytical methods
The details of investigated oxidative stress-related biomarkers are given in .
Table 1. Prostate tissue oxidative stress-related parameters analyzed.
Assays of protein oxidation markers
Protein carbonyl groups
We performed PCO analysis as described by Reznick and Packer [Citation6] with some of the slight modifications in order to apply small volumes of supernatant samples. PCO groups react with 2,4-dinitrophenylhydrazine (DNPH) (100 μL supernatant: 400 μL DNPH) to generate chromophoric dinitrophenylhydrazones. DNPH was dissolved in HCl, and after DNPH reaction, proteins were precipitated with an equal amount of 20% (w/v) trichloroacetic acid and washed three times with 400 µL of an ethanol/ethyl acetate mixture (1:1). Washing was done by mechanical disruption of pellets in the washing solution and re-pelleting by centrifugation at 3000 × g for 5 min. Finally, the protein precipitates were dissolved in a 200-µL 6 M guanidine–HCl solution and the related absorbance were measured at 360 nm using the molar extinction coefficient of DNPH, ε = 22 000 M−1cm−1 The coefficients of intra- and inter-assay variations for modified carbonyl assay were 4.1% (n = 8) and 8.1% (n = 8), respectively. The PCO–BSA positive control and untreated 7BSA were both prepared according to the method of Lenarczyk et al. [Citation7] and tested according to the PCO assay protocol.
Advanced oxidation protein products
AOPP concentrations was assessed by the modification of Hanasand's method [Citation8]. Samples were prepared in the following way: 10 μL of supernatant, 40 μL of phosphate buffered saline (PBS), 200 μL citric acid solution (20 mmol/L) were mixed in microplate. After 1 minute, 10 μL of 1.16 M potassium iodide was added to the microplate well, the absorbance of the reaction mixture was read at 340 nm against reagent blank. The chloramine-T absorbance at 340 nm being linear within the range of 0–100 μmol/L. AOPP concentration was expressed as micromoles per liter of chloramine-T equivalents. All readings were performed within two minutes after potassium iodide addition for avoiding uncontrollable color development which leads to possible deviation from the chloramine standard curve. The coefficients of intra- and inter-assay variations were 1.5% (n = 8) and 2.2% (n = 8), respectively. The AOPP–BSA positive control and untreated BSA were both prepared in vitro and tested according to the AOPP assay protocol [Citation9].
Protein thiol groups
Redox-sensitive biomarkers, prostatic tissue T-SH, NP-SH, and P-SH concentrations, were determined by using 5,5-dithiobis (2-nitrobenzoic acid) (DTNB) as described by Sedlak and Lindsay [Citation10]. We revised with some modifications from previously described T-SH method in order to apply small volumes of supernatant samples. A part (20 μL) of the supernatant was mixed in 1.5-mL test tubes with 400 μL of 0.2 M Tris buffer, pH 8.2, and 20 μL of 0.01 M 5,5-dithiobis 2-nitrobenzoic acid (DTNB) for the determination T-SH groups. NP-SH samples were assayed in the following way: 20 μL of supernatant were mixed in 400 μL of 50% TCA. The tubes were mixed intermittently for 10 min and centrifuged at approximately 3000 × g for 15 min. Supernatant fractions were assayed as T-SH. The absorbance values of the resulting samples were read at 412 nm wavelength against reagent blank. The value of molar extinction coefficient of thiol (–SH) groups at wavelength 412 nm is approximately ε = 13 100 M−1cm−1. The PSH groups were calculated by subtracting the NP-SH from T-SH. The coefficients of intra- and inter-assay variations were 1.4% (n = 8) and 3.5% (n = 9), respectively.
