Abstract
Context: Lobophora variegata J.V. Lamouroux (Dictyotaceae) is a brown marine alga widely encountered in the Brazilian sea coast that presents high content of fucans. Anti-inflammatory effects of fucans are reported mostly in models in vitro, but little is known about its effects in vivo.
Objective: To investigate vascular and cellular effects of a sulfated polysaccharide from the brown marine algae L. variegata (SP-Lv) in acute inflammatory models.
Materials and methods: SP-Lv was isolated by DEAE-cellulose and analyzed by agarose gel electrophoresis and evaluated for its inhibitory effect on paw edema, vascular permeability, leukocyte migration and peritoneal nitrite content induced by zymosan in Wistar rats. Anticoagulant activities and possible systemic toxicity were also evaluated.
Results: SP-Lv inhibited the paw edema (120 min: 1.42 ± 0.11 vs. 0.95 ± 0.05 mL), plasma exudation (21.53 ± 0.62 vs. 11.96 ± 0.68 μg/g), nitrite content (4.42 ± 0.33 vs. 2.86 ± 0.003 μM) and leukocyte migration (5.15 ± 1.21 vs. 1.99 ± 0.16 cells/103 mL) induced by zymosan. SP-Lv and l-NAME reduced the paw edema (60–120 min) elicited by l-arginine. However, at 180 min SP-Lv effect was more accentuated and sustained until 240 min, while that of l-NAME was abolished. Similarly to indomethacin, SP-Lv inhibited the entire edema time-course induced by phospholipase A2, except for the time of 60 min.
Discussion and conclusion: The anti-edematogenic effect of SP-Lv seems to occur via inhibition of nitric oxide synthase and cyclooxygenase activities. These results suggest a potential applicability of polysaccharides from alga origin in acute inflammatory conditions.
Keywords::
Introduction
Sulfated polysaccharides are complex macromolecules of broad action due to their chemical structure, rich in polyanions, which allow connection to a large number of proteins, either in matrix or plasma cells (CitationArfors & Ley, 1993). These macromolecules are widely distributed in nature, being found in microorganisms, animals (CitationCássaro & Dietrich, 1977) and also in marine algae that present a high content of sulfated polysaccharides of important biological activities and large potential applicability.
The anticoagulant (CitationMourão & Pereira, 1999) and antithrombotic (CitationFarias et al., 2000) properties are the most studied, but sulfated polysaccharides are known to modulate a large number of other activities, such as antiviral (CitationDamonte et al., 1994), antitumor (CitationLins et al., 2009), antiatherosclerotic (Engelberg, Citation1991), antioxidant (CitationZhang et al., 2003) and antinociceptive (CitationAssreuy et al., 2008).
In respect to inflammation, it was demonstrated that a sulfated polysaccharide from the red marine algae Champia feldmannii Díaz-Piferrer (Champiaceae) presents edematogenic activity, paralleled with increase in vascular permeability and leukocyte migration into rat peritoneal cavities (CitationAssreuy et al., 2008). On the other hand, polymers of sulfated polysaccharides extracted from brown algae (fucans) showed potent inhibition on the human complement system in vitro (CitationBlondin et al., 1994; CitationTissot et al., 2003). In this line, fucans of high molecular weight were able to inhibit the venular leukocyte rolling and endothelial lymphocyte adhesion induced by zymosan (CitationAruffo et al., 1991; CitationWeston & Parish, 1991). Further, it was shown that a fucoidan extracted from the brown marine algae Fucus vesiculosus Linn. (Fucaceae), commonly known as bladderwrack, presents antithrombotic, anti-complement, and anti-proliferative effects in smooth muscle cells (CitationYang et al., 2006). Later, a heterofucan from the brown seaweed of L. variegata J.V. Lamouroux (Dictyotaceae) subcutaneously injected in rats was shown to inhibit leukocyte migration induced by thioglycolate and ear swelling induced by croton oil in sensitized animals (CitationMedeiros et al., 2008).
Since there are few studies of sulfated fucans in models of inflammation in vivo, we have investigated vascular and cellular effects of a sulfated polysaccharide from the brown marine algae L. variegata in acute inflammatory models.
Methods
Animals
Male Wistar rats (150–250 g body weight) were maintained in a controlled 12/12 h light/dark cycle, temperature of 25°C with free access to food and water. Experimental protocols were approved by the Institutional Animal Care and Use Committee of the State University of Ceará – UECE (no. 0559924-4), Fortaleza, Brazil, in accordance with the National Institutes of Health (NIH, 1985) for the Care and Use of Laboratory Animals. No. 85–23, Revised 1985.
