Abstract
Aristolochia elegans Mast. (Aristolochiaceae) has been used to treat scorpion envenoming in Mexican traditional medicine. In vitro studies of the pharmacological activity of raw extracts from A. elegans roots have shown activity against scorpion bite. The aim of the present study was to determine for the first time the antagonistic effect of hexane and methanol extracts of the aerial parts and roots from micropropagated A. elegans plants in a model of isolated guinea-pig ileum contracted by scorpion bite. Results showed that the methanol extracts of aerial organs (74%) and roots (65%) of micropropagated plants have a similar antitoxin activity against scorpion poisoning to hexane extracts of wild plants (65%). These results suggest that using methanol extracts from the micropropagated plant material instead of wild plant root extracts from A. elegans is an alternative for treatment against scorpion bite symptoms, and will contribute to the conservation of this medicinal species.
Introduction
In Mexico, 134 scorpion species of the Buthidae family have been described, 30 of which belong to the genus Centruroides. Eight of them are known to represent a lethal risk: Centruroides elegans Thorell, C. infamatus infamatus Pocock, C. limpidus limpidus Karsch, C. limpidus tecomanus Hoffmann, C. noxius Hoffmann, C. pallidiceps Pocock, C. sculpturatus Ewing, and C. suffusus suffusus Pocock (CitationDehesa-Dávila et al., 1995).
The endemic region of the scorpion in Mexico covers 30.3% of the country’s territory, and includes the north and central states of Sonora, Sinaloa, Durango, Nayarit, Colima, Jalisco, Michoacán, Guanajuato, Puebla, Morelos, Guerrero, and Oaxaca. This region is inhabited by 36.8% of the country’s population. The scorpion bite is a public health problem, since 250,000 cases are reported annually, with a mortality rate of up to 1000 deaths per year (CitationBush & Charles, 2003; CitationSecretaría de Salud, 2007). Although institutional treatment for the scorpion puncture exists and has improved with the use of hyperimmune equine serum (CitationNOM-033-SSA-2002, 2003), which diminishes allergic reactions, its high price and poor availability in rural areas hamper its application, and most cases continue to be treated by medicinal plants.
In Mexican traditional medicine, several plant species are used for scorpion envenoming, including Aristolochia elegans Mast. (Aristolochiaceae), Bouvardia ternifolia Schltdl. (Rubiaceae), Vitex mollis HBK. (Verbenaceae), and Ipomea arborescens (Humb. & Bonpl. ex Willd.) G. Don. (Convolvulaceae) (CitationJiménez-Ferrer et al., 2005). The traditional use of A. elegans suggests that ingestion of its root infusion is useful for the treatment of poisoning by scorpion puncture, as well as asthma and rheumatic pain; it also increases uterine activity (MartÃnez, 1959; CitationLozoya et al., 1987; CitationMonroy & Castillo, 2007).
Pharmacological assays have demonstrated that the extract of A. elegans shows cytotoxic (CitationMurillo et al., 2001), antibiotic (CitationGadhi et al., 2001), insecticidal (CitationBroussalis et al., 1999), and anticholinergic properties (CitationRastrelli et al., 1997). These activities are related to aristolochic acid, a phospholipase-specific inhibitor, which is able to diminish the release of pain and inflammation mediators (CitationHutt & Houghton, 1998). Our group has studied the pharmacological relaxing activity of wild A. elegans in an isolated guinea-pig ileum model contracted by scorpion venom, and it was found that the methanol extract of the roots was not as active as the hexane extract (CitationJiménez-Ferrer et al., 2005).
Until now, all pharmacological and phytochemical research has been done using material obtained from wild plants, but it is becoming increasingly difficult to find A. elegans in its natural habitat (CitationMonroy & Castillo, 2007). Since the root is the commonly used part, the whole plant is inevitably destroyed (CitationJiménez-Ferrer et al., 2005). A useful alternative would be to establish the conditions of propagation and large-scale culture to allow the production of plants focused on medicinal use. These plants would present homogeneous characteristics in their morphology as well as pharmacological activity (CitationRates, 2001). A possible strategy to produce enough biomass could be micropropagation. This process is a biotechnologically useful tool that helps to achieve healthy and vigorous plants in shorter times than required in natural conditions. Additionally, this technique can be helpful in preserving the natural flora of an area (CitationChawla, 2002; CitationRout et al., 2000). Recently, CitationOsuna et al. (2007) reported the micropropagation of A. elegans, although the anti-scorpion venom activity of the extracts of micropropagated plants was not evaluated.