Assay of lipid peroxidation marker
Lipid hydroperoxides
LHPs levels were determined spectrophotometrically by the method that uses oxidation of ferrous ions with xylenol orange FOX2 (Ferrous Oxidation with Xylenol orange, version 2) [Citation11]. LHPs oxidized ferrous to ferric ions selectively in dilute acid, and the amount resultant ferric ions were determined by using ferric-sensitive dye, which represents the concentration of LHPs. Xylenol orange binds to ferric ions with high selectivity to produce a colored (blue–purple) complex. Fifth microliters aliquots of supernatant were transferred into microcentrifuge reaction vials. FOX2 reagent (950 μL) was then added, and the samples were mixed on vortex. After incubation with FOX2 reagent at room temperature for 30 min, the samples were centrifuged at 3000 × g at 20 °C for 10 min, the resulting supernatant was carefully transferred into microplate wells, and absorbance was read at 560 nm against reagent blank The coefficients of intra- and inter-assay variations were 2.3% (n = 8), and 2.5% (n = 11), respectively.
Estimation of antioxidant capacity
Ferric reducing/antioxidant power (FRAP) assay
Prostatic tissue antioxidant status was evaluated using FRAP assay [Citation12]. The modified FRAP assay uses antioxidants as reductants in a redox-linked colorimetric method. In this assay, at low pH a ferric-2,4,6—tripyridyl-s-triazine (FeIII –TPTZ) complex reduced to the ferrous form, which is blue-colored and monitored by measuring the change in absorption at 593 nm. The change in absorbance is directly proportional to the reducing power of the electron-donating antioxidants present in the prostatic tissue. Three hundred mmol/L of acetate buffer (pH 3.6); 10 mmol/L 2,4,6-tri-pyridyl-s-triazine (TPTZ) in 40 mmol/L HCl and 20 mmol/L FeCl3.6H2O in the ratio of 10:1:1 give the working FRAP reagent. Fe(II) standards were used. Fe(II) (1000 μmol/L) is equivalent to 1000 μmol/L of FRAP. 750 μL of working FRAP reagent was mixed with 25 μL supernatant or standard in a test tube. The absorbance at 593 nm is read against reagent blank. The absorbance change is translated into a FRAP value in mM by relating the change of absorbance at 593 nm of test sample to that of a standard solution of known FRAP value (3–0.375 mM). The coefficients of intra- and inter-assay variations for modified FRAP assay were 1.2% (n = 8) and 2.4% (n = 8), respectively.
Cu-Zn-superoxide dismutase activity
Cu-Zn-SOD (EC 1.15.1.1) activity was measured by using the method of Sun and Oberley with some of the slight modifications [Citation13]. This method involves the inhibition of nitrobluetetrazolium (NBT) reduction, with xanthine oxidase (XO) used as a superoxide generator. Enzyme activity was determined by measuring the inhibition rate of substrate hydrolysis in the assay mixture containing 0.3 mmol/L xanthine, 0.6 mmol/L Na2EDTA, 150 μmol/L NBT, 400 mmol/L Na2CO3, 1g/L BSA. The pH value of the assay mixture should be adjusted to pH 10.2. Nine hundred seventy-two microliters assay mixture and 13-µL XO (167 U/L) are added into 25-µL supernatant sample. At the end of the 20-min incubation period, 250 µL 0.8 mmol/L CuCl2 was added to the well in order to terminate reaction. The final absorbance was read at 560 nm against reagent blank. Percent inhibition rate was calculated according to the following equation:
One unit of Cu-Zn-SOD is defined as the amount of enzyme needed to exhibit a 50% dismutation of superoxide radical anion. The coefficients of intra- and inter-assay variations for modified Cu-Zn-SOD assay were 3.9% (n = 8) and 4.8% (n = 8), respectively.
Estimation of total protein content
Protein determinations were carried out fluorometrically by Qubit analyzer (Invitrogen, Carlsbad, CA).
Estimation of total prostate specific antigen
Prostate specific antigen (PSA) concentrations of the tissue samples were determined by using an enzyme-linked immunosorbent assay detection kit (MyBioSource, Inc., San Diego, CA).
Data processing
Statistical calculations were performed using SPSS 20 (ver.20.0, SPSS, Chicago, IL). Prostate tissue oxidative damage biomarker levels in two groups of Sprague–Dawley rats were compared using the Mann–Whitney U-test. Data are expressed as the mean ± SEM of the mean for each group.
Results
We observed hypertrophic prostate tissue in NA rats when we compared them to YC rats during the surgical removal of prostate tissue. The protein oxidation biomarker profile of YC and NA rats was given in . shows the effect of aging on LHP formation. Antioxidant capacity biomarkers in prostate tissue of YC and NA rats were given in .