Algae
Specimens of the brown marine alga Lobophora variegata Lamouroux (Phylum ochrophyta, class: Phaeophyceae, order: Dictyotales, family: Dictyotaceae) are widely encountered on the southeast and northeast Brazilian coast. The species was collected in September 2008 from the Pacheco beach of Caucaia, Ceará, Brazil and classified by Wladimir R.L. Farias, Department of Fishery Engineering, Federal University of Ceará, Brazil, and a voucher specimen (no. 1809) is deposited in the Marine Sciences Institute Herbarium, Federal University of Ceará, Brazil.
Drugs and reagents
Dextran sulfate, carrageenan, Evans blue, zymosan, cetylpyridinium chloride, sodium acetate, l-arginine, papain, N-cetyl-N,N,N-trimethylammonium bromide, acetic acid, 1-9-di-metylene blue (DMB), ethylenediaminetetraacetic acid (EDTA), indomethacin, l-N-nitro-arginine methyl ester (l-NAME), toluidine blue, agarose gel (Sigma, St. Louis, MO and Steinheim, Germany); absolute ethanol, sodium chloride, calcium chloride (Merck, Darmstadt, Germany); DEAE-cellulose (Amersham Bioscience, Upsala, Sweden); heparin (Cristália Produtos Químicos e Farmacêuticos, São Paulo, Brazil); activated cephalin (Celite Biolab, Mérieux, France); cysteine (Alfagene, Carcavelos, Portugal). The enzymatic kits used for evaluation of the systemic toxicity were from LABTEST (Diagnostic Tests-Brazil).
Extraction and isolation of sulfated polysaccharides
Crude extract
Algae dry tissue (2 g) were suspended in 100 mL of 0.1 M sodium acetate buffer (pH 5.0), containing 6.8 mL papain solution (30 mg/mL), 5 mM EDTA and 5 mM cystein, and incubated at 60°C for 24 h. The incubation mixture was filtered, centrifuged (2560 × g; 20 min; 4°C) and the supernatant saved. Residue was washed with 55.2 mL distilled water, filtered and the two supernatants combined. Sulfated polysaccharides were precipitated with 6.4 mL of 10% cetylpyridinium chloride (CPC) and left at room temperature for 24 h. The mixture was centrifuged (2560 × g; 20 min; 5°C) and pellet washed with 200 mL of 0.05% CPC, dissolved in 70 mL of 2 M NaCl:ethanol (100:15 v/v) and precipitated with 122 mL absolute ethanol. After 24 h at 4°C, the precipitate was collected by centrifugation (2560 × g; 20 min; 5°C), washed twice with 200 mL of 80% ethanol, and once with the same volume of absolute ethanol. The final precipitate containing total sulfated polysaccharides was dried at 60°C for 24 h (CitationFarias et al., 2000). The algal pellet was submitted to four additional extractions yielding approximately 28% (dry weight).
Ion exchange chromatography and agarose gel electrophoresis
Total sulfated polysaccharides of L. variegata (5 mg), obtained from algae tissue papain digestion, were dissolved in 5 mL of 50 mM sodium acetate (pH 5.0). From this, 1 mL was applied to a DEAE-cellulose analytical column (90 × 20 mm) equilibrated in the same buffer. Elution was performed using 20 mL/fraction of the equilibrium buffer in NaCl stepwise (0.5, 0.7, 0.9, 1.2, 1.4, 1.6 M). The flow rate was 60 mL/h and each fraction of 1 mL was collected for dosage of sulfated polysaccharide and total carbohydrate assessed by metachromatic assay (CitationFarndale et al., 1986) and Dubois method (CitationDubois et al., 1956), respectively (Ultrospec 1000, Spectrophotometer-Pharmacia, Amersham Biosciences).
Total and polysaccharide fractions (15 μg) were applied to a 0.5% agarose gel and run for 60 min at 110 V in 0.05 M 1,3-diaminopropane:acetate (pH 9.0). Sulfated polysaccharides in the gel were fixed with 0.1% N-cetyl-N,N,N-trimethylammonium bromide. After 12 h, the gel was dried and stained with 0.1% toluidine blue in acetic acid:ethanol:water (0.1:5:5 v/v) (CitationFarias et al., 2000). The purified polysaccharide from L. variegata will be referred to as SP-Lv.
Clotting assays
Clotting times were assessed by the activated partial thromboplastin time (aPTT) according to the manufacturer’s specifications using human plasma obtained from healthy donors at the Hematology Center of the Federal University of Ceara, Brazil. The anticoagulant action was recorded in a coagulometer (Drake, Quick-timer, Presidente Prudente, São Paulo, Brazil) and expressed as international units/mg (IU/mg) using a parallel curve based on heparin standard (100 IU/mg).
Paw-edema model
Paw volume was measured immediately before subcutaneous (s.c.) injection of inflammatory stimuli (0 min) into the hind paw of rats and at selected time intervals thereafter (0.5, 1, 2, 3, 4 and 5 h) by hydroplethysmometry (PanLab, LE 7500) (CitationMota et al., 2006). Results were expressed as increase or reduction in paw volume (mL) calculated by subtracting the basal volume measured at 0 min. Area under the curve (AUC) was expressed in arbitrary units (CitationLanducci et al., 1995).
