Abstract
Context: The flowers of Woodfordia fruticosa Kurz. (Lythraceae) are commonly used for the treatment of several ailments which includes rheumatism, leucorrhea, menorrhagia, asthma, liver disorder, and inflammatory conditions.
Objective: To evaluate the hepatoprotective property of Woodfordia fruticosa flowers against acetaminophen-induced hepatic injury in rats.
Material and methods: Acetaminophen (3 g/kg bw)-induced hepatotoxicity study was carried out by observing the effect of methanol extract of Woodfordia fruticosa flowers (400 and 600 mg/kg, bw) on some serum marker enzymes, albumin, blood urea nitrogen levels as well as liver total protein, nonenzymetic glutathione reduced content, and enzymatic antioxidant glutathione peroxidase, with histopathological evidence.
Results and discussion: Pretreatment of rats with methanol extract of Woodfordia fruticosa flowers effectively prevented the acetaminophen-induced hepatic damage as indicated by the serum marker enzymes aspartate aminotransferase, alanine aminotransferase, and alkaline phosphatase and other biochemical parameters (albumin and blood urea nitrogen). Parallel to these changes, the methanol extract of Woodfordia fruticosa flowers also prevented acetaminophen-induced oxidative stress in the rat liver by inhibiting depletion of liver total protein and restoring the levels of nonenzymatic antioxidant glutathione reduced. The biochemical changes were consistent with histopathological observations suggesting marked hepatoprotective effect of the methanol extract of Woodfordia fruticosa flowers.
Conclusion: The results suggested that methanol extract of Woodfordia fruticosa flowers possesses protective effect against acetaminophen-induced hepatotoxicity.
Introduction
Acetaminophen/paracetamol (APAP) has been widely used as a medicine for pain and fever relief (CitationSmilkstein et al., 1994). As APAP can be purchased easily from any pharmaceutical outlet and even from supermarkets, without prescriptions from clinicians, it is commonly considered as a ‘‘safe drug’’ when taken within the suggested therapeutic dose. However, APAP can be hepatotoxic when an overdose is administered. Clinically, APAP has been demonstrated to be nephrotoxic and hepatotoxic from animal experiments and in human beings (CitationVermeulen et al., 1992; CitationBonkovsky et al., 1994; CitationHau et al., 2009). The recommended maximum “safe” dose of APAP that can be ingested over 24 h is 4 g in adults and 60 mg/kg in children. While APAP is the classical example of a dose-dependent hepatotoxic drug, there is no definite threshold dose for hepatic injury. The amount of APAP ingested as a single dose required to produce injury is relatively variable. Single doses of APAP exceeding 7 to 10 g in adults or 150 mg/kg body weight in children are enough to cause significant hepatocellular necrosis (CitationHamlyn et al., 1978; CitationKnight et al., 2003).
The World Health Organization (WHO) has long recognized and drawn the attention of many countries to the ever increasing interest of the public in the use of medicinal plants and their products in the treatment of various ailments. Plants have wide acceptability by the people and serve as cheaper alternatives to orthodox medicine (CitationAkah & Nwabie, 1994; CitationAkuodor et al., 2010). Woodfordia fruticosa Kurz. (Lythraceae) is a shrub widely grown in India, East Africa, and South East Asia. In India, it is a much used medicinal plant in Ayurvedic and Unani systems of medicines (CitationDymock et al., 1995). In India (Gujarat), it is known as Dhavdi (CitationShome et al., 1981; CitationKhare, 2004). All parts of this plant possess valuable medicinal properties viz. anti-inflammatory (CitationAlam et al., 1990), antitumor (CitationYoshida et al., 1990), hepatoprotective (CitationChandan et al., 2008), immunomodulatory (CitationShah & Juvekar, 2010), antibacterial (CitationParekh & Chanda, 2007), and free radical scavenging activity (CitationKumaraswamy & Satish, 2008). Many marketed drugs are composed of flowers, fruits, leaves, and buds mixed with pedicels and thinner twigs of the plant (CitationNadkarni, 1954; CitationAhuja, 1965). The flowers are being used in the preparation of Ayurvedic fermented drugs called “Aristha’s” and “Asava’s” (CitationAtal et al., 1982; CitationDas et al., 2007). In the present study, we have evaluated the potential hepatoprotective effects of methanol extract of Woodfordia fruticosa flowers against APAP-induced hepatic damage in rats.