Thus, the aim of the present study was to determine the effect of hexane and methanol extracts of micropropagated A. elegans plants on the characteristic symptoms following scorpion bite, and in particular, to determine their antagonistic activity on guinea pig ileum contraction.
Materials and methods
Biological materials
Seeds of A. elegans were obtained from the Centro de Investigación Biomédica del Sur (CIBIS-IMSS), Xochitepec, Morelos (México). The plants were previously identified by personnel of the herbarium, IMSSH, and registered with the code HPMIMSS13595. These seeds were disinfected and germinated in in vitro conditions, as described by CitationOsuna et al. (2007). Axillary buds were used as a source of explants, which were placed on 100% Murashige and Skoog (MS) medium (CitationMurashige & Skoog, 1962) supplemented with benzylaminopurine (BAP) 10 µM during 8 weeks for shoot induction. Thereafter they were transferred to MS medium supplemented with indole butyric acid (IBA) 1.5 µM, for root induction, and finally the plantlets were acclimatized to greenhouse conditions.
Extract preparation
The leaves and stems (aerial parts) as well as the roots of in vitro propagated A. elegans grown for 20 weeks in greenhouse conditions were dried separately in dark conditions at room temperature, and the dried material was ground. Hexane (HE) and methanol (ME) extracts were prepared by successive percolation of dried material, in 0.8:1 (v/dw) proportions of solvent and biological material, respectively. Finally, the extracts were concentrated at 40°C under vacuum in a rotary evaporator (Büchi, Switzerland), and freeze-dried. Samples were stored in amber bottles to protect them from the light and kept at between 0 and 4°C until use.
Obtaining of scorpion venom
The scorpion venom was obtained from 100 exemplars of C. limpidus limpidus Karsch collected at Puente de Ixtla, Morelos, Mexico. The venom was collected in a glass dish using a telson-directed electrical impulse. The venom was dissolved in 10 mL deionized water and clarified by centrifugation (14,000 rpm, 10 min at 4°C). Aliquots of 500 µL from the supernatant were placed in 1 mL vials and lyophilized.
In vitro antagonism test
Animals
Hartley male guinea-pigs (400–600 g weight) were kept in the CIBIS-IMSS bioterium, at 22 ± 2°C with 12 h light–dark cycles and unlimited access to food and water. The guinea-pigs were sacrificed by cervical dislocation and the distal ileum was obtained by laparotomy. After washing with Tyrode’s solution, a 2 cm section of the ileum was dissected and placed in an incubation chamber. The tissue was fixed at the bottom of the chamber with silk thread, while the other side was tied with the same thread via a force transducer, which was connected to an analogous digital data acquisition processor (Maclab 8; USA). The tissue was maintained with 1 g force of tension at 37°C in Tyrode’s solution and bubbled with a mixture of 5% CO2 and 95% O2 Citation(Jiménez-Ferrer et al., 2005). All studies were performed in accordance with Official Mexican Norm NOM-062-ZOO-1999 (technical specifications for production, care, and use of laboratory animals).
In order to quantify the percentage of ileum contraction, the preparation was exposed to 10−4 M carbamylcholine solution (analogous to acetylcholine). The contraction generated was considered as 100% and used as a reference. In the first of two concentration–response curves, a wide range of venom concentrations (1, 10, 100, and 1000 µg/mL) were evaluated. In the second experiment, the venom concentration was limited to the range showing the highest effect (10, 20, 30, 40, 60, and 100 µg/mL).
Relaxation test
For the relaxation test, the scorpion venom was dissolved in isotonic saline solution (ISS) at a concentration of 40 µg/mL and added to the chamber. After 30 s, HE and ME (extracts dissolved in Tyrode’s solution) were added separately at concentrations of 50, 100, 150, 200, 250, and 300 µg (dw/mL) and incubated for 2 min. The effect of the extracts was determined by calculating the percentage of decrease of the contraction provoked by the venom.
