Abstract
Hot water-soluble crude polysaccharides were extracted from the rhizomes of wild turmeric, Curcuma aromatica Salisb. (Zingiberaceae), using dry grinding, boiling water extraction, and then ethanol precipitation. The crude polysaccharide extract was then fractionated by DEAE-cellulose ion exchange column chromatography, and subsequently further purified by Superdex G-200 gel filtration column chromatography, giving two relatively abundant polysaccharide fractions, called P11 and P21, and a much less common fraction P22 obtained in insufficient amounts for further analysis. The two main polysaccharide fractions were evaluated for monosaccharide composition by acid hydrolysis and high performance liquid chromatography (HPLC), whilst the molecular weight and functional groups were determined by gel permeable chromatography (GPC) and FT-IR, respectively. Fractions P11 and P21 were found to be polyxyloses with molecular weight-averages of 469,171 and 157,665 Da, respectively. P11 (100 μg/mL) could significantly induce human gingival fibroblast cells proliferation by 30%, while P21 (100 μg/mL) could significantly inhibit gingival fibroblast cells proliferation by 92%. The in vitro human primary gingival fibroblast cell proliferation in cell culture at a concentration of 100 μg/mL.
Introduction
Important and biologically active carbohydrates are usually those with high molecular weights, i.e., polysaccharides. Most natural polysaccharides are somewhat ubiquitous in living organisms and can be isolated from plants, animals and microorganisms, but each polysaccharide is different in individual characteristics including bioactivities. This variation occurs between species, individuals within a species and between tissues or cells within an individual (CitationLindhorst, 2003). Accordingly, there is much interest in studying polysaccharides, especially the biological activities of purified polysaccharides which are extracted from plants.
This interest is largely due to the observations that previous studies have indicated that plant poly-saccharides in general have many biological activities, including immunomodulatory, antitumor and antiviral and microbial activities, as well as thickeners and stabi-lizers for food. For example, polysaccharides extracted from Dioscorea nipponica Makino (Dioscoreaceae) (CitationLuo, 2008) and Gynostemma pentaphyllum Makino (Cucurbitaceae) (CitationWang & Luo, 2007) have antioxidant activities, whilst those from Alpinia galangal L. (Zingiberaceae) (CitationBendjeddou et al., 2003), Aconitum carmichaeli Debx. (Ranunculaceae) roots (CitationZhao et al., 2006) and Dendrobium huoshanense Z. Z. Tang & S. J. Cheng (Orchidaceae) stems (CitationZha et al., 2007) have immunostimulating activities. Finally, polysaccharides extracted from the pollen of Brassica napus L. (Brassicaceae) and Phoenix dactylifera L. (Arecaceae) (CitationYang et al., 2007a, Citation2007b) have anticancer and antitumor activities, respectively.
The glycoprotein extracted from Aloe barbadensis Mill (Liliaceae) (also known as Aloe vera) was reported to be able to increase human gingival fibroblast cell proliferation at a concentration of 50 and 100 μg/mL, while at 600 μg/mL it decreased the proliferation of cells (CitationThunyakitpisal et al., 2004). Previously another 29 kDa dimeric glycoprotein of two 14 kDa subunits from this gel extract was reported to have in vitro cell-proliferation promoting activity on human and hamster cells (CitationYagi et al., 1997), and likewise fibroblast proliferation was noted when treated with Carrisyn isolated from the gel extract of this plant (CitationReynolds & Dweck, 1999). Alopesin, from the same plant gel, has also been reported to stimulate proliferation of cultured human SK-HEP1 cells, a hepatoma like cell line (CitationDavis et al., 1994).