Anti-inflammatory activities of SP-Lv
SP-Lv (0.01–1 mg/kg; 0.1 mL/100 g of body weight) was injected intravenously (i.v.) 30 min before edema induction by s.c. administration of dextran sulfate (300 μg), carrageenan (2 mg) or zymosan (1 mg). Negative controls received sterile saline (NaCl 0.9%) s.c. or i.v.
Inflammatory mediators search
Rats were treated i.v. with SP-Lv (1 mg/kg) or saline, 30 min before s.c. injection of bovine phospholipase A2 (30 mg) or l-arginine (15 µg) into animal paws. Indomethacin (5 mg/kg, s.c.) or l-N-nitro-arginine methyl ester (l-NAME, 30 mg/kg, i.v.) were injected 30 and 60 min, respectively, before inflammatory stimuli as inhibitory controls (CitationCirino et al., 1989).
Vascular permeability
Zymosan (1 mg, s.c.) was administered into animal paws and compared to control animals injected with saline. Rats received Evans blue (25 mg/kg; i.v.) 1 h before sacrifice. Paws were sectioned at the ankle, weighed and incubated with 4 mL formamide at 37°C for 72 h. The optical density (at 600 nm) of the extracted dye was spectrophotometrically (Ultrospec 1100) estimated and expressed as μg of Evans blue/g of tissue based on a standard Evans blue curve (CitationWilhelm, 1962). SP-Lv treatment (1 mg/kg, i.v.) was performed 30 min before zymosan.
Peritonitis model
Animals were intraperitoneally (i.p.) injected with zymosan (1 mg/mL) or saline and sacrificed 4 h later. Cells were harvested by washing peritoneal cavities with 10 mL saline (5 IU heparin) for total and differential leukocyte (neutrophils, eosinophils, mast cells and mononuclear) counts (cytocentrifuge Wescor, cytopro 7620) (CitationSouza & Ferreira, 1985). SP-Lv was injected i.v. (1 mg/kg), 30 min before zymosan. Results were expressed as cells per mL of peritoneal wash.
Measurement of nitric oxide concentration in peritoneal exudates
Nitric oxide (NO) production was evaluated according to the Griess method (CitationGreen et al., 1982). Nitrite (NO2–) concentration of the peritoneal exudates from animals 4 h after i.p. injection of zymosan (1 mg/mL) or sterile saline (control) was measured using a spectrophotometer (At 540 nm). Results were expressed as µM of nitrite.
Systemic effects
Rats were injected daily with a single dose of saline or SP-Lv (1 mg/kg; i.v.), for 7 consecutive days. Animals were weighed at the beginning and at the end of treatment. Peripheral blood was collected for leukogram (CitationSouza & Ferreira, 1985) and biochemical analysis of urea, creatinine, alanine amine transferase (ALT), plasma albumin and globulin (determined by enzymatic and colorimetric tests – LABTEST). After sacrifice, heart, spleen, kidney and liver were removed, weighed (wet weight expressed as g/100 g of body mass) and macroscopically analyzed (CitationMota et al., 2006).
Statistical analysis
Data are presented as mean ± SEM of n animals per group and statistical differences were analyzed using analysis of variance (ANOVA) followed by Duncan or Student’s t-test. Significance was set at p <0.05.
Results
Purification of sulfated polysaccharides from L. variegata
Anion exchange chromatography on DEAE cellulose column separated total sulfated polysaccharides of L. variegata into six fractions (F1-F6), that were eluted with 0.5, 0.7, 0.9, 1.2, 1.4 and 1.6 M NaCl, respectively (). Fractions F1 and F2 presented high metachromatic activity, but only F1 showed high hexose content. Minor amounts of sulfated polysaccharides (F5 and F6) were still eluted with 1.4 and 1.6 M NaCl, respectively. The chromatogram profiles of the five-times extracted total polysaccharides were similar (data not shown), but only F1 (SP-Lv), eluted at 0.5 M, showed a single band, although polydisperse, revealed by agarose gel electrophoresis ().
Figure 1. Purification of sulfated polysaccharides from L. variegata. Fractions collected from DEAE-cellulose were assayed for metachromasia (□), Dubois reaction (•), and NaCl concentration (arrows). Fractions were pooled, dialyzed against distilled water, and lyophilized (A). Total (TSP) and fractions (F1-SP-Lv, F2, F3 and F4) of sulfated polysaccharides were applied to a 0.5% agarose gel electrophoresis and stained with 0.1% toluidine blue in acetic acid/ethanol/water (0.1:5:5, v/v) (B).