Figure 1. Protein oxidation biomarkers in prostate tissue of naturally aged rats (NA; n = 10), and their corresponding young controls (YC; n = 8). (A) PCO, (B) AOPP, (C) P-SH. Results are expressed as mean ± S.E.M. Data are statistically different between groups, *p < 0.05, **p < 0.01; PCO, protein carbonyl groups; AOPP, advanced oxidation protein end products; P-SH, protein thiol groups; pr, protein.
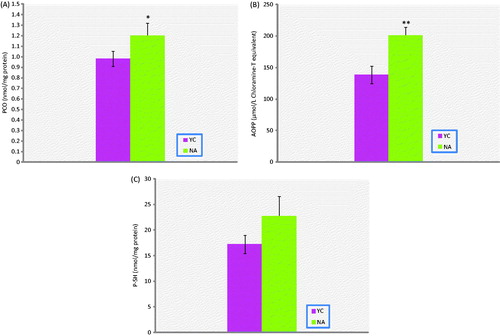
Figure 2. Lipid peroxidation status in prostate tissue of naturally aged rats (NA; n = 10) and their corresponding young controls (YC; n = 8). Results are expressed as mean ± S.E.M. Data are statistically different between groups, *p < 0.05; LHP, lipidhydroperoxides.
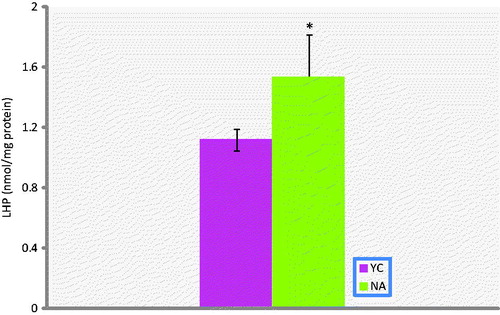
Figure 3. Antioxidant capacity biomarkers in prostate tissue of naturally aged rats (NA; n = 10) and their corresponding young controls (YC; n = 8). (A) FRAP, (B) Cu-Zn-SOD, (C) TSH, (D) NP-SH. Results are expressed as mean ± SEM. Data are statistically different between groups, *p < 0.05, ***p < 0.001; FRAP, ferric reducing/antioxidant power; Cu-Zn-: Cu-Zn-superoxide dismutase; T-SH, total thiol groups; NP-SH, non-protein thiol groups.
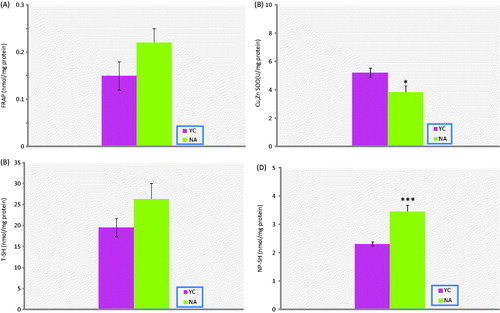
AOPP, PCO, NP-SH and LHP levels of aged rats were significantly higher than in the young rat group (p < 0.01, p < 0.05, p < 0.001, p < 0.05, respectively). On the other hand, T-SH, P-SH and FRAP levels were all found to be not different. In addition, Cu-Zn-SOD activities in prostate tissue of experimental group were significantly lower than the corresponding young controls (p < 0.05).
PSA levels were not found to be different between the two groups of rats (data not shown).
Discussion
Identifying the oxidation of proteins is critical for cellular homeostasis, and it could potentially provide important information concerning molecular mechanisms underlying the development and progression of aging and age-related disorders linked to oxidative stress. The association of aging with prostate cancer is undisputable as well as the association of aging with oxidative stress. Nevertheless, supportive evidence linking an increase in oxidative stress with prostate cancer is still scarce [Citation14]. No established data or mechanistic explanations exist in current literature that focuses on how aging process induces oxidative protein damage with the increase of PCO and AOPP formation in prostate tissue of aged subjects.