Statistical analysis
The assays were analyzed by bifactorial analysis of variance (ANOVA) and post hoc Tukey test. The significance level for all tests was 5% (α = 0.05). Sigma-Stat (V. 3.0) software was used for all statistical analysis.
Results
The ME of the roots (Ro) presented a viscous appearance and amber color, while aerial organ extracts (Ao) were green. The HE of both Ro and Ao showed a pasty consistency, with a yellow color and a very strong smell.
shows the extraction yield of the different organs of micropropagated A. elegans plants. The best yields were obtained for ME (18.06% for Ao and 9.44% for Ro), while HE showed lower yields (Ao 0.83% and Ro 0.37%, respectively). These results contrast with the yields obtained from A. elegans wild plants by CitationJiménez-Ferrer et al. (2005), who reported 0.57% for HEAo. The difference might be explained by seasonal variability, or the phenophase of the plant, both of which influence biosynthesis and secondary metabolite accumulation. Our results confirm those of CitationVila et al. (1997), who observed some differences in composition of the essential oil of A. elegans, mainly in sesquiterpenes, depending on the season as well as the plant phenology.
Table 1. Hexane and methanol extraction yield from aerial organs and roots of micropropagated A. elegans.
In order to know the optimal concentration of scorpion venom that induces maximal ileum contraction, we evaluated several concentrations, as shown in . It was observed that the highest contraction was obtained at a concentration range between 10 and 100 µg/mL (). In an approximation (), we found that a concentration of 40 µg/mL was sufficient to induce the maximal response. Both curves show typical sigmoid tendencies, and depicts the three characteristic phases described by CitationWilliamson et al. (1998). In the first and shortest stationary phase, the concentrations reach the stimulus threshold. The second linear phase is characterized by an increase of the response in correlation with the concentration. Finally, in the third, saturation phase, increases in concentration do not affect the contraction response.
Figure 1. Effect of venom concentration on ileum contraction. (a) Exploratory curve, (b) determinant curve; n = 6.
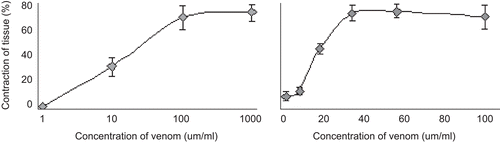
depicts two superimposed recordings in real time of isolated guinea-pig ileum force and movement. The grey line shows the effect of the venom (sustained tissue contraction), while the black line reveals the relaxation effect on the contracted ileum after the addition of MEAo of A. elegans (200 µg/mL). Ileum contraction induced by venom was reversed by the addition of the extracts from micropropagated A. elegans plants. As shown in , the contraction was strongly antagonized, reaching an effect of 74.38% with MEAo.
Figure 2. Recording showing force and movement of isolated guinea-pig ileum and the effect produced by the addition of 40 µg/mL of C. limpidus limpidus venom (grey line) and 40 µg/mL venom followed by addition of 200 µg/mL MEAo of A. elegans (black line).
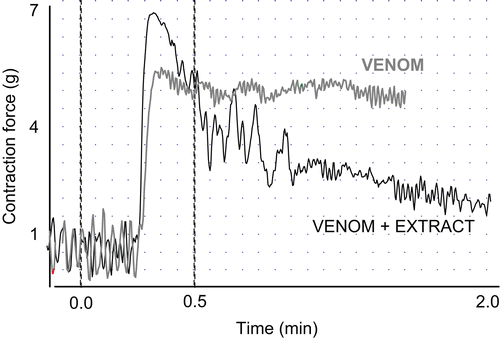
The relaxation effect of the extracts is represented by concentration–response curves. compares the effects of the different extracts of micropropagated A. elegans on the relaxation of the ileum and data for the HERo of wild plants. The effect of all tested extracts was similar up to a concentration of 150 µg/mL, after which some differences were observed. HERo and HEAo presented similar tendencies in that relaxation responses were not affected by increments in their concentration. These results suggest that the system reached saturation with 150 µg/mL of extract concentration (42 and 45%, respectively). On the other hand, MERo and MEAo reached their maximal response, 65 and 74%, respectively, at a concentration of 300 µg/mL. Generally, we observed a concentration-dependence effect, which means that each increase in the extract concentration had an effect on the relaxation response.