Within Asia, plants from the Zingiberaceae family have been valued and used since ancient times as not only spices and food preservatives but also important medicines. Within this diverse family, wild turmeric or jujin, Curcuma aromatica Salisb. (Zingiberales: Zingiberaceae), is a wild plant found in the warm forests of the eastern Himalayas in India (CitationRavindran et al., 2007). Along with turmeric (C. longa) and Javanese turmeric (C. xanthorrhiza), it has been widely used, especially the aromatic and carminative rhizomes, in medicine for such ailments as diabetes, atherosclerosis, indigestion, jaundice and hepatitis as well as bacterial infections (CitationKuhn & Winston, 2001). Previously it has been reported that certain components of C. aromatica, such as xanthorrhizol, 7-methanoazulene and curcumene, have anti-mosquito activity (CitationChoochote et al., 2005; CitationCao et al., 2006), additionally several major antitumor ingredients are found in extracts, including elemicin, curcumol, and curdione. Furthermore, oil extract of C. aromatica has been shown to exert various medical activities such as promoting blood circulation to remove blood stasis and treating cancer (CitationWu et al., 2000). Although other substances in C. aromatica, such as curdione, neocurdione, curcumol, tetramethylpyrazine and 1,2-hexadecanediol have been found (CitationHuang et al., 2000), they currently remain without any reported biological activities. In addition, at present there are no reports about the extraction and biological activities of the polysaccharides of C. aromatica. Consequently, we report on characterization of the polysaccharides from C. aromatica rhizomes and their biological activity on the in vitro cell proliferation of primary human gingival fibroblast cells.
Materials and methods
Plant material
Fresh rhizomes of C. aromatica were purchased from the Chatujak Market, Bangkok, Thailand, in March 2007. Thus, the cultivar, geographical region of cultivation, cultivation conditions and season of growth, which may all influence to some degree the level and type of bioactive compounds, remains unknown. Nevertheless, although these factors are known to affect some secondary plant metabolites, including within C. aromatica (CitationKojima et al., 1998), most polysaccharides are more generally expressed and this study can act as the initial reference for further cultivar regional or cultivation specific clarifications. To this end a voucher specimen (BK60395) is deposited at the Bangkok Herbarium (BK) of the Plant Variety Protection Division, Department of Agriculture, Thailand, by Kongkanda Chayamarit (purchaser). The fresh rhizomes were sliced and then dried at 50°C overnight in the oven before grinding into a power.
Extraction and precipitation of crude polysaccharide
The crude polysaccharide was extracted by hot water and precipitated by 4 volumes of 95% ethanol (CitationBendjeddou, et al., 2003 CitationZhao, et al., 2006). The dried and ground C. aromatica rhizome powder was suspended in distilled water at 1 g per 20 mL and stirred in a heated water bath at 95°C for 3 h to solvate the hot water soluble components. The suspension-solution mixture was then allowed to cool and was stored overnight at 4°C prior to harvesting of the solute by centrifugation for 30 min at 13000 g and 20°C. The supernatant was harvested, concentrated in a rotary evaporator under reduced pressure at 50°C and clarified by filtration. The filtrate was then precipitated with four volumes of 95% (v/v) ethanol and the suspension kept overnight at 4°C. The precipitate was then harvested by centrifugation for 45 min, 13000 g at 20°C, washed with ethanol, air dried and then dissolved in distilled water 600 mL. This solution was then deproteinized three times with a mixed solvent extraction (1:5 (v/v) ratio of butanol: chloroform) to precipitate the protein. The supernatant was dialyzed against distilled water for 72 h, precipitated by four volumes of 95% (v/v) ethanol overnight and then harvested by centrifugation as detailed above. The crude polysaccharide precipitate was then washed by 20 mL of 95% (v/v) ethanol and freeze dried for 24-36 h, yielding the dried crude polysaccharide extract.
Further purification of the crude polysaccharide extract
The crude polysaccharide preparation was dissolved in distilled water at 1.5 mg/mL and then 10 mL of solution injected into a DEAE-cellulose anion exchange column (1.6 cm × 15 cm; Amersham Pharmacia Biotech; Piscataway New jersey USA). The column was initially eluted with 500 mL distilled water at 1 mL/min collecting 10 mL fractions, and then followed by 500 mL of 0.5 M NaCl as a gradient elution at the same flow rate and fraction collection volume. The major polysaccharide fractions, determined by phenol sulfuric acid method (CitationNielsen, 2003), were then purified by Superdex G-200 gel filtration column chromatography (1.6 × 60 cm; Amersham Pharmacia Biotech), eluted with 1000 mL of distilled water at a flow rate of 0.5 mL/ min and collecting 10 mL fractions. The polysaccharide fractions were determined by the phenol sulfuric acid method before being pooled and dried.