In the current study, we found that the prostate tissue AOPP, PCO, and NP-SH levels of aged rats were significantly higher than in the young rat group. NP-SH consists of mostly reduced glutathione, cysteine, coenzyme A and other thiols [Citation15]. The direct organo or cytoprotective effects of NP-SH groups were demonstrated biochemically in vivo against ROS in aging [Citation5,Citation16]. We suggest that increased NP-SH levels found in aged rats may point to an adaptive reaction to oxidative protein damage, reflecting AOPP and PCO overproduction. AOPP contains a variety of oxidation products such as PCO, dityrosine, pentosidin and due to this, it is thought that the increase in protein carbonyl levels may cause a partial increase in AOPP [Citation5,Citation8]. It is also known that the tyrosine remnants in proteins of prostate tissue play a role in signal transduction [Citation17]. We thought that the dityrosine fraction in increased AOPP content is related to the impairment of signal transduction mechanisms in prostate tissue of aged subjects. PCO groups may be formed by the formation of adducts between some amino acid residues and the products of lipid peroxidation [Citation18]. At the same time the high level of LHP–PCO couple in prostate tissue of aged rats demonstrates the activation of the formation of adducts between some amino acid residues and the products of lipid peroxidation.
Total antioxidant capacity determinations are simple, inexpensive, and able to evaluate the capacity of known and unknown antioxidants and their additive, synergistic and/or antagonistic actions, in chemical and biological systems. However, different TAC assays are poorly correlated with each other, since each of the total antioxidant capacity assay is sensitive to a particular combination of the compounds, but it also excludes many others [Citation19]. FRAP assay measures the ferric-reducing ability of the biological sample which is using ferric iron as a redox sensor [Citation12]. Relative contribution of the total antioxidant capacity to age-related oxidative stress is not well defined. While some studies demonstrated that the total antioxidant capacity decreases in aged rats [Citation20], and others show that it is unchanged [Citation21]. Additionally, this discrepancy may also be explained by the fact that FRAP assay measures the reducing power of biological sample, including reductants that are not classical biological antioxidants. Lack of measurement of the contribution of thiols groups and the acidic pH represents the main limitation of the method. The current study found that the tendency of NP-SH to decrease in prostate tissue of aged rats.
Previous studies have been shown that the erythrocyte Cu-Zn-SOD activities in patients with carcinoma of prostate are increased [Citation22]. Decreased activities of Cu-Zn -SOD would be expected to increase the susceptibility of prostate tissue to oxidative injury in aged rats. On the other hand, there are no established data or explanations in the current literature about why the prostate tissue Cu-Zn -SOD activities of aged rats are lower than in young controls and how high erythrocyte activity is associated with decreased Cu-Zn -SOD in prostate.
In prostate cancer, oxidative stress, an innate key event characterized by supraphysiological ROS concentrations, has been identified as one of the hallmarks of the aggressive disease phenotype. Specifically, oxidative stress is associated with prostate cancer development, progression and the response to therapy [Citation23]. There are no current experimental evidences that analyze redox homeostasis of prostate proteins in human and rodents in the current literature, so we propose that the increased levels of oxidative protein damage markers and decreased Cu-Zn -SOD dismutase activity in aged prostate may be considered as a predisposing factor for prostate cancer. Increased NP-SH levels found in aged rats may prevent further dissemination of oxidative damage, reflecting advanced oxidation protein products and protein carbonyl overproduction. Additional studies are required to clarify the potential contributions of all these oxidative changes as predisposing factors in the association of aging with prostate cancer risk.
Declaration of interest
This study was supported partially by TUBITAK (2209) research grant and Istanbul University research grant (UDP-34391). U. Ç., P. A. and S. A. were the principal investigators and mentors who took primary responsibility for the research. K. Y., D. U., T. C., T. O., M. M., A. I. K. also took responsibility for housing, care, and maintenance of experimental animals and made contributions to work at the laboratory. K. Y. performed the statistical analysis and prepared the figures. U. Ç., P. A., K. Y., and A. K. wrote the paper principally, U. Ç., A. K. made contributions to the first draft. All the authors read and approved the final manuscript. The authors declare that there have no competing interests with respect to the contents of this article.