Material and methods
Chemicals
All chemicals were of analytical-reagent grade and obtained from the following sources: Silymarin from Micro Labs Ltd. (Solan, HP, India); APAP from GlaxoSmithKline Pharmaceuticals Ltd. (Mumbai, India); Petroleum ether, methanol, acetic acid, hydrochloric acid from Merck (Darmstadt, Germany); Biochemical kits from Span Diagnostics Ltd (Sachin, Surat, India); Dipotassium hydrogen phosphate, potassiun dihydrogen orthophosphate, sodium citrate, hydrogen peroxide (H2O2), trichloroacetic acid (TCA), 5,5-dithiobis (2-nitrobenzoic acid) (DTNB), sodium azide, glutathione reduced (GSH), Tris free base were purchased from Himedia (Mumbai, India).
Plant material
The fresh flowers of Woodfordia fruticosa were collected from Junagadh (Girnar region), Gujarat, India in the month of March 2009. The plant was compared with voucher specimen (voucher specimen No. PSN303) deposited by Dr. P.S. Nagar at Department of Biosciences, Saurashtra University, Rajkot, Gujarat, India.
Preparation of the extract
The flowers were washed under tap water, air-dried, homogenized to fine powder, and stored in airtight bottles. Dried powder (10 g) was first defatted with petroleum ether and then extracted with methanol by using Soxhlet apparatus (CitationLin et al., 1999). The solvent was evaporated to dryness by rotary evaporator and the dried crude extract was stored in an air tight bottle at 4°C. The percentage yield of methanol extract was 36%. For testing, the Woodfordia fruticosa methanol (WFM) extract was dissolved in sterile distilled water and diluted to the desired concentrations.
Animals
Wistar albino rats of either sex (180–220 g) were used for the study. The animals were obtained from Xcelris Labs Ltd., Ahmedabad. All the rats were kept in standard plastic rat cages with stainless steel coverlids and wheat straw was used as bedding material. The animals were kept at the animal house of Department of Biosciences, Saurashtra University, Rajkot. The animals were facilitated with standard environmental condition of photoperiod (12:12 h dark: light cycle) and temperature (25 ± 2°C). They were provided with commercial rat and mice feed (Pranav Agro Industries Ltd., Amruth Brand rat & mice pellet feed) and water given ad libitum. The use of these animals and the study protocols were approved by CPCSEA-recognized local ethical committee.
Hepatoprotective study
The rats were divided into five groups with six rats in each. Group I (Control) served as normal and received the vehicle alone (sterile distilled water 10 ml/kg body weight, p.o.) for 5 days. Group II (toxin control) animals received APAP (3 g/kg, p.o.) on the fourth day. Groups III (WFM-400) and IV (WFM-600) were treated with WFM at a dose level of 400 mg/kg and 600 mg/kg body weight p.o. per day, respectively, for 5 days and, on the fourth day, APAP (3 g/kg, p.o.) was given 1 h after the treatment of the extract. Group V (standard) was treated with standard drug silymarin (100 mg/kg p.o.) for 5 days and, on the fourth day, APAP (3 g/kg, p.o.) was given 1 h after the treatment of the drug. The animals were sacrificed 48 h after the dose of acetaminophen under mild ether anesthesia. The blood was collected and allowed to stand for 30 min at 37°C and then centrifuged to separate the serum.
Preparation of liver homogenate
The liver was quickly removed and perfused immediately with ice-cold saline (0.9% NaCl). A portion of the liver was homogenized in chilled Tris-HCl buffer (0.025 M, pH 7.4) using a homogenizer. The homogenate obtained was centrifuged in a centrifuge at 5000 rpm for 10 min, supernatant was collected and used for various antioxidant enzyme assays.
Measurement of biochemical parameters and antioxidant enzymes
The separated serum was estimated for various biochemical parameters, including albumin (CitationCorcoran & Durnan, 1977), blood urea nitrogen (BUN; CitationFawcett & Scott, 1960), aspartate aminotransferase (AST; CitationReitman & Frankel, 1957), alanine aminotransferase (ALT; CitationReitman & Frankel, 1957), and alkaline phosphatase (ALP; CitationKind & King, 1954) levels. Glutathione peroxidase (GPx; CitationMills, 1959; CitationRotruck et al., 1973) activity, content of total protein (TP; CitationLowry et al., 1951), and GSH (CitationEllman, 1959) was determined in the liver homogenate. The absorbance of all the parameters was measured in a UV-VIS Spectrophotometer (Shimadzu, Tokyo, Japan).