Determination of the molecular weight by gel permeable chromatography (GPC)
The molecular weight of each purified polysaccharide sample was determined on a Waters model 600E composed of a Waters Ultra-hydroseal linear 1 column and a refractive index detector. The purified polysaccharide solution 2 mg/mL (20 μL) was injected with 0.05 M sodium bicarbonate buffer (pH 11) as a mobile phase at 0.6 mL/min. Pullulan was used as a standard (MW of 5,900−788,000 Da).
Monosaccharide composition
Polysaccharide samples (10 mg) were hydrolyzed with 2 M trifluoroacetic acid (TFA) at 100°C for 5 h, neutralized by 1M NaOH and dialyzed by distilled water then injected to high performance liquid chromatography (HPLC) (Alltech; Nicholasville Kentucky USA) using a LiChrospher NH2 column (Merck; Darmstadt Germany), and eluted with a 17:3 (v/v) ratio mixture of acetonitrile: distilled water at 25°C with a flow rate of 1.5 mL/min and detected by an evaporative light scattering detector (ELSD). Glucose, galactose, fructose, ribose and xylose were used as monosaccharide standards.
FT-IR spectroscopy
The polysaccharides were ground with KBr powder and then pressed into pellets for determination of FT-IR using a Model 1600 FT-IR machine (PerkinElmer, Waltham Massachusetts USA).
Polysaccharide mediated in vitro effects on primary human gingival fibroblast cell proliferation
Human gingival fibroblast cells were obtained from the Faculty of Dental Medicine of Chulalongkorn University, Thailand, and grown in complete DMEM (Dulbecco’s Modified Eagle’s Medium with 10% (v/v) FBS, antibiotics and 2 mM l-glutamine). The cells were incubated at 37°C in a humidified atmosphere of 5% (v/v) CO2: 95% (v/v) air. The media (5 mL/big plate) was changed every two days. For the subcultivation of cells, adhered cells at % confluency were separated from culture plates with trypsin solution (500 μL/big plate) for 45 sec (CitationThunyakitpisal et al., 2004).
Evaluation of cell proliferation by the MTT cell assay
The effect of these two polysaccharides upon primary human gingival fibroblast cell proliferation was determined by using a modification of the MTT assay (CitationThunyakitpisal et al., 2004). The human gingival fibroblast cells were plated at 7 × 104 cells/well in 500 μL of complete DMEM in 24-well plastic tissue culture plates and incubated as above for 24 h. After 24 h the complete DMEM was removed from each well and replaced with 500 μL serum-free DMEM per well and then incubated as before for 24 h, with a change of serum-free DMEM every 3 h, and this procedure was repeated twice. The test polysaccharides were then mixed with the serum-free DMEM at various concentrations (100, 10, 1, 0.1 and 0 μg/mL), and added, in 500 μg/mL per well, after removing the serum-free DMEM and incubated for 24 h as above. The cell proliferation was determined by the MTT method as follows. The MTT solution (7 mg/ mL) was added (400 μL) to each well and incubated for 8-10 min as above, then removed from the wells and the cells washed with fresh DMEM and drained. The MTT-formazan crystals formed in the cells were then dissolved by adding 800 μL of dimethylsulfoxide (DMSO) to each of the wells and the solution aspirated and transferred to cuvettes for measurement by spectrophotometer, using test wavelength at 570 nm and using DMEM with 10% FBS as a positive control.
Statistical analysis
All the data were evaluated using the SPSS (Statistical Package for the Social Science) program, to determine the mean ± standard deviation, and to analyze for statistically significant differences between means using one-way ANOVA and Turkey’s method, with significant differences accepted at the p < 0.05 level.
Results and discussion
Purification
Crude polysaccharide (6.16 g) from Curcuma aromatica was obtained from 600 g wet weight rhizome by drying, grinding and extracting from the dry powder by hot water and subsequent precipitation by ethanol, as detailed in the methods section, and is summarized in . The subsequent extraction and purification steps of crude polysaccharides, detailed in the Methods section, are schematically summarized in . DEAE-cellulose anion exchange column chromatography separated the crude polysaccharide preparation into two peaks; an unbound fraction, called P1, and a bound fraction, called P2, respectively (). Since P1 was eluted by water it is likely to be a neutral polysaccharide, whereas since P2 was eluted by 0.5 M NaCl it is likely to be an acidic polysaccharide.