Figure 3. Inhibition produced by the addition of different concentrations of several A. elegans extracts. Each data point shows the mean and standard deviation of six observations. The dark line represents the results reported by CitationJiménez-Ferrer et al. (2005) with HE of wild plants, which is the mean and standard deviation of five repetitions.
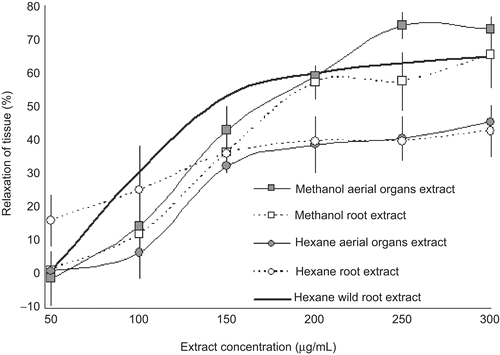
shows the three typical phases of sigmoid curves. The stationary phase was short, and it was evident that in this time the stimulus threshold was reached. The second phase was characterized by a linear tendency, where the response was directly proportional to the extract concentration and the maximal value could be attributed to saturation of the stimulus receptors. Finally, in the third phase the response was stable and independent of the extract concentration.
The most relevant result is that the ME of both roots and aerial organs of the micropropagated plants of A. elegans had the same effect on biological activity as HERo extracts reported for wild plants (CitationJiménez-Ferrer et al., 2005). This suggests that using extracts from the aerial parts of micropropagated plant material instead of the roots of wild plants of A. elegans will contribute to conservation of the species and present an alternative treatment against scorpion bite symptoms.
Discussion
The bite of dangerous toxic scorpions is considered an acute intoxication in humans, eventually fatal, due to the neurotoxic effect of the poison’s active principles (CitationDehesa-Dávila et al., 1995). The study of scorpionism has not achieved great importance, despite the recent isolation of scorpion toxins that act selectively on certain ion channels and that have served to pharmacologically characterize the structures of the excitable cells (CitationCatterall et al., 2007). More information is needed to explain the physiopathological effects of the poison and to develop rational treatment schemes (CitationSecretaría de Salud, 2007).
In Mexico, there is an estimated 70% under-registration of scorpion bites; thus, considering the 300,000 annual cases reported nationally, it can be estimated that there are up to 1,000,000 accidents of this type (CitationNOM-033-SSA-2002, 2003; CitationZúñiga et al., 2008). It is also known that 70–80% of the population have used traditional herbal medicine as their first therapeutic option (CitationGupta, 1997). Thus, if in the Mexican state of Morelos 32,499 accidents were reported for 2007 (CitationSecretaría de Salud, 2007), it can be estimated that, due to under-registration, up to 75,000 accidents actually occurred in the rural area of the state, bearing in mind that these are the same patients who can employ guaco (A. elegans) as their main herbal remedy (CitationBaytelman, 1993).
Therefore, annual consumption of the fresh plant (root) as a treatment could be between 2 and 2.5 tons. This high demand for A. elegans roots together with the loss of natural spaces where the plant grows means it is of great importance to establish in vitro cultures of the species (CitationDirzo & García, 1992; CitationTorres, 2004). This would secure a constant supply of excellent quality control-associated plant material, in addition to allowing pharmacological, phytochemical, and secondary metabolic studies in vitro to continue without depleting the resource.
In this work, we corroborated, to our knowledge for the first time, the antivenom activity of micropropagated A. elegans aerial parts and roots. Comparing our results with those obtained previously with wild plants (CitationJiménez-Ferrer et al., 2005), we can see that the micropropagated plants maintained the ability to antagonize the toxic effects of scorpion venom. It was also observed that the methanol extract from the micropropagated plant’s aerial parts (leaves and stems) presents greater activity than the wild plant’s root-derived hexane extract and the micropropagated plant’s root-derived methanol extract (10 and 15% more, respectively). This could be attributed to the maturity of the plants in question, since the micropropagated plants were 4 months old and the wild plant material was more than 1 year old.