Histopathological study
A portion of the liver was cut into ~6 mm size and fixed in phosphate buffered 10% formaldehyde solution. After embedding in paraffin wax, thin sections of 5 µm thickness of liver tissue were cut and stained with hematoxylin-eosin. The thin sections of liver were made into permanent slides and examined under Zeiss microscope with photographic facility and photomicrographs were taken.
Statistical analysis
The data obtained from animal experiments are expressed as mean ± SEM (standard error of mean). Graphs were prepared using GraphPad Prism and data were subjected to analysis of variance (ANOVA) followed by Dunnett’s t-test. Values are considered statistically significant at P < 0.05.
Results
Serum biochemical analysis
Oral administration of APAP caused significant liver damage as evidenced by altered biochemical parameters (). APAP (toxin control) significantly (P < 0.001) decreased serum level of albumin as compared to normal control group. The level of BUN in APAP-treated group increased but not to a significant level. APAP significantly (P < 0.001) enhanced AST, ALP levels and also ALT (P < 0.05) in toxin control group; about 3-fold increase was observed in ALT and AST levels in serum.
Figure 1. Effect of Woodfordia fruticosa methanol (WFM) on different biochemical parameters in acetaminophen (APAP)-induced hepatic damage in rats. Group I: Normal control, Group II: Toxin control APAP, Group III: WFM-400 mg/kg + APAP, Group IV: WFM-600 mg/kg + APAP, Group V: Silymarin-100 mg/kg + APAP. Results are expressed as mean ± SEM, (n = 6). #P < 0.05, ##P < 0.01, ###P < 0.001 as compared with normal control group; *P < 0.05, **P < 0.01, ***P < 0.01 as compared with toxin control group.
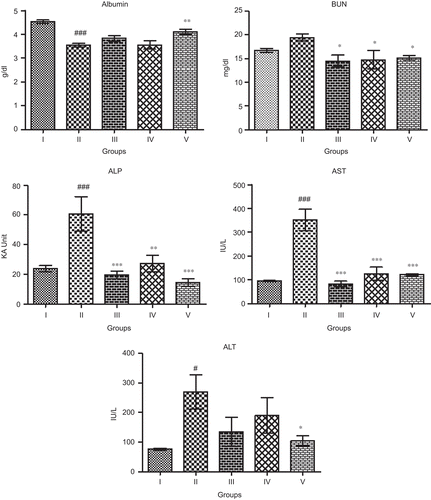
Treatment with WFM did not exhibit potential effect on recovery of albumin levels; while in standard drug-treated group, the level of albumin increased significantly (P < 0.01). BUN level decreased significantly (P < 0.05) in WFM-treated animals as compared to toxin control group. The ALP level also decreased significantly in lower as well as higher dose groups (P < 0.001, P < 0.01, respectively). 400 and 600 mg/kg of WFM-treated groups showed significant (P < 0.001) decrease in AST level as compared to toxin control group. The result of AST is better or similar to that of the standard drug-treated group (P < 0.001). ALT level decreased in WFM-treated groups toward normalization, though not significantly.
Liver antioxidant analysis
The result of relative liver weight, liver TP, GSH, and antioxidant enzyme GPx in APAP-induced hepatotoxicity are given in . The administration of APAP significantly increased the liver weight (P < 0.001) as compared to normal control group. Significant fall was observed in hepatic TP (P < 0.01). The administration of APAP decreased the hepatic non-enzymatic antioxidant GSH contents, but not to a significant level. The treatment of WFM decreased liver weight at both the dose levels, significant difference was observed at lower dose (P < 0.05) as compared to toxin control group. In higher dose, the level of hepatic TP increased significantly (P < 0.01). The hepatoprotective efficacy of the test drug was comparable with that of standard drug silymarin. WFM treatment enhanced the production of GSH toward normal control, but not to a significant level. Administration of APAP did not diminish the anti-oxidative status of hepatic GPx activity.