Table 1. The polysaccharide yield from 600 g (wet weight) fresh Curcuma aromatica rhizomes.
Figure 1. Schematic diagram summarizing the main stages in the extraction and purification of polysaccharides from fresh C. aromatica rhizomes.
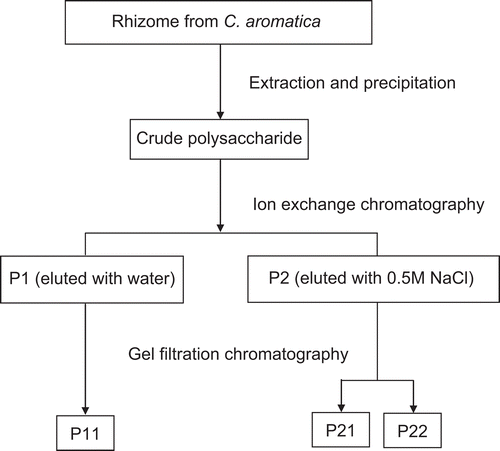
Figure 2. (A) DEAE-cellulose ion exchange column chromatogram showing the separation profile of crude polysaccharide, giving P1 and P2 from distilled water and 0.5M NaCl elution, respectively. (B) Superdex 200 gel filtration column chromatogram for fraction P1 (B) and fraction P2 (C), from the distilled water elute.
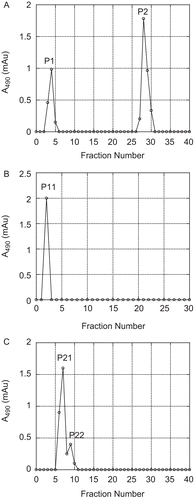
These two fractions, P1 and P2, were then subjected to further purification using Superdex G-200 gel filtration column chromatography, resulting in only a single peak from fraction P1, called P11, at fraction numbers 2-3 (), in a moderate yield of some 4% of the total crude polysaccharide fraction. For fraction P2, two peaks were separated, called P21 and P22, at fraction numbers 5-8 and 8-10, respectively (). These two peaks slightly overlapped each other and were at lower yields, being 1.8% and 0.01% of the total crude polysaccharide preparation respectively. Due to the low yield attained for P22, this fraction was not analyzed any further in this study.
Characterization of purified products
The fractions P11 and P22 were each analyzed by FT-IR and HPLC for structure elucidation, and by gel permeation chromatography (GPC) for molecular weight determination.
The results from FT-IR spectroscopy supported that P11 and P21 are both polysaccharides from their functional groups (supplementary data Figure S1). The intensity of the bands between 3600-3200 cm−1 are ascribed to O-H stretching in the constituent sugar residues of polysaccharides, whilst the absorbance at ∼2929 cm−1 represent C-H stretching in the sugar ring. Both P11 and P21 have residual water bands around 1640-1644 cm−1, whilst bands at 1200-1460 cm−1 represent the C-H and O-H deformation vibrations, and those at 730-900 cm−1 the C-H deformation vibrations. The band at 1000-1160 cm−1 that appears only in P11 is a C-O bond, whilst the bands from 1000-1125 cm−1 are forms of xylan and those at 1035-1111 cm−1 indicate the C-O-C and O-H residues in a pyran structure.
HPLC-based analysis of P11 and P21, following hydrolysis to monosaccharides, showed a single peak of hydrolyzed product for both P11 () and P21 () at a retention time of 4.75 which is the same retention time as that of the xylose standard (). Given that xylose is a heterocyclic ring consisting of five carbon atoms and one oxygen atom, the combined results from FT-IR and HPLC analyses suggest that P11 and P21 are both polyxyloses. That P21 is an acidic polysaccharide would suggest additional modifications. The molecular weights (MW) of P11 and P21 derived from GPC gave a weight-average of 469,171 and 157,665 Da, respectively (supplementary data Figure S2). Moreover, the MW of P11 and P22 derived from gel filtration chromatography also concurred with the results of GPC in that that P11 has higher molecular weight than P21.