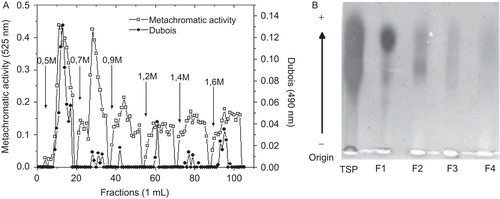
Despite high content of carbohydrate and metachromatic activity, SP-Lv did not present anticoagulant activity showing clotting time similar to the normal human plasma (45.6 s).
SP-Lv inhibits paw edema, vascular permeability and leukocyte migration
Dextran and carrageenan elicited typical edematogenic effects, starting at 30 min (1.22 ± 0.06 mL vs. saline: 0.40 ± 0.07 mL) and 60 min (0.92 ± 0.07 mL vs. saline: 0.07 ± 0.04 mL), respectively. SP-Lv showed anti-edematogenic effect, which varied in accordance with the inflammatory stimuli. The i.v. pre-treatment with SP-Lv inhibited about 19% (0.98 ± 0.06 mL) the first 30 min of the edema induced by dextran () and about 47% (0.48 ± 0.04 mL) the second h of that induced by carrageenan ().
Figure 2. SP-Lv inhibits the initial phase of the paw edema induced by dextran and the second hour of that induced by carrageenan. A) Dextran (300 μg) or B) carrageenan (2 mg) were administered s.c. as inflammatory stimuli into animal paws. Negative controls received saline. Edema was measured by hydroplethysmometry before (0 min) and 30, 60, 120, 180, 240 and 300 min after stimuli and expressed as the variation in paw volume (mL). SP-Lv (1 mg/kg; i.v.) was injected 30 min before inflammatory agents. Mean ± SEM (n = 6). ANOVA followed by Duncan’s test. *p < 0.05 × saline; #p < 0.05 × dextran or carrageenan.
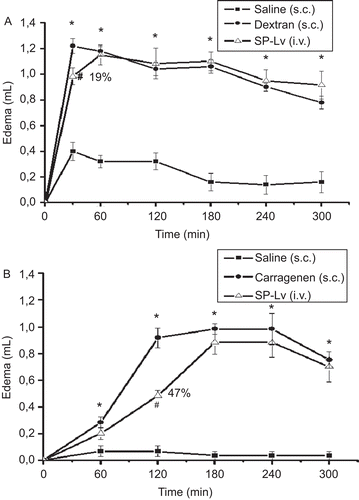
Zymosan evoked a typical paw edema, maximal at 60 min (1.59 ± 0.06 mL vs. saline: 0.27 ± 0.07 mL). SP-Lv, at the highest dose (1 mg/kg; i.v.), inhibited almost the entire edema time-course starting at 1 h and presenting maximal inhibition by 32% at the second h (zymosan: 1.42 ± 0.11 mL vs. SP-Lv: 0.95 ± 0.05 mL), an effect sustained up to 300 min (). At the second h, SP-Lv inhibited the increase in vascular permeability induced by zymosan by 44% (zymosan: 21.53 ± 0.62 μg/g × SP-Lv: 11.96 ± 0.68 μg/g) ().
Figure 3. SP-Lv inhibits the time course of paw edema and the increased vascular permeability induced by zymosan. Zymosan (1 mg) or saline were administered s.c. into animal paws. SP-Lv (1 mg/kg, i.v.) was injected 30 min before zymosan. Edema was measured by hydroplethysmometry before (0 min) and 30, 60, 120, 180, 240 and 300 min after stimuli and expressed as the variation in paw volume (mL) (A). Animals received Evans blue (25 mg/kg; i.v.) and were sacrificed 1 h later. Optical Density (At 600 nm) was estimated and results presented as μg of Evans blue/g of tissue (B). Mean ± SEM (n = 7). ANOVA followed by Duncan test. *p < 0.05 × saline; #p < 0.05 × zymosan.
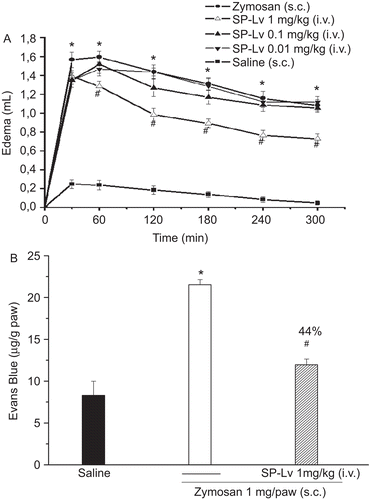
SP-Lv inhibited the leukocyte migration induced by zymosan (5.15 ± 1.21 cells/103 mL vs. saline: 1.28 ± 0.14 cells/103 mL) about 61% (1.99 ± 0.16 cells/103 mL). The decrease in leukocyte number was mainly due to the inhibitory action upon neutrophils, about 75% (0.46 ± 0.12 cells/103 mL) compared to zymosan (4.2 ± 1.08 cells/103 mL) (). Additionally, the nitrite (NO2) concentration of the peritoneal exudates obtained from SP-Lv treated animals was decreased by 35% (2.86 ± 0.003 μM) compared to the non-treated, stimulated with zymosan (4.42 ± 0.33 μM) ().