Figure 2. Effect of WFM on relative liver weight and different liver non-enzymatic and enzymatic antioxidants in APAP-induced hepatic damage in rats. Group I: Normal control, Group II: Toxin control APAP, Group III: WFM-400 mg/kg + APAP, Group IV: WFM-600 mg/kg + APAP, Group V: Silymarin-100 mg/kg + APAP. Results are expressed as mean ± SEM, (n = 6). #P < 0.05, ##P < 0.01, ###P < 0.001 as compared with normal control group; *P < 0.05, **P < 0.01, ***P < 0.01 as compared with toxin control group.
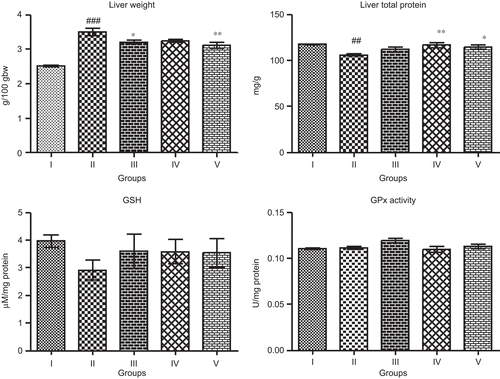
Histopathological study
Liver histopathological examination () showed no histological abnormalities in normal control liver; the hepatic lobular architecture was normal, connective tissue proliferation was not seen. In APAP-treated animals, the liver pathological changes were characterized by severe hepatocellular degeneration and necrosis along with periportal mononuclear cell infiltration due to APAP toxicity. WFM protected the liver tissue against APAP toxicity, with mild hepatocellular degeneration, necrosis, less inflammatory cell infiltration, and well-preserved hepatocytes were observed in most areas when compared with that of APAP group.
Discussion
APAP, a frequently used analgesic and antipyretic drug, is known to be hepatotoxic in high doses, which is primarily metabolized by sulfation and glucuronidation to unreactive metabolites and then activated by the cytochrome P450 system to produce liver injury. It is established that APAP is bioactivated to a toxic electrophile, N-acetyl p-benzoquinone imine (NAPQI), which binds covalently to tissue macromolecules, and probably also oxidizes lipids, or the critical sulphydryl groups (protein thiols) and alters the homeostasis of calcium (CitationLin et al., 1997). The massive production of reactive species may lead to depletion of protective physiological moieties (glutathione and α-tocopherol, etc.), ensuing wide-spread propagation of the alkylation as well as peroxidation, causing damage to the macromolecules in vital biomembranes (CitationAldridge, 1981; CitationGilani et al., 2005). The experimental evidence suggests that during metabolism of this type of drug, different reactive metabolites are produced that covalently modify proteins (CitationBernareggi, 1998), impose oxidative stress (CitationBerson et al., 1991; CitationRitter & Malejka-Giganti, 1998), and causes mitochondrial injury (CitationMingatto et al., 2000).
In the present study, a reduction in serum albumin level observed in the APAP-treated rats may be associated with the decrease in the number of hepatocytes which in turn may result into decreased hepatic capacity to synthesize albumin. But the treatment with WFM did not have protective effects in regard to albumin levels. BUN is also a marker of liver and renal functions, which is used to diagnose acute and chronic diseases related to liver and kidney. APAP administration to the rats increased the BUN level. The increase in BUN after APAP administration was prevented by WFM.
The hepatic cells consist of higher concentrations of AST, ALT, and ALP in cytoplasm and AST in particular exists in mitochondria (CitationWells, 1988). Due to the damage caused to hepatic cells, the leakage of plasma cause an increased level of hepatospecific enzymes in serum (CitationZimmerman & Seef, 1970). The elevated serum enzyme levels are indicative of cellular leakage and functional integrity of cell membrane in liver (CitationDrotman & Lawhorn, 1978; CitationSathesh Kumar et al., 2009). The hepatoprotective index of a drug can be evaluated by its capability to reduce the injurious effects or to preserve the normal hepatic physiological mechanisms, which have been induced by a hepatotoxin. The measurement of serum AST, ALT, and ALP levels serves as a means for indirect assessment of condition of liver. The level of these enzymes significantly increased in serum, when animals were administered with APAP. The pretreatment of the animals with WFM with respect to intoxication with APAP controlled the AST, ALT, and ALP levels when compared with the toxic group.