The genus Aristolochia is characterized by a marker-related carcinogenic compound denominated aristolochic acid (aa), which tends to accumulate mainly in the roots of several species of the genus (CitationWu et al., 2000, Citation2002). It has been demonstrated that chronic intake of this compound (aa) can be nephro- and hepatotoxic (CitationMei et al., 2006; CitationZhang et al., 2007).
In our laboratory, we evaluated the effect of aa against scorpion venom (data not presented) and observed that the 50% lethal dose (LD50) of the venom was not modified, nor was the guinea-pig isolated ileum contraction counteracted. With respect to the plant extract’s activated fraction, we discarded the presence of aa; nonetheless, we detected neolignan-type compounds with antitoxic activity that exhibited chromatographic characteristics distinct from aa (data not presented). The methanol extracts (MEAo, MERo) were more effective but less potent than the hexane extracts (HEAo, HERo). The Emax values were 90.7 and 71.42% for MEAo and MERo, respectively, and the IC50 values were 92.27 and 73.92 μg/mL for each extract. On the other hand, the hexane extracts showed Emax values of 43.5 and 45.5% and IC50 values of 44.9 and 46.7 μg/mL for aerial parts and root extracts, respectively. Thus, based on our results, we can conclude that instead of the roots, the aerial parts may be used for studies of biological activity and mechanism of action, in addition to contributing to the study of secondary metabolism in vitro.
The results we have obtained are a contribution to the study of traditional medicine usage, corroborating scorpion antivenom activity in an A. elegans micropropagated-plant aerial-part in vitro model.
Declaration of interest
The authors wish to thank the General Coordination of Postgraduate Studies and Research – IPN (Coordinacion General de Posgrado e Investigación – IPN) for financial resources given to the research project “Production of secondary metabolites of pharmacological interest (anti-scorpion venom) in Aristolochia elegans (Mast.) plantlets cultured in vitro”, CGPI20030343. Three of the authors (E.V.Z., A.J.A., and K.B.T.) are grateful for the support of COFAA-IPN. The authors wish to thank Lucia Brzoska (UB) for reviewing the manuscript.
References
- Baytelman B (1993): Acerca de Plantas y de Curanderos. Etnobotánica y Antropología Médica en el Estado de Morelos. México, INAH, pp. 452.
- Broussalis AM, Ferraro GE, Martino VS, Pinzón R, Coussio JD, Calle AJ (1999): Argentine plants as potential source of insecticidal compounds. J Ethnopharmacol 67: 219–223.
- Bush SP, Charles JG (2003): Scorpion envenomation. Available at: http://www.emedicine.com/Emerg/topic524.htm. Accessed 24 July 2008.
- Chawla HS (2002): Introduction to Plant Biotechnology. Enfield, NH, Science Publishers, Inc., pp. 18.
- Catterall WA, Cestèle S, Yarov-Yarovoy V, Yu FH, Konoki K, Scheuer T (2007): Voltage-gated ion channels and gating modifier toxins. Toxicon 49: 124–141.
- Dehesa-Dávila M, Alagón A, Possani LD (1995): Clinical toxicology of scorpion sting. In:Jürg MJW, ed., Clinical Toxicology of Animal Venoms and Poisons. Boca Raton, FL, CRC Press, pp. 221–238.
- Dirzo R, García M (1992): Rates of deforestation in Los Tuxtlas, a neotropical area in southeast México. Conserv Biol 6: 84–90.
- Gadhi CA, Benharref A, Jana M, Brasile AM, Contet-Audonneau N, Fortier B (2001): Antidermathophytic properties of extracts from the leaves of Aristolochia paucinervis Pomel. Phytother Res 15: 79–81.
- Gupta M (1997): La industria de fitofármacos en Latinoamérica. In:Lozoya X, Gómez E, eds., I Fitofármacos. México, IMSS-Farmasa Schwabe, pp. 339–337.
- Hutt MJ, Houghton PJ (1998): A survey from the literature of plants used to treat scorpion stings. J Ethnopharmacol 60: 97–110.