Figure 4. SP-Lv inhibits the leukocyte migration and elevated NO2 levels induced by zymosan. Animals received zymosan (1 mg/mL; i.p.) or saline and were sacrificed 4 h later. Peritoneal fluid was harvested with 10 mL saline (5 IU heparin) and collected for total (A) and differential (B) leukocyte counts (cells/mL) and NO2 levels (μM) were determined (C). SP-Lv (1 mg/kg; i.v.) was injected 30 min before zymosan. Mean ± SEM (n = 6). ANOVA followed by Duncan test. *p < 0.05 × saline; #p < 0.05 × zymosan.
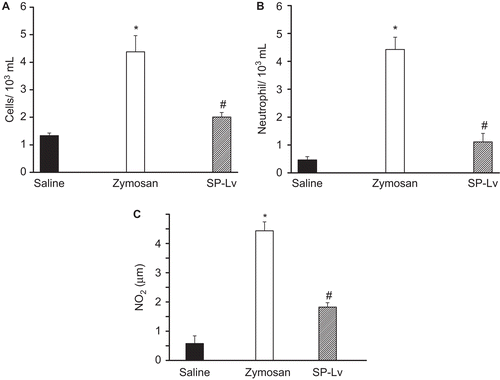
Involvement of inflammatory mediators in the SP-Lv anti-edematogenic effect
l-Arginine used as a substrate for NO generation, evoked intense paw edema (expressed as AUC) (l-arginine: 241.5 ± 21.24 vs. saline: 25 ± 11.68). This edematogenic effect was inhibited by SP-Lv at the following times: 60 min (0.52 ± 0.02) by 43%, 120 min (0.35 ± 0.05) by 59%, 180 min (0.18 ± 0.04) by 79% and 240 min (0.22 ± 0.02 mL) by 65% compared to respective controls (0.9 ± 0.01, 0.86 ± 0.04, 0.88 ± 0.04, 0.62 ± 0.08 mL). Pre-treatment of animals with l-NAME similarly reduced the paw edema at 60 min (0.5 ± 0.01 mL) and 120 min (0.38 ± 0.08 mL) in 44 and 56%, respectively. However, at 180 min, the SP-Lv inhibitory effect was more accentuated and sustained until 240 min, while that of l-NAME was abolished. The edema induced by l-arginine was inhibited about 31% by l-NAME and 57% by SP-Lv ().
Figure 5. SP-Lv inhibits the paw edema induced by l-arginine and phospholipase A2. Animals were treated i.v. with SP-Lv (1 mg/kg) or saline, 30 min before s.c. injection of phospholipase A2 (30 mg/paw) or l-arginine (15 µg/paw). Indomethacin (5 mg/kg; s.c.) or l-NAME (30 mg/kg; i.v.) were injected 30 min and 60 min, respectively, before inflammatory stimuli. Edema was measured by hydroplethysmometry before 0 min and 30, 60, 120, 180 and 240 min after stimuli and expressed as the variation in paw volumes (mL). Mean ± SEM (n = 6). ANOVA followed by Duncan test. *p < 0.05 × saline; #p < 0.05 × l-arginine or phospholipase A2.
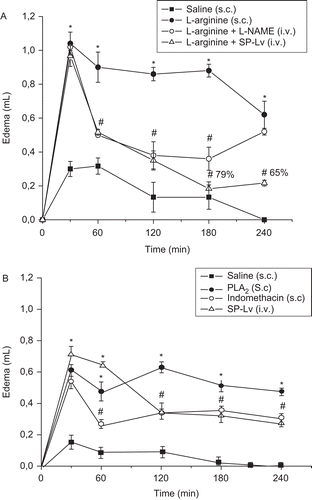
Phospholipase A2, an enzyme that stimulates prostanoids release, promoted intense edema (expressed as AUC) (124 ± 3.75) compared to saline (21 ± 4.88). SP-Lv significantly inhibited the edema time-course (19%) at the following times: 120 min (0.35 ± 0.04) by 45%, 180 min (0.33 ± 0.03) by 35%, 240 min (0.28 ± 0.03) by 41% and 300 min (0.28 ± 0.03 mL) by 24% compared to respective controls (0.63 ± 0.04, 0.52 ± 0.03, 0.48 ± 0.02, 0.37 ± 0.02 mL). The inhibitory effect of indomethacin was similar to SP-Lv along the entire edema time-course, except for 60 min ().