The relative liver weight significantly increased in APAP-intoxicated animals indicating toxic effect of APAP. Pretreatment of WFM decreased the liver weight significantly indicating recovery of liver tissue from damage. GSH, a major known protein thiol in living organisms, plays a central role in coordinating the body’s antioxidant defense process (CitationBoyd et al., 1981). Excessive peroxidation causes increased GSH consumption. GSH is a scavenger of toxic metabolites, including NAPQI, which is a metabolite of APAP (CitationHwang et al., 2008; CitationYuan et al., 2010). GSH plays a major protective role as a scavenger of free radicals that combine with non-protein thiols at the GSH reactive center to abolish free radical toxicity (CitationSwierkosz et al., 1995). Antioxidation by GSH protects the body from many diseases and conditions such as damage by H2O2, ethanol, and numerous other toxins (CitationChoi et al., 2009). As GSH plays an important role in the antioxidant defense system (CitationHsu et al., 2008), it becomes the key determinant in the APAP-induced hepatotoxicity. In the present study, the contents of liver protein and GSH in the APAP-treated animals significantly decreased after APAP administration, when compared with control group. And as expected, pretreatment with WFM restored the total protein and GSH levels toward normalization. Administration of APAP as well as WFM did not have any toxic or potential effects in GPx activity.
Histopathological observations after APAP administration showed severe damage in hepatocytes, which basically supported the alterations observed in biochemical assays. Hepatocellular necrosis, infiltration of periportal mononuclear cell of liver cells, the characteristic alterations occurred due to APAP intoxication. Treatment of WFM decreased focal necrosis, vacuolation, and reduced the lymphocytic infiltration in liver and showed regenerative effects. This can be considered as an expression of the functional improvement of hepatocytes, which might be due to accelerated regeneration of parenchymal cells or little damage of cells.
In conclusion, on the basis of biochemical analysis and histopathological study, Woodfordia fruticosa flowers’ methanol extract can protect liver against APAP-induced hepatic necrosis in rats.
Acknowledgement
We are thankful to Mr. Vishavas Ranpariya for the help in histopathological analysis. One of the authors, Yogesh Baravalia is thankful to University Grants Commission, New Delhi, India for providing financial support as JRF.
Declaration of interest
The authors report no conflicts of interest.
References
- Ahuja BS. (1965). Medicinal Plants of Saharanpur. Gurukul Kangri Printing Press, Hardwar, India.
- Akah PA, Nwambie AI. (1994). Evaluation of Nigerian traditional medicines: 1. Plants used for rheumatic (inflammatory) disorders. J Ethnopharmacol, 42, 179–182.
- Akuodor GC, Idris-Usman MS, Mbah CC, Megwas UA, Akpan JL, Ugwu TC, Okoroafor DO, Osunkwo UA. (2010). Studies on anti-ulcer, analgesic and antipyretic properties of the ethanolic leaf extract of Gongronema latifolium in rodents. Afr J Biotechnol, 9, 2316–2321.
- Alam M, Susan T, Joy S, Ak SU, Kundu AB. (1990). Anti-inflammatory activity of Plectranthus urticifolius Hook. F. and Woodfordia fruticosa Kurz. in albino rats. Indian Drug, 27, 559–562.
- Aldridge WN. (1981). Mechanism of toxicity: New concepts are required in toxicology. Trends Pharmacol Sci, 2, 228–231.
- Atal CK, Bhatia AK, Singh RP. (1982). Role of Woodfordia fruticosa Kurz (Dhataki) in the preparation of asavas and aristhas. J Res Ayurveda Siddha, 3, 193–199.
- Bernareggi A. (1998). Clinical pharmacokinetics of nimesulide. Clin Pharmacokinet, 35, 247–274.
- Berson A, Wolf C, Berger V, Fau D, Chachaty C, Fromenty B, Pessayre D. (1991). Generation of free radicals during the reductive metabolism of the nitroaromatic compound, nilutamide. J Pharmacol Exp Ther, 257, 714–719.