References
- Yılmaz HH, Yazıhan N, Tunca D, et al. Cancer trends and incidence and mortality patterns in Turkey. Jpn J Clin Oncol 2010;41:10–16
- Gupta-Elera G, Garrett AR, Robison RA, O'Neill KL. The role of oxidative stress in prostate cancer. Eur J Cancer Prev 2012;21:155–62
- Richie JP Jr, Das A, Calcagnotto AM, et al. Age related changes in selenium and glutathione levels in different lobes of the rat prostate. Exp Gerontol 2012;47:223–8
- Khandrika L, Kumar B, Koul S, et al. Role of oxidative stress in prostate cancer. Cancer Lett 2009;282:125–36
- Aydın S, Yanar K, Atukeren P, et al. Comparison of oxidative stress biomarkers in renal tissues of D-galactose induced, naturally aged and young rats. Biogerontology 2012;13:251–60
- Reznick AZ, Packer L. Oxidative damage to proteins: spectrophotometric method for carbonyl assay. Methods Enzymol 1994;233:357–63
- Lenarczyk M, Cohen EP, Fish BL, et al. Chronic oxidative stress as a mechanism for radiation nephropathy. Radiat Res 2009;171:164–72
- Hanasand M, Omdal R, Norheim KB, et al. Improved detection of advanced oxidation protein products in plasma. Clinica Chimica Acta 2012;413:901–6
- Zeng JH, Zhong ZM, Li XD, et al. Advanced oxidation protein products accelerate bone deterioration in aged rats. Exp Gerontol 2014;50:64–71
- Sedlak J, Lindsay RH. Estimation of total, protein-bound, and nonprotein sulfhydryl groups in tissue with Ellman's reagent. Anal Biochem 1968;25:192–205
- Wolff SP. Ferrous ion oxidation in presence of ferric ion indicator xylenol orange for measurement of hydroperoxides. Methods Enzymol 1994;233:182–9
- Benzi IFF, Strain JJ. Ferric reducing/antioxidant power assay.direct measure of total antioxidant activity of biological fluids and modified versionfor simultaneous measurement of total antioxidant power and ascorbic acid concentration. Methods Enzymol 1999;299:15–27
- Sun Y, Oberley LW, Li Y. Simple method for clinical assay of superoxide dismutase. Clin Chem 1988;34:497–500
- Minelli A, Bellezza I, Conte C, Culig Z. Oxidative stress-related aging: a role for prostate cancer? Biochim Biophys Acta 2009;1795:83–91
- Nagy L, Nagata M, Szabo S. Protein and non-protein sulfhydryls and disulfides in gastric mucosa and liver after gastrotoxic chemicals and sucralfate: possible new targets of pharmacologic agents. World J Gastroenterol 2007;13:2053–60
- Çakatay U, Telci A, Kayalì R, et al. Relation of oxidative protein damage and nitrotyrosine levels in the aging rat brain. Exp Gerontol 2001;36:221–9
- Cen B, Mahajan S, Wang W, Kraft AS. Elevation of receptor tyrosine kinases by small molecule AKT inhibitors in prostate cancer is mediated by Pim-1. Cancer Res 2013;73:3402–11
- Çakatay U, Aydın S, Yanar K, Uzun H. Gender-dependent variations in systemic biomarkers of oxidative protein, DNA, and lipid damage in aged rats. Aging Male 2010;13:51–8
- Fraga CG, Oteiza PI, Galleano M. In vitro measurements and interpretation of total antioxidant capacity. Biochim Biophys Acta 2014;1840:931–4
- Sivonová M, Tatarková Z, Duracková Z, et al. Relationship between antioxidant potential and oxidative damage to lipids, proteins and DNA in aged rats. Physiol Res 2007;56:757–64
- Nakamura YK, Omaye ST. Age-related changes of serum lipoprotein oxidation in rats Life Sci 2004;23:1265–75
- Surapaneni KM, Venkata GR. Lipid peroxidation and antioxidant status in patients with carcinoma of prostate. Indian J Physiol Pharmacol 2006;50:350–4
- Paschos A, Pandya R, Duivenvoorden WC, Pinthus JH. Oxidative stress in prostate cancer: changing research concepts towards a novel paradigm for prevention and therapeutics. Prostate Cancer and Prostatic Diseases 2013;16:217–25