SP-Lv does not cause systemic toxicity
An important observation of this study was that SP-Lv (1 mg/kg, i.v.) did not cause systemic toxicity in vivo. The seven-day SP-Lv treatment did not affect animals’ body mass or the wet weight of important immune system organs such as heart, spleen, kidney and liver compared to controls injected with sterile saline (). All organs evaluated appeared normal (showing no edema) and stomach mucosa intact, without visible lesions. Osmotic equilibrium, seen by the dosage of plasma albumin and globulin, was also unchanged. The number of blood circulating leukocytes was unaltered, suggesting that SP-Lv treatment does not promote leukocyte agglutination or secondary effects in lymphoid tissues, therefore preserving the number of defense cells. Also, dosage of urea, creatinine and the kinetics of ALT, used as markers of renal and hepatic function did not differ from controls ().
Table 1. Treatment of rats with SP-Lv did not alter animal body mass, organ wet weight or leukogram.
Table 2. Treatment of rats with the SP-Lv does not alter plasma biochemical profile.
Discussion
The different charge density is a common feature among algae polysaccharides (CitationAssreuy et al., 2008). Accentuated variations in the yield of sulfated polysaccharide purifications are described to be mostly dependent on the algae species and extraction process (CitationChotigeat et al., 2004). In this study the employment of sequential extractions of sulfated polysaccharides optimized the purification process.
SP-Lv did not present anticoagulant activity showing clotting times similar to normal human plasma. In general, discrete variations in content and position of the sulfate group result in significant changes in the anticoagulant activity (CitationFarias et al., 2000) and for galactans, 2,3-dissulfated α-galactose units are required for its anticoagulant effect (CitationPereira et al., 2005).
SP-Lv showed anti-edematogenic effect varying in accordance with the inflammatory stimuli. Dextran is an inflammatory substance that elicits osmotic edema with important participation of histamine, serotonin, and bradykinin (CitationLo et al., 1982). However, carrageenan induces a biphasic and multimediated edema with intense leukocyte infiltrate (CitationDiRosa et al., 1971). SP-Lv inhibited both types of edema, more effectively the non-osmotic. The i.v. pre-treatment with SP-Lv inhibited about 19% the first 30 min of the edema induced by dextran and about 47% that induced by carrageenan.
SP-Lv, at the highest dose, inhibited almost the entire edema time-course starting at 1 h and presenting maximal inhibition by 32% at the second h, an effect sustained up to 300 min. At the second h, SP-Lv inhibited the increase in vascular permeability induced by zymosan about 44%. Zymosan produced intense inflammatory response with activation of the complement system and generation of chemotactic factors (CitationSnyderman et al., 1970; CitationFernandez et al., 1978). The SP-Lv anti-edematogenic effect is in line with the in vitro studies showing inhibitory effects of sulfated fucans upon the complement system (CitationBlondin et al., 1994; CitationTissot et al., 2003). The inhibitory action of SP-Lv upon plasma exudation was higher than its anti-edematogenic effect. It possibly justifies the inhibition observed in the initial phase of the osmotic edema induced by dextran. Besides, the inhibitory effect upon the paw swelling induced by zymosan and carrageenan, suggests an action of SP-Lv not only at vascular level but also in cellular events of the inflammatory process.
In fact, SP-Lv inhibited the leukocyte migration induced by zymosan about 61%, mainly due to the inhibitory action upon neutrophils, which was about 75% compared to zymosan. The major inhibitory effect of SP-Lv on neutrophils, cells found in abundance in the first 24 h of the inflammatory process, characterize a phase acute response. Accordingly, fucoidan and heterofucan have been shown to reduce leukocyte migration by different approaches (CitationKlintman et al., 2002; CitationMedeiros et al., 2008). Besides, the inhibitory effect of SP-Lv on the nitrite dosage from the peritoneal fluid of animals stimulated with zymosan reinforces the involvement of nitric oxide in the polysaccharide anti-inflammatory effect.
It is known that edema formation is a synergic response involving various inflammatory mediators which results in increased vascular permeability and/or blood flow (CitationWilliams & Peck, 1977). The paw edema elicited by zymosan shows a biphasic release of NO (early stage) and prostaglandins (late phase) (CitationDamas & Prunesco, 1990). It is also described that the paw edema elicited by zymosan stimulates iNOS messenger RNA expression in a period of 150 min to 24 h after injection (CitationGühring et al., 2001). Increasing evidence argues in favor of a considerable “cross talk” between the NO and prostaglandins biosynthetic pathways (CitationWeinberg, 2000). Therefore, phospholipase A2 initiates the cyclooxygenase and lipoxygenase cascades, leading to prostaglandins, thromboxanes and leukotrienes formation (CitationKini & Evans, 1989). The present finding demonstrated that SP-Lv inhibited the edema induced by phospholipase A2, except for the time of 60 min, following the same inhibitory pattern of indomethacin, a non-selective blocker of cyclooxygenase. SP-Lv also inhibited the edema induced by l-arginine similarly to l-NAME, a non-selective inhibitor of nitric oxide synthase, at 60 and 120 min, although the SP-Lv inhibitory action had been higher after this period. Based on the evidence that prostaglandin and nitric oxide participate in the paw edema induced by zymosan (CitationDamas & Prunesco, 1990), and in our present demonstration about the involvement of these mediators at the times in which SP-Lv was inhibitory against both stimuli (zymosan and carrageenan), it is possible to postulate that SP-Lv, beyond the interference with NO synthase, may be blocking the cyclooxygenase pathway.