- Bonkovsky HL, Kane RE, Jones DP, Galinsky RE, Banner B. (1994). Acute hepatic and renal toxicity from low doses of acetaminophen in the absence of alcohol abuse or malnutrition: evidence for increased susceptibility to drug toxicity due to cardiopulmonary and renal insufficiency. Hepatology, 19, 1141–1148.
- Boyd SC, Sasame HA, Boyd MR. (1981). Gastric glutathione depletion and acute ulcerogenesis by diethylmaleate given subcutaneously to rats. Life Sci, 28, 2987–2992.
- Chandan BK, Saxena AK, Shukla S, Sharma N, Gupta DK, Singh K, Suri J, Bhadauria M, Qazi GN. (2008). Hepatoprotective activity of Woodfordia fruticosa Kurz flowers against carbon tetrachloride induced hepatotoxicity. J Ethnopharmacol, 119, 218–224.
- Choi EY, Hwang HJ, Kim IH, Nam TJ. (2009). Protective effects of a polysaccharide from Hizikia fusiformis against ethanol toxicity in rats. Food Chem Toxicol, 47, 134–139.
- Corcoran RM, Durnan SM. (1977). Albumin determination by a modified bromcresol green method. Clin Chem, 23, 765–766.
- Das PK, Goswami S, Chinniah A, Panda N, Banerjee S, Sahu NP, Achari B. (2007). Woodfordia fruticosa: Traditional uses and recent findings. J Ethnopharmacol, 110, 189–199.
- Drotman RB, Lawhorn GT. (1978). Serum enzymes as indicators of chemically induced liver damage. Drug Chem Toxicol, 1, 163–171.
- Dymock W, Warden CJH, Hooper D. (1995). A History of the Principal Drugs of Vegetable Origin Met within British India Republic. Pharmacographia Indica, Vol. I. Vivek Vihar, Delhi, India.
- Ellman GL. (1959). Tissue sulfhydryl groups. Arch Biochem Biophys, 82, 70–77.
- Fawcett JK, Scott JE. (1960). A rapid and precise method for the determination of urea. J Clin Pathol, 13, 156–159.
- Gilani AH, Jabeen Q, Ghayur MN, Janbaz KH, Akhtar MS. (2005). Studies on the antihypertensive, antispasmodic, bronchodilator and hepatoprotective activities of the Carum copticum seed extract. J Ethnopharmacol, 98, 127–135.
- Hamlyn AN, Douglas AP, James O. (1978). The spectrum of paracetamol (acetaminophen) overdose: Clinical and epidemiological studies. Postgrad Med J, 54, 400–404.
- Hau DK, Gambari R, Wong RS, Yuen MC, Cheng GY, Tong CS, Zhu GY, Leung AK, Lai PB, Lau FY, Chan AK, Wong WY, Kok SH, Cheng CH, Kan CW, Chan AS, Chui CH, Tang JC, Fong DW. (2009). Phyllanthus urinaria extract attenuates acetaminophen induced hepatotoxicity: involvement of cytochrome P450 CYP2E1. Phytomedicine, 16, 751–760.
- Hsu CC, Lin KY, Wang ZH, Lin WL, Yin MC. (2008). Preventive effect of Ganoderma amboinense on acetaminophen-induced acute liver injury. Phytomedicine, 15, 946–950.
- Hwang HJ, Kim IH, Nam TJ. (2008). Effect of a glycoprotein from Hizikia fusiformis on acetaminophen-induced liver injury. Food Chem Toxicol, 46, 3475–3481.
- Khare CP. (2004). Encyclopedia of Indian Medicinal Plants: Rational Western Therapy, Ayurvedic and Other Traditional Usage. Botany, Berlin: Springer, pp. 483–484.
- Kind PR, King EJ. (1954). Estimation of plasma phosphatase by determination of hydrolysed phenol with amino-antipyrine. J Clin Pathol, 7, 322–326.
- Knight TR, Fariss MW, Farhood A, Jaeschke H. (2003). Role of lipid peroxidation as a mechanism of liver injury after acetaminophen overdose in mice. Toxicol Sci, 76, 229–236.
- Kumaraswamy MV, Satish S. (2008). Free radical scavenging activity and lipoxygenase inhibition of Woodfordia fructicosa Kurz and Betula utilis Wall. Afr J Biotechnol, 7, 2013–2016.