Cell proliferation activity of P11 and P21
P21 was tested for effects upon the in vitro proliferation of cultured primary human gingival fibroblast cells (passage 5) using a modified MTT assay as outlined in the methods section. At a concentration of 10 and 100 μg/mL the P21 fraction slightly (but not statistically) and markedly (and statistically p < 0.05) inhibited the cell growth (92.2% ± 3.5% relative to the control (). Thus, P21 can decrease or obstruct mitochondrial function leading to insufficient energy levels in the human gingival fibroblast cells for their repair and regeneration (CitationChang et al., 2001; CitationIssa et al., 2008). Certainly the polysaccharide arecoline is reported to be cytotoxic to human gingival fibroblast cells by inhibiting the mitochondrial function (CitationChang et al., 2001), where 50-200 μg/mL arecoline can inhibits 16-56% of mitochondrial activity. Given that here P21 at 100 μg/mL appeared to inhibit around 90% of mitochondrial activity (), then P21 would appear to be a significantly more efficient inhibitor than arecoline. Furthermore, although heavy metal ions, such as Cd2+, Hg2+, MeHg, Au3+ and Cu2+, have been reported to inhibit mitochondrial activity, at 14, 20.2, 12.1, 40, 46 and 92 mg/mL, respectively, they attained only ∼50% inhibition of mitochondrial activity (CitationZhao et al., 2006), which is significantly less than that achieved here by P21 at 100 μg/mL.
Figure 4. The effect of polysaccharides (P11 and P21) on the in vitro cell proliferation of human primary gingival fibroblasts (passage 5), as determined by the MTT assay. Cells were treated with P11 or P21 at the indicated final concentrations (there is no 1,000 μg/mL for P21) for 24 h. Data are shown as the mean ± 1 SD, and are derived from eight replicates. *Denotes a significant difference from the control group (p < 0.05). The cell culture in DMEM with 10% FBS as a positive control can increase the cell around 195% compared to control.
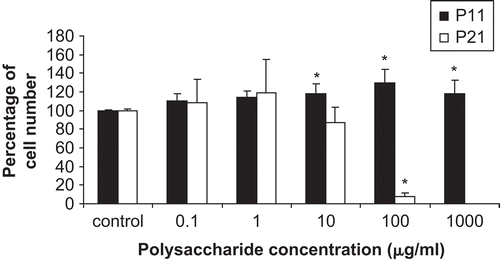
On the other hand, P11 at concentrations of 10, 100 and 1,000 μg/mL showed a slight (18.2 ± 10.6%, 30.1 ± 13.7% and 18.5 ± 14.4%, respectively, increase relative to the control) but statistically p < 0.05 ability to increase the number of human gingival fibroblast cells. The commercial acetylated mannan (glucomannan), acemannan, extracted from Aloe barbadensis Mill. (Liliaceae), was reported to significantly induce the cell proliferation (p < 0.05) of human gingival fibroblast cells at a concentration of 400, 800, and 1,000 μg/mL (CitationJettanacheawchankit et al., 2008), but this again is using at least a four-fold higher concentration of acemannan than that required by P11.
Declaration of interest
This work was supported by Chulalongkorn University graduate scholarship.
References
- Bendjeddou D, Lalaoui K, Satta D (2003): Immuno-stimulating activity of the hot water-soluble polysaccharide extracts of Anacyclus pyrethrum, Alpinia galanga and Citrullus colocynthis. J Ethnopharmacol 88: 155–160.
- Cao W, Li XQ, Liu L, Wang M, Fan HT, Li C, Lv Z, Wang X, Mei Q (2006): Structural analysis of water-soluble glucans from the root of Angelica sinensis (Oliv.) Diels. Carbohydrate Res 341: 1870–1877.
- Chang YC, Hu CC, Lii CK, Tai KW, Yang SH, Chou MY (2001): Cytotoxicity and arecoline mechanisms in human gingival fibroblasts in vitro. Clin Oral Investig 5: 51–56.