The SP-Lv anti-inflammatory effect associated with the high tolerance shown by the animals during treatment suggest a potential applicability of sulfated polysaccharides from alga origin in acute inflammatory conditions.
Conclusions
The sulfated polysaccharide from the brown marine alga Lobophora variegata presented anti-inflammatory effect inhibiting edema, increasing vascular permeability and neutrophil migration and was well tolerated by animals. The anti-edematogenic effect seemed to occur via inhibition of nitric oxide sinthase and cyclooxygenase activities.
Acknowledgments
The authors thank the Conselho Nacional de Desenvolvimento Científico e Tecnológico-CNPq (Conselho Nacional de Desenvolvimento Científico e Tecnológico), Fundação Cearense de Amparo a Pesquisa-FUNCAP, Coordenação de Aperfeiçoamento de Pessoal de Nível Superior-CAPES (Coordenação de Aperfeiçoamento de Pessoal de Nível Superior) and Gabriela Fernandes Oliveira Marques Domingos for technical assistance.
Declaration of interest
R.C.L. Siqueira was the recipient of a fellowship from CAPES. A.M.S. Assreuy, N.M. Alencar, B.S. Cavada and A.H. Sampaio are senior investigators of CNPq/Brazil.
References
- Arfors KE, Ley K. (1993). Sulfated polysaccharides in inflammation. J Lab Clin Med, 121, 201–202.
- Aruffo A, Kolanus W, Walzs G, Fredman P, Seed B. (1991). CD26/P-selectin recognition of myeloid and tumor cell sulfatides. Cell, 67, 35–44.
- Assreuy AM, Gomes DM, Silva MS, Torres VM, Siqueira RC, Pires AF, Criddle DN, Alencar NM, Cavada BS, Sampaio AH, Farias WR. (2008). Biological effects of a sulfated-polysaccharide isolated from the marine red algae Champia feldmannii. Biol Pharm Bull, 31, 691–695.
- Blondin C, Fisher E, Boisson-Vidal C, Kazatchkine M, Jozefonvicz J. (1994). Inhibition of complement activation by natural sulfated polysaccharides (fucans) from brown seaweed. Mol Immunol, 31, 247–253.
- Cássaro CMF, Dietrich CP. (1977). Distribution of sulfated mucopolysaccharides in vertebrates. J Biol Chem, 252, 2254–2261.
- Cirino G, Peers SH, Flower RJ, Browning JL, Pepinsky RB. (1989). Human recombinant lipocortin 1 has acute local anti-inflammatory properties in the rat paw edema test. P Natl Acad Sci, 86, 3428–3432.
- Chotigeat W, Tongsupa S, Supamataya K, Phongdara A. (2004). Effect of fucoidan on disease resistance of black tiger shrimp. Aquac, 233, 23–30.
- Damas J, Prunesco P. (1990). Presence of immunoreactive platelet-activating factor in peritoneal exudate induced by zymosan in rats. Arch Int Pharmacodyn Ther, 322, 115–123.
- Damonte E, Neyts J, Pujol CA, Snoeck R, Andrei G, Ikeda S, Witvrouw M, Reymen D, Haines H, Matulewicz MC. (1994). Antiviral activity of a sulfated polysaccharide from red seaweed Nothogenia fastigata. Biochem Pharmacol, 47, 2187–2192.
- DiRosa M, Giroud JP, Willoughby DA. (1971). Studies of the mediators of the acute inflammatory response induced in rats in different sites by carrageenan and turpentine. J Path, 104, 15–29.
- Dubois M, Gilles KA, Hamilton JK, Rebers PA, Smith F. (1956). Colorimetric method for determination of sugars and related substances. Anal Chem, 28, 350–356.
- Engelberg H. 1991. Heparin, non-heparin glycosaminoglycans and heparinoids: An overview of their application in artherosclerosis. Semin Thromb Hemost, 17, 5–8.
- Farias WR, Valente AP, Pereira MS, Mourão PA. (2000). Structure and anticoagulant activity of sulfated galactans. J Biol Chem, 275, 29299–29307.
- Farndale RW, Buttle DJ, Barret AJ. (1986). Improved quantitation and discrimination of sulphated glycosaminoglycans by use of dimethylmethylene blue. Biochim Biophys Acta, 883, 173–177.
- Fernandez HN, Henson PM, Otani A, Hugli TE. (1978). Chemotactic response to human C3a and C5a anaphylatoxins. I. Evaluation of C3a and C5a leukotaxis in vitro and under stimulated in vivo conditions. J Immunol, 120, 109–115.