- Lin CC, Shieh DE, Yen MH. (1997). Hepatoprotective effect of the fractions of Ban-zhi-lian on experimental liver injuries in rats. J Ethnopharmacol, 56, 193–200.
- Lin J, Opoku AR, Geheeb-Keller M, Hutchings AD, Terblanche SE, Jäger AK, van Staden J. (1999). Preliminary screening of some traditional zulu medicinal plants for anti-inflammatory and anti-microbial activities. J Ethnopharmacol, 68, 267–274.
- Lowry OH, Rosebrough NJ, Farr AL, Randall RJ. (1951). Protein measurement with the Folin phenol reagent. J Biol Chem, 193, 265–275.
- Mills GC. (1959). The purification and properties of glutathione peroxidase of erythrocytes. J Biol Chem, 234, 502–506.
- Mingatto FE, dos Santos AC, Rodrigues T, Pigoso AA, Uyemura SA, Curti C. (2000). Effects of nimesulide and its reduced metabolite on mitochondria. Br J Pharmacol, 131, 1154–1160.
- Nadkarni KM. (1954). Indian Materia Medica, Vol. 2, 3rd ed. Popular Book Depot, Mumbai, India, p. 489.
- Parekh J, Chanda S. (2007). In vitro antibacterial activity of the crude methanol extract of Woodfordia fruticosa Kurz. flower (Lythraceae). Braz J Microbiol, 38, 204–207.
- Reitman S, Frankel S. (1957). A colorimetric method for the determination of serum glutamic oxalacetic and glutamic pyruvic transaminases. Am J Clin Pathol, 28, 56–63.
- Ritter CL, Malejka-Giganti D. (1998). Nitroreduction of nitrated and C-9 oxidized fluorenes in vitro. Chem Res Toxicol, 11, 1361–1367.
- Rotruck JT, Pope AL, Ganther HE, Swanson AB, Hafeman DG, Hoekstra WG. (1973). Selenium: Biochemical role as a component of glutathione peroxidase. Science, 179, 588–590.
- Sathesh Kumar S, Ravi Kumar B, Krishna Mohan G. (2009). Hepatoprotective effect of Trichosanthes cucumerina var cucumerina L. on carbon tetrachloride induced liver damage in rats. J Ethnopharmacol, 123, 347–350.
- Shah AS, Juvekar AR. (2010). In vitro and in vivo immunostimulatory activity of Woodfordia fruticosa flowers on non-specific immunity. Pharm Biol, 48, 1066–1072.
- Shome U, Mehrotra S, Sharma HP. (1981). Pharmacognostic studies on the flower of Woodfordia fruticosa Kurz. Proc Indian Acad Sc (Plant Science), 90, 335–351.
- Smilkstein MJ, Douglas DR, Daya MR. (1994). Acetaminophen poisoning and liver function. N Engl J Med, 331, 1310–1; author reply 1311.
- Swierkosz TA, Mitchell JA, Warner TD, Botting RM, Vane JR. (1995). Co-induction of nitric oxide synthase and cyclo-oxygenase: interactions between nitric oxide and prostanoids. Br J Pharmacol, 114, 1335–1342.
- Vermeulen NP, Bessems JG, Van de Straat R. (1992). Molecular aspects of paracetamol-induced hepatotoxicity and its mechanism-based prevention. Drug Metab Rev, 24, 367–407.
- Wells FE. (1988). Tests in liver and biliary tract disease, In: Gowenlock HA, ed. Varley’s Practical Clinical Biochemistry. CRC Press, Florida.
- Yoshida T, Chou T, Nitta A, Miyamoto K, Koshiura R, Okuda T. (1990). Woodfordin C, a macro-ring hydrolyzable tannin dimer with antitumor activity, and accompanying dimers from Woodfordia fruticosa flowers. Chem Pharm Bull, 38, 1211–1217.
- Yuan HD, Jin GZ, Piao GC. (2010). Hepatoprotective effects of an active part from Artemisia sacrorum Ledeb. against acetaminophen-induced toxicity in mice. J Ethnopharmacol, 127, 528–533.
- Zimmerman HJ, Seef LB. (1970). Enzymes in hepatic disease, In: Goodly EI, ed. Diagnostic Enzymology. Lea and Febiger, Philadelphia.