- Jiménez-Ferrer JE, Pérez-Terán YY, Roman-Ramos R, Tortoriello J (2005): Antitoxin activity of plants used in Mexican traditional medicine against scorpion poisoning. Phytomedicine 12: 116–22.
- Lozoya X, Aguilar A, Camacho AR (1987): Encuesta sobre el uso actual de la medicinal tradicional mexicana. Rev Med IMSS 25: 283–291.
- Martínez M (1959): Las Plantas Medicinales de México. Mexico, Botas, pp. 268–276.
- Mei N, Arlt VM, Phillips DH, Heflich RH, Chen T (2006): DNA adduct formation and mutation induction by aristolochic acid in rat kidney and liver. Mutat Res 602: 83–91.
- Monroy OC, Castillo EP (2007): Plantas Medicinales Utilizadas en el Estado de Morelos. México, Centro de Investigación en Biotecnología (CEIB), Centro de Investigaciones Biológicas, Universidad Autónoma del Estado de Morelos (UAEM), pp. 47.
- Murillo AJ, Encarnación DR, Franzblau SG (2001): Antimicrobial and cytotoxic activity of some medicinal plants from Baja California Sur (Mexico). Pharm Biol 39: 445–449.
- Murashige T, Skoog F (1962): A revised medium for rapid growth and bioassays with tobacco cultures. Physiol Plant 15: 473–497.
- NOM-033-SSA-2002 (2003): Norma Oficial Mexicana Secretaría de Salud para la vigilancia, prevención y control de la intoxicación por picadura de alacrán. Available at: http://www.salud.gob.mx/unidades/cdi/nom/033ssa202.html. Accessed 4 June 2009.
- Osuna TL, Mora IA, Ventura ZE, Jiménez FJE, Bazaldúa MC, Jiménez AA (2007): Micropropagation of Aristolochia elegans (Mast.). J Crop Sci Biotechnol 10: 105–110.
- Rastrelli L, Capasso A, Piza C, De Tommasi N, Sorentino L (1997): New protopine and benzyl tetrahydropeotoberberine alkaloids from Aristolochia constricta and their activity on isolated guinea pig ileum. J Nat Prod 60: 1065–1069.
- Rates SMK (2001): Plants as source of drugs. Toxicon 39: 603–613.
- Rout GR, Samantaray S, Das P (2000): In vitro manipulation and propagation of medicinal plants. Biotechnol Adv 18: 91–120.
- Secretaría de Salud (2007): Sistema nacional de vigilancia epidemiológica. Epidemiología 24: 1–3.
- Torres RJM (2004): Estudio de tendencias y perspectivas del sector forestal en América Latina al año 2020. Informe Nacional. Available at: http://www.fao.org/docrep/006/j2215s/j2215s00.htm. Accessed 4 June 2009.
- Vila R, Mundina M, Muschietti L, Priestap HA, Bandoni AL, Adzet T, Canigueral S (1997): Volatile constituents of leaves, roots and stems from Aristolochia elegans. Phytochemistry 46: 1127–1129.
- Williamson E, Okpak TD, Evans JR (1998): Selection, Preparation and Pharmacological Evaluation of Plant Material, Vol. 1. Chichester, Wiley & Sons, pp. 25–27.
- Wu TS, Tsai YL, Damu AG, Kuo PC, Wu PL (2002): Constituents from the root and stem of Aristolochia elegans. J Nat Prod 65: 1522–1525.
- Wu TS, Leu YL, Chan YY (2000): Constituents from the stem and root of Aristolochia kaempferi. Biol Pharm Bull 23: 1216–1219.
- Zhang L, Mu X, Fu J, Zhou Z (2007): In vitro cytotoxicity assay with selected chemicals using human cells to predict target-organ toxicity of liver and kidney. Toxicol In Vitro 21: 734–740.
- Zúñiga CIR, Lozano JC, Esparza AM, Zaragoza JCA, Herbas RIM, Aguilar AN, Gómez ACM (2008): Alacranismo: Enfoque clínico y epidemiológico para el personal de salud. Vacunación Hoy 15: 84–91.