- Choochote W, Chaiyasit D, Kanjanapothi D, Rattanachanpichai E, Jitpakdi A, Tuetun B, Pitasawat B (2005): Chemical composition and anti-mosquito potential of rhizome extract and volatile oil derived from Curcuma aromatica against Aedes aegypti (Diptera: Culicidae). Vector Ecol 30: 302–309.
- Davis RH, Donato JJ, Hartman GM, Haas RC (1994): Anti-inflammatory and wound healing activity of a growth substance in Aloe vera. J Am Pediatr Med Assoc 84: 77–81.
- Huang KX, Tao ZM, Zhang AJ, Peng SL, Ding LS (2000): Studies on chemical constituents of Curcuma aromatica Salisb. Zhongguo Zhong Yao Za Zhi 25: 163–165.
- Issa Y, Brunton P, Waters CM, Watts DC (2008): Cytotoxicity of metal ions to human oligodendroglial cells and human gingival fibroblasts assessed by mitochondrial dehydrogenase activity. Dent Mater 24: 281–287.
- Jettanacheawchankit N, Sasithanasate S, Sangvanich P, Banlunara W, Thunyakitpisal P (2008): Acemannan stimulates GFs proliferation, KGF-1, VEGF expressions and wound healing. Paper given at the Annual Meeting of International Association for Dental Research, Toronto, Canada. July 5th 2008.
- Kojima H, Yanai T, Toyota A (1998): Essential oil constituents from Japanese and Indian Curcuma aromatica rhizomes. Planta Med 64: 380–381.
- Kuhn MA, Winston D (2001): Herbal Therapy and Supplements: A scientific and Traditional Approach. New York, Lippincott.
- Lindhorst TH (2003): Essentials of Carbohydrate Chemistry and Biochemistry. Weinheim, Germany, Wiley.
- Luo DH (2008): Identification of structure and antioxidant activity of a fraction of polysaccharide purified from Dioscorea nipponica Makino. Carbohydrate Polymer 71: 544–549.
- Nielsen S (2003): Food Analysis Laboratory Manual. Netherlands, Kluwer Academic/Plenum.
- Ravindran PN, Nirmal Babu K, Sivaraman K (2007): Turmeric: The Genus Curcuma. Boca Raton, CRC Press.
- Reynolds T, Dweck AC (1999): Aloe vera leaf gel: A review update. J Ethno-Pharmacol 68: 3–37.
- Thunyakitpisal, P, Tavedhikul, K, Heamkulchon, K (2004): Aloe Vera gel extract stimulates the proliferation of primary cultured human periodontal ligament cells and pulpal fibroblasts. J Pharmacol Sci 94: 403–409.
- Wang ZJ, Luo DH (2007): Antioxidant activities of different fractions of polysaccharide purified from Gynostemma pentaphyllum Makino. Carbohydrate Polymer 68: 54–58.
- Wu WY, Xu Q, Shi LC, Zhang WB (2000): Inhibitory effects of Curcuma aromatica oil on proliferation of hepatoma in mice. World J Gastroenterol 6: 216–219.
- Yagi A, Egusa T, Arase M, Tanabe M, Tsuji H (1997): Isolation and characterization of the glycoprotein fraction with a proliferation-promoting activity on human and hamster cells in vitro from aloe vera gel. Planta Med 63: 18–21.
- Yang X, Guo D, Zhang J, Wu M (2007a): Characterization and antitumor activity of pollen polysaccharide. Int Immunopharmacol 7: 401–408.
- Yang X, Guo D, Zhang J, Wu M (2007b): Characterization and antitumor activity of pollen polysaccharide. Int Immunopharmacol 7: 427–434.
- Zha XQ, Luo JP, Luo SZ, Jiang ST (2007): Structure identification of a new immunostimulating polysaccharide from the stems of Dendrobium huoshanense. Carbohydrate Polymer 69: 86–93.
- Zhao C, Li M, Luo YF, Wu WK (2006): Isolation and structural characterization of an immuno stimulating polysaccharide from fuzi, Aconitum carmichaeli. Carbohydrate Res 341: 485–491.