- Green LC, Wagner DA, Glogowski J. (1982). Analysis of nitrate, nitrite, and [15N] nitrate in biological fluids. Anal Biochem, 126, 131–138.
- Gühring H, Tegeder I, Lötsch J, Pahl A, Werner U, Reeh PW, Rehse K, Brune K, Geisslinger G. (2001). Role of nitric oxide in zymosan induced paw inflammation and thermal hyperalgesia. Inflamm Res, 50, 83–88.
- Kini RM, Evans HJ. (1989). A model to explain the pharmacological effects of snake venom phospholipases A2. Toxicon, 27, 613–635.
- Klintman D, Schramm R, Menger MD, Thorlacius H. (2002). Leukocyte recruitment in hepatic injury: Selectin-mediated leukocyte rolling is a prerequisite for CD18-dependent firm adhesion. J. Hepatol, 36, 53–59.
- Landucci EC, Antunes E, Donato JL, Faro R, Hyslop S, Marangoni S, Oliveira B, Cirino G, de Nucci G. (1995). Effect of crotapotin and heparin on the rat paw oedema induced by different secretory phospholipases A2. J Pharmacol, 114, 578–583.
- Lins KO, Bezerra DP, Alves AP, Alencar NM, Lima MW, Torres VM, Farias WR, Pessoa C, de Moraes MO, Costa-Lotufo LV. (2009). Antitumor properties of a sulfated polysaccharide from the red seaweed Champia feldmannii (Diaz-Pifferer). J Appl Toxicol, 29, 20–26.
- Lo TN, Almeida AP, Beaven MA. (1982). Dextran and carrageenan evoke different inflammatory response in rat with respect to composition of infiltrates in effect of indomethacin. J Pharmacol Exp Ther, 221, 261–267.
- Medeiros VP, Queiroz KC, Cardoso ML, Monteiro GR, Oliveira FW, Chavante SF, Guimarães LA, Rocha HA, Leite EL. (2008). Sulfated galactofucan from Lobophora variegata: Anticoagulant and anti-inflammatory properties. Biochemistry (Mosc), 73, 1018–1024.
- Mota MR, Criddle DN, Alencar NM, Gomes RC, Meireles AV, Santi-Gadelha T, Gadelha CA, Oliveira CC, Benevides RG, Cavada BS, Assreuy AM. (2006). Modulation of acute inflammation by a chitin-binding lectin from Araucaria angustifolia seeds via mast cells. Naunyn Schmiedebergs Arch Pharmacol, 374, 1–10.
- Mourão PA, Pereira MS. (1999). Searching from alternatives to heparin sulfated fucans from marine invertebrates. Trends Cardiovasc Med, 9, 225–232.
- Pereira MG, Benevides NM, Melo MR, Valente AP, Melo FR, Mourão PA. (2005). Structure and anticoagulant activity of a sulfated galactan from the red alga, Gelidium crinale. Is there a specific structural requirement for the anticoagulant action? Carbohyd Res, 340, 2015–2023.
- Snyderman R, Phillips J, Mergenhagen SE. (1970). Polymorphonuclear leukocyte chemotactic activity in rabbit serum and guinea pig serum treated with immune complexes: Evidence for C5a as the major chemotactic factor. Infect Immun, 1, 521–525.
- Souza GE, Ferreira SH. (1985). Blockade by antimacrophage serum of the migration of PMN neutrophils into the inflamed peritoneal cavity. Agents Actions, 17, 97–113.
- Tissot B, Montdargent B, Chevolot L, Varenne A, Descroix S, Gareil P, Daniel R. (2003). Biological properties of sulfated fucans: The potent inhibiting activity of algal fucoidan against the human complement system. Biochim Biophys Acta, 1651, 5–16.
- Weinberg JB. (2000). Nitric oxide synthase 2 and cyclooxygenase 2 interactions in inflammation. Immunol Res, 22, 319–341.
- Weston SA, Parish CR. (1991). Modification of lymphocyte migration by mannans and phosphomannans: Different carbohydrate structures control entry of lymphocytes into spleen and lymphonodes. J Immunol, 146, 4180–4186.
- Wilhelm DL. (1962). The mediation of increased vascular permeability in inflammation. Pharmacol Rev, 14, 251–280.
- Williams TJ, Peck MJ (1977). Role of prostaglandin-mediated vasodilatation in inflammation. Nature, 270, 530–532.
- Yang JW, Yoon SY, Oh SY, Kim SK, Kang KW. (2006). Bifunction effects of fucoidan on the expression of inducible nitric oxide synthase. Biochem Biophys Res Commun, 346, 345–350.
- Zhang Q, Li N, Zhou G, Lu X, Xu Z, Li Z. (2003). In vivo antioxidant activity of polysaccharide fraction from Porphyra haitanesis (Rhodophyta) in aging mice. Pharmacol Res, 48, 151–155.