Abstract
Context: Centaurea L. (Astreaceae) species are used as herbal remedies in Turkey. Centaurea calolepis Boiss. is an endemic species of Anatolia that has not been subjected to phytochemical studies except essential oil analysis.
Objective: Secondary metabolite determination, isolation and structure elucidation of pure compounds were performed on C. calolepis. Cnicin, which is the main component of several Centaurea species, was tested for its in vitro anti-inflammatory, antioxidant and cytotoxic activities.
Materials and methods: Chloroform and methanol extracts of the aerial parts of C. calolepis were subjected to isolation process using column chromatography. The structures of the compounds were characterized by 1D- and 2D-NMR experiments. Thin–layer chromatography and high performance liquid chromatography were used in determination of phenolics. Cnicin was subjected to a panel of cellular assays to test for inhibition of nuclear factor κB (NF-κB), inducible nitric oxide synthase (iNOS), reactive oxygen species and cytotoxicity.
Results: Cnicin, lucenin-2, schaftoside and 3-O-feruloylquinic acid were isolated from C. calolepis extracts. Vicenin-2, vitexin, isovitexin, homoorientin, rutin, orientin, luteolin-7-O-glycoside and chlorogenic acid were determined in fractions. Cnicin showed inhibition of NF-κB and inhibition of iNOS activity with IC50 Values of 1.8 and 6.5 µM, respectively. Cytotoxic activity of cnicin was observed toward pig kidney epithelial (LLC-PK11), human malignant melanoma (SK-MEL) and human ductal carcinoma (BT-549) cells with IC50 values of 23.3, 14.0 and 18.3 µM, respectively.
Discussion and conclusion: This is the first detailed report of secondary metabolites of C. calolepis. Evaluation of biological activity of cnicin establishes the potential of this compound as an anti-inflammatory and cytotoxic agent.
Introduction
The genus Centaurea L. (Asteraceae) is represented by 192 taxons in Turkish flora, and 61.1% of them are endemic (CitationWagenitz, 1975; CitationUysal et al., 2007; CitationUysal, 2008). Centaurea calolepis Boiss. [Sect: Acrolophus (Cass.) DC.] is an endemic species distributed in West and South West Anatolia (CitationWagenitz, 1975). The plant is not used in Turkish traditional medicine; however, various species of Centaurea are used as herbal remedies for their tonic, digestive, expectorant, antipyretic and antidiarrheal effects (CitationBaytop, 1999). Only one chemical study performed on C. calolepis describes the essential oil composition of the plant, which includes hexadecanoic acid and carvacrol as main components (CitationKaramenderes et al., 2008). To our knowledge, no additional phytochemical study has been reported on C. calolepis. Previously, antioxidant, anti-inflammatory (CitationKaramenderes et al., 2007a), antileishmanial and antimicrobial (CitationKaramenderes et al., 2006) activities of the extracts obtained from the aerial parts of C. calolepis were investigated.
As a continuation of our research on Turkish Centaurea species (CitationKaramenderes et al., 2006; Citation2007a,Citationb,Citationc; Citation2008), in this study, we report the isolation and structure elucidation of cnicin, lucenin-2, shaftoside and 3-O-feruloylquinic acid from C. calolepis (1–4). In addition, seven flavonoids (vicenin-2, vitexin, isovitexin, homoorientin, rutin, orientin, luteolin-7-O-glycoside) and one phenolic acid (chlorogenic acid) were determined in fractions by direct thin layer chromatography (TLC) and high performance liquid chromatography (HPLC) comparisons with authentic samples (5–11). A sesquiterpene lactone cnicin, known for its anti-inflammatory (CitationSchneider & Lachner, 1987), antifungal (CitationBarrero et al., 2000), antibacterial and cytotoxic activities (CitationBarrero et al., 1995; CitationSaroglou et al., 2005), is the main compound of several Centaurea species (CitationGousiadou & Skaltsa, 2003; CitationDjeddi et al., 2008) and probably their pharmacological properties are due on this substance. However, biological activities of this germacrenolide are not completely elucidated. To get an insight into the anti-inflammatory potential of cnicin (1), its effects on the activity of nuclear factor κB (NF-κB), inducible nitric oxide synthase (iNOS) and intracellular generation of reactive oxygen species (ROS) as a result of oxidative stress were investigated in SW1353, RAW 264.7 and HL-60 cells, respectively. These cell lines are stimulated by inflammatory signals such as phorbol myristate acetate (PMA) and lipopolysaccharide (LPS) and have been used as representative models in previously described procedures (CitationQuang et al., 2006; CitationKaramenderes, 2007a; CitationReddy et al., 2007; CitationAnkisetty et al., 2010). Cytotoxicity against a panel of human solid tumor cell lines as well as noncancerous monkey kidney fibroblast and pig kidney epithelial cells was also determined.
Materials and methods
All cells were obtained from ATCC (Rockville, MD). DCFH-DA, RPMI-1640 and DMEM/F12 media were from Invitrogen (Carlsbad, CA). Fetal bovine serum (FBS) and bovine calf serum were from Atlanta Biologicals (Lawrenceville, GA). All other chemicals and reagents were from Sigma (St. Louis, MO).
General experimental procedures
NMR spectra were run on a Varian instrument operating at 400 MHz and 100 MHz for 1H and 13C, respectively, and or on a Bruker DRX 600 (Bruker BioSpin, Rheinstetten, Germany) operating at 600 MHz (1H) and 150 MHz (13C). The NMR data were measured in CD3OD and DMSO-d6. Proton and carbon chemical shifts are relative to TMS. 2D-NMR spectra [heteronuclear multiple quantum coherence, heteronuclear multiple-bond correlation (HMBC)] were run using standard Varian or Bruker pulse programs. Mass spectra were recorded on 3200 Q TRAP (AB Sciex, Foster City, CA) spectrometer with ESI Turbo Spray Source. Column chromatography was carried out on Silica gel (40 µm, Merck, Darmstadt, Germany), Sephadex LH-20 (GE Healthcare Bio-Sciences AB, Uppsala, Sweden), Polyamide (Fluka Chemie AG, Buchs, Switzerland), RP C-18 (25–40 µm, Merck, Darmstadt, Germany) and Mega Bond RP-18 columns (Varian, Darmstadt, Germany). TLC analyses were carried out on Silica gel 60 F254 (Merck, Darmstadt, Germany) and RP C-18 F254 (Merck, Darmstadt, Germany) plates. High Speed Counter Current Chromatography (PharmaTech CCC1000, Baltimore, MD) system was also used in fractionation of the extracts. Compounds were detected by ultraviolet (UV) (254 and 366 nm) and 1% vanillin/H2SO4 spraying reagent followed by heating at 105°C for 1–2 min. Natural Products Polyethylene Glycol Reagent (NPR, Roth, Karlsruhe, Germany) was also used for detection of flavonoids. HPLC system equipped with Perkin Elmer 200 pomp and autosampler, Perkin Elmer (Norwalk, CT) UV-VIS detector and Turbochrom Navigator Software was used. Reversed phase Agilent Hypersil BDS, 5 µm particle size, in a 250 mm × 4 mm i.d. stainless steel column was used. Flow rate was 1 ml/ml. Gradient elution was performed with H2O (System A) and acetonitrile/H2O (80:20 v/v) (System B). The gradient conditions for the analysis of flavon diglycosides were 13% B (5 min), 13–16% B (30 min), 16–18% B (15 min) and 18–100% B (35 min). The conditions for the analysis of flavon monoglycosides were 13% B (5 min), 5–20% B (20 min), 20% B (25 min) and 2–26% B (20 min). Detections were at 270 nm and 340 nm. Aliquots of the fractions (10 µl) were injected into the HPLC system. Each analysis was carried out triplicate. Authentic samples used for TLC and HPLC comparisons were obtained from Extrasynthese (Genay, France).
Plant material
Aerial parts of C. calolepis were collected from Karacasu, Denizli, Turkey, in June 2004. A voucher specimen identified by Prof. Dr. Ozcan SECMEN, from Section of Botany, Department of Biology, Faculty of Science, Ege University, is deposited at the Herbarium of Ege University, Faculty of Pharmacy, Izmir, Turkey (IZEF5668).
Extraction and isolation
Dried and ground aerial parts (1200 g) of the plant were extracted with n-hexane, CHCl3 and MeOH at room temperature in an ultrasonic bath (3 × 4 l, 48 h). The combined extracts were evaporated separately under reduced pressure to dryness, which afforded 9.8, 36.0, and 97.0 g, respectively. A portion of CHCl3 extract (20 g) was dissolved in CH2Cl2 and partitioned with MeOH-H2O (1:1) two times. The residue of the apolar phase was submitted to silica gel column chromatography eluted with CH2Cl2 and then CH2Cl2-acetone mixtures (10%→50%, 800 ml, each), CH2Cl2-MeOH mixtures (10%→100%, 800 ml, each) and finally with 100% MeOH. After combining 87 fractions, Fr. 32–34 (205 mg) were selected for further purification. Fifty milligram of the fraction was subjected to vacuum liquid chromatography by RP-18 column (12 ml) to give pure cnicin (1, 5 mg).
MeOH extract (78.6 g) was suspended in H2O and then partitioned successively with EtOAc (300 ml × 3). The residue of the H2O layer (40.14 g) was repartitioned with n-BuOH (300 ml × 3). The concentrated and lyophilized H2O layer (24.16 g) was subjected to a polyamide column, eluted with water and subsequently with H2O:MeOH mixtures (10%→100% MeOH). Six hundred and thirty fractions were collected and combined into 15 fractions according to TLC results. Fr. 301–320 (187 mg) was applied to high speed counter current chromatography using solvent system EtOAc:BuOH:H2O (2:1:2) in the ascending mode to give two flavone glycosides; lucenin-2 (2, 3.2 mg) and schaftoside (3, 11.2 mg). Fr. 581–630 (74 mg) was submitted to silica gel column chromatography by elution with CHCl3-MeOH (10%→100% MeOH, 100 ml, each) to afford 242 fractions. Fractions 132–162 were combined to give pure 3-feruloylquinic acid (4, 7 mg). In addition, seven flavonoids; vicenin-2 (5), vitexin (6), isovitexin (7), homoorientin (8), rutin (9), orientin (10), luteolin-7-O-glycoside (11) and one phenolic acid; chlorogenic acid (12) were determined in fractions 221–300, 321–350, 441–500, 501–530 and 561–580 by the direct TLC and HPLC comparisons with authentic samples. Compound 4 and 12 are also compared by TLC on silica plate to identify these two phenolic acids [solvent system: 100:11:11:26 (EtOAc:formic acid:acetic acid:H2O), UV: 366 nm, reagent: NPR].
Assay for inhibition of NF-κB activity
The assay was performed in human chondrosarcoma (SW1353) cells as described earlier (CitationKaramenderes et al., 2007a). Cells were cultured in 1:1 mixture of DMEM/F12 supplemented with 10% FBS, 100 U/ml penicillin G sodium and 100 µg/ml streptomycin at 37°C in an atmosphere of 5% CO2 and 95% humidity. Cells (1.2 × 107) were washed once in an antibiotic and FBS-free DMEM/F12, and then resuspended in 500 µl of antibiotic-free DMEM/F12 containing 2.5% FBS. NF-kB luciferase plasmid construct was added to the cell suspension at a concentration of 50 µg/ml and incubated for 5 min at room temperature. The cells were electroporated at 160 V and one 70-ms pulse using BTX disposable cuvettes model 640 (4 mm gap) in a BTX Electro Square Porator T 820 (BTX I, San Diego, CA). After electroporation, cells were plated to the wells of 96-well plate at a density of 1.25 × 105 cells per well. After 24 h, cells were treated with different concentrations of test compound (cnicin or parthenolide as a positive control) for 30 min and then induced with PMA (70 ng/ml) for 8 h. Cells were lysed by adding 40 µl of a 1:1 mixture of lucLite reagent and phosphate buffered saline containing 1 mM calcium and magnesium (Packard Instrument Company, Meriden, CT). Light output was detected on a SpectraMax plate reader.
Assay for inhibition of iNOS activity
The assay was performed in mouse macrophages (RAW264.7) cultured in phenol red–free RPMI medium with 10% bovine calf serum and 100 U/ml penicillin G sodium, and 100 µg/ml streptomycin as above. Cells were seeded in 96-well plates (50,000 cells/well) and incubated for 24 h for a confluency of 75% or more. Test samples (cnicin or parthenolide) diluted in serum-free medium were added, and after 30 min of incubation, LPS (5 µg/ml) was added and cells were further incubated for 24 h. The concentration of nitric oxide produced as a result of iNOS activity was determined by measuring the level of nitrite in the cell culture supernatant by using Griess reagent (CitationQuang et al., 2006, CitationAnkisetty et al., 2010). Percent inhibition of nitrite production by the test compound was calculated in comparison to vehicle control as an index of inhibition of iNOS activity by the test compound. IC50 value (the concentration that causes an inhibition of 50%) was obtained from dose curve. Parthenolide, a sesquiterpene lactone, which is known to inhibit iNOS (CitationFukuda et al., 2000), was used as positive control. In parallel to inhibition of iNOS activity, cytotoxicity of test compounds toward macrophages was also determined by measuring the viability of the cells at the end of 24 h incubation period by neutral red assay (as described below for in vitro cytotoxicity).
Assay for antioxidant activity
Inhibition of intracellular generation of ROS was assayed in myelomonocytic HL-60 cells. Cells were seeded in 96-well plates (100,000 cells/well) and treated with test samples for 30 min. Cells were then stimulated with PMA (100 ng/ml) for 30 min. ROS generation is determined by using 2′,7′-dichlorfluorescein-diacetate as described earlier (CitationReddy et al., 2007). The IC50 values were calculated from dose curves of the percent 2′,7′-dichlorfluorescein production versus test concentrations. Vitamin C and Trolox (a water soluble derivative of vitamin E), known for their antioxidant potential, were used as positive controls.
Assay for in vitro cytotoxicity
Cnicin was tested for its in vitro cytotoxicity against a panel of four human solid tumor cell lines (SK-MEL malignant melanoma; KB oral epidermal carcinoma; BT-549 breast ductal carcinoma and SK-OV-3 ovary carcinoma) and two noncancerous kidney cell lines (Vero monkey kidney fibroblast and LLC-PK11 pig kidney epithelial cells). These cell lines have been routinely used as the representative mammalian cell lines to test cytotoxic potential of compounds under investigation (CitationMustafa et al., 2004; CitationAnkisetty et al., 2010). All cell lines were obtained from ATCC. Cells (25,000 cells/well) were seeded to the wells of 96-well plate and incubated for 24 h for confluency. Test samples or positive control were added at different dilutions and cells were incubated for 48 h. The number of viable cells was determined using the supravital dye neutral red according to a modification of the procedure of CitationBorenfreund et al. (1990) as described earlier (CitationMustafa et al., 2004). Briefly, the cells were washed with saline and incubated for 3 h with a solution of neutral red. The cells were washed again to remove extracellular dye. A solution of acidified ethanol was added to liberate the incorporated dye from viable cells and the absorbance was read at 450 nm. IC50 value (the concentration that causes a 50% decrease in cell viability) was obtained from dose response curve of percent viability versus test concentrations. Doxorubicin, a cytotoxic drug, was used as positive control.
Statistical analysis
All experiments were performed in duplicates. Data is represented as mean ± SEM of three determinations.
Results and discussion
The compounds isolated from the aerial parts of C. calolepis were identified by comparison of their NMR data ( and ) with those previously described in the literature (CitationSeikel & Mabry, 1965; Österdahl, 1978; CitationRustaiyan et al., 1982; CitationMorishita et al., 1984; CitationXie et al., 2003) as cnicin (1), lucenin-2 (2), shaftoside (3) and 3-O-feruloylquinic acid (4) ().
Table 1. 1H-NMR data obtained with compounds 1–4 (δ ppm).
Table 2. 13C-NMR data obtained with compounds 1–4 (δ ppm).
Figure 2. Chemical structures of compounds of Centaurea calolepis determined by direct thin–layer chromatography and high performance liquid chromatography comparisons with authentic samples.
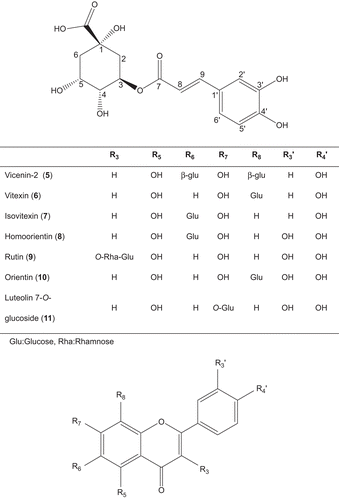
Compound 1 (m/z 378; molecular formula C20H26O7) is the main compound of several Centaurea species (CitationTesevic et al., 2007). Up to now, more than 40 species placed in Acrolophus D.C. section have been reported with the presence of germacrenolide cnicin (CitationGousiadou & Skaltsa, 2003; CitationDjeddi et al., 2008). Our result was in agreement with the previous studies.
Compound 2 (m/z 610; C27H30O16) was characterized by 2D-NMR experiments (including DQF-COSY, total correlation spectroscopy, nuclear overhauser enhancement spectroscopy, heteronuclear single quantum correlation and HMBC). At room temperature, two mostly broad signal sets were observed due to restricted rotation (CitationButaud et al., 2006) hampering the detailed analysis. Therefore, all measurements were done in DMSO-d6 at elevated temperature above coalescence (55°C) on a 600 MHz NMR spectrometer. In the literature, 1H-NMR data are only available for the trimethylsilylether at low frequency (CitationSeikel & Mabry, 1965; Österdahl, 1978) and 13C-NMR chemical shifts were assigned only tentatively (Österdahl, 1978). So the NMR data for lucenin-2 are presented here: 1H-NMR (600 MHz, δ), aglycone: 7.432 (1H, dd, 8.2 Hz, 2.0 Hz, H-6′), 7.396 (1H, d, 2.0 Hz, H-2′), 6.823 (1H, d, 8.2 Hz, H-5′), 6.438 (1H, s, H-3); glucose at position 6: 4.702 (1H, d, 9.8 Hz, H-1), 3.889 (1H, broad, H-2), 3.666 (1H, dd, 11.8 Hz, 2.1 Hz, H-6), 3.536 (1H, dd, 11.8 Hz, 4.7 Hz, H-6), 3.268 (1H, m, H-4), 3.266 (1H, m, H-3), 3.240 (1H, m, H-5); glucose at position 8: 4.843 (1H, d, 9.7 Hz, H-1), 3.829 (1H, t, 9.7 Hz, H-2), 3.753 (1H, d, 11.2 Hz, H-6), 3.581 (1H, dd, 11.2 Hz, 5.7 Hz, H-6), 3.379 (1H, m, H-4), 3.338 (1H, m, H-3), 3.319 (1H, m, H-5); 13C NMR (150 MHz, δ extracted from HSQC and HMBC spectra), aglycone (for C-4 and C-10 no HMBC cross peaks observed): 163.5 (C-2), 159.7 (C-5 and C-7), 155.3 (C-9), 150.6 (C-3′), 146.3 (C-4′), 121.9 (C-1′), 119.4 (C-6′), 116.0 (C-5′), 113.6 (C-2′), 108.8 (C-6), 105.1 (C-8), 102.1 (C-3); glucose at position 6: 81.2 (C-5), 79.0 (C-3), 74.4 (C-1), 71.6 (C-2), 70.4 (C-4), 61.3 (C-6); glucose at position 8: 82.0 (C-5), 79.2 (C-3), 74.8 (C-1), 72.0 (C-2), 70.8 (C-4), 61.7 (C-6) ( and ).
C-Diglycosides are not very common in Centaurea species. This is the first report of lucenin-2 from Centaurea genus. Also among Asteraceae family there are only a limited number of reports of this compound (CitationKroschewski et al., 1969; CitationSaleh et al., 1985; CitationGobbo-Neto & Lopes, 2008).
Compound 3 (m/z 564; molecular formula C26H28O14), another flavon C-diglycoside was previously isolated from C. horrida (CitationFlamini et al., 2002), C. solstitialis (CitationKamanzi and Raynaud, 1976), C. melitensis (CitationKamanzi et al., 1983) and C. hierapolitana (CitationKaramenderes et al., 2007b). Concerning the phenolic glycosides, even though there have been some reports on chlorogenic acid presence in several Centaurea species (CitationFlamini et al., 2004; CitationShoeb et al., 2007), this is also a first report of its metabolite, 4 (m/z 368; molecular formula C17H20O9), for the genus.
Seven flavonl glycosides (5–11) and one phenolic acid (12) were determined in fractions of C. calolepis by the direct TLC and HPLC comparisons with authentic samples (). These compounds were previously isolated from several Centaurea species; 5 from C. montana (CitationGonnet, 1992), C. incana (CitationAkkal et al., 1997), C. nicaensis (CitationAthmani et al., 1998) and C. horrida (CitationFlamini et al., 2002); 6 from C. thracica (CitationPutun et al., 1989), C. montana (CitationGonnet, 1992) and C. horrida (CitationFlamini et al., 2002); 7 from C. thracica (CitationPutun et al., 1989), C. macrocephala (CitationReynaud et al., 1992; CitationRibeiro et al.; 2002), C. virgata (Oksüz et al., 1984) and C. horrida (CitationFlamini et al., 2002); 9 from C. macrocephala (CitationReynaud et al., 1992), C. incana (CitationAkkal et al., 1997) and C. horrida (CitationFlamini et al., 2002); 8 and 10 from C. solstitialis (CitationKamanzi and Raynaud, 1976), C. melitensis (CitationKamanzi et al., 1983), C. thracica (CitationPutun et al., 1989), C. montana (CitationGonnet, 1992), C. horrida (CitationFlamini et al., 2002) and C. gigentea (CitationShoeb et al., 2007) and 11 from C. thracica (CitationPutun et al., 1989) and C. melitensis (CitationKamanzi et al., 1983). It is interesting that among Turkish Acrolophus D.C. section, only one flavon-C-glycoside (isovitexin) has been reported from C. virgata, which is defined as the closest species to C. calolepis from taxonomical point of view (CitationWagenitz, 1975).
Sesquiterpene lactones are regarded as one of the major classes of natural compounds with a wide spectrum of biological activities including anti-inflammatory and cytotoxic activities (CitationPicman, 1986; CitationBork et al., 1997 ). It is known that the wide range of biological activities of sesquiterpene lactones against mammalian cells lies basically in their α-methylene-γ-lactone and is enhanced by an additional Michael acceptor group (CitationHoffmann & Rabe, 1985). Sesquiterpene lactones are generally known to impair the activity of the NF-κB (CitationBork et al., 1997). Chloroform extracts of the aerial parts of C. calolepis were previously tested for its in vitro anti-inflammatory activity in the reporter gene assay for inhibition of transcription factor NF-κB and showed strong effect with IC50 value of 4.4 µg/ml (CitationKaramenderes et al., 2007a). In this study, 1 isolated from CHCl3 extract of C. calolepis showed strong inhibition of NF-κB activity in PMA-induced SW1353 cells with an IC50 of 1.8 µM as compared to the IC50 of 6.4 µM for parthenolide used as a positive control in the same assay. Parthenolide is a sesquiterpene lactone that is known for its anti-inflammatory action through inhibiting NF-κB activity (CitationSheehan et al., 2002). A luciferase construct with binding sites for Sp-1 was used as a control transcription factor, which is relatively unresponsive to inflammatory mediators (such as PMA). Compound 1 inhibited Sp-1–dependent luciferase expression at about 10-fold higher concentration (IC50 16 µM), which could be due to its cytotoxic effect. Parthenolide also inhibited Sp-1 activity at much higher concentration (IC50 49.3 µM). iNOS plays a regulatory role in the expression of proinflammatory mediators in the process of inflammatory pathway (CitationHye-Jung et al., 2008). Compound 1 showed an inhibition of iNOS activity in LPS-induced macrophages (RAW 264.7 cells) with IC50 of 6.5 µM. The activity was less potent than the activity of parthenolide (IC50 1.7 µM) in the same assay. However, compound 1 was more toxic to macrophages than parthenolide (IC50 7.9 µM vs 41.3 µM, ). In the antioxidant assay, no effect was observed on PMA-induced intracellular generation of ROS in HL-60 cells. Although in vivo anti-inflammatory properties of cnicin were reported earlier in a rat paw edema test (CitationSchneider & Lachner, 1987), this in vitro study indicates that the action of cnicin could be mediated by inhibition of NF-κB and iNOS activities. The effect of cnicin on NF-kB was stronger than the effect of parthenolide whereas parthenolide was more effective in inhibiting iNOS activity. The inhibition of iNOS activity also seems to be associated with toxicity that was observed toward macrophages ().
Table 3. Inhibition of NF-κB, Sp-1, iNOS and toxicity to macrophages by cnicin (values are IC50 in µM).
Cnicin was tested against mammalian kidney fibroblasts (Vero) and kidney epithelial (LLC-PK11) cells to determine its general cytotoxic effect. It was also tested for its in vitro anticancer potential in four human solid tumor cell lines namely malignant melanoma (SK-MEL), oral epidermal carcinoma (KB), breast ductal carcinoma (BT-549) and ovary carcinoma (SK-OV-3). Cnicin was not toxic to Vero cells but showed mild toxicity toward LLC-PK11 cells (IC50: 23 µM). Among the cancer cell lines, cytotoxicity was observed toward SK-MEL and BT-549 cell lines (IC50: 14 and 18 µM, respectively) as shown in . The cytotoxicity of cnicin was much less potent than cytotoxic drug doxorubicin. Previously, cnicin was tested against five other cell lines, namely DLD1 (colon cancer), SF268 (CNS), MCF7 (breast cancer), H460 (non–small cell lung cancer) and OVCAR3 (ovarian cancer) and was found to be active against MCF7 and OVCAR3 (CitationSaroglou et al., 2005). CitationBarrero et al. (1995) also reported the cytotoxic activity of cnicin against the P-388 (leukemia), A-549 (lung carcinoma) and HT-29 (colon carcinoma) cell lines. However, this is the first report of the cytotoxic activity of compound 1 against LLC-PK11, SK-MEL and BT-549 cell lines.
Table 4. Cytotoxic activity of cnicin (values are IC50 in µM).
In conclusion, to our knowledge this is the first detailed report on the secondary metabolites of the endemic species C. calolepis. Evaluation of biological activity of cnicin in the present study establishes the potential of cnicin as an anti-inflammatory and cytotoxic agent. However, the effect of cnicin on the anti-inflammatory targets was found to be associated with mild toxicity in both chondrocytes and macrophages and the effect of cnicin on NF-kB was more specific than its effect on iNOS.
Acknowledgements
We thank Prof. Dr. Özcan Seçmen and Serdar Şenol for their valuable assistance for collection and identification of the plant material. Technical assistance from Mr. John Trott, Mr. Paul Bates and Ms. Katherine Martin in performing bioassays is also acknowledged.
Declaration of interest
This research was supported by TUBITAK (The Scientific and Technical Council of Turkey) research project (Nr.106S197) and TUBITAK International PhD fellowship program.
References
- Akkal S, Benayache F, Benayache S, Jay M. (1997). Flavonoids from Centaurea incana (Asteraceae). Biochem Syst Ecol, 25, 361–62.
- Ankisetty S, Gochfeld D J, Diaz M C, Khan S I and Slattery M. (2010). Chemical constituents of the deep reef Caribbean sponges Plakortis angulospiculatus and Plakortis halichondrioides, and their anti-inflammatory activities. J Nat Prod, 73, 1494–98.
- Athmani G, Benayache F, Benayache S, Dendougui H, Jay M. (1998). Flavonoids extracted from Centaurea nicaeensis All. J Soc Alger Chim, 8, 29–36.
- Barrero AF, Oltra JE, Alvarez M, Raslan DS, Saude DA, Akssira M. (2000). New sources and antifungal activity of sesquiterpene lactones. Fitoterapia, 71, 60–64.
- Barrero AF, Oltra JE, Rodriguez I, Barragan A, García-Grávalos D, Ruiz P. (1995). Lactones from species of Centaurea. Cytotoxic and antimicrobial activities. Fitoterapia, 66, 227–30.
- Baytop T.(1999). Türkiye’de Bitkiler ile Tedavi. Istanbul, Turkey: Nobel Tıp Kitabevleri.
- Borenfreund E, Babich H, Martin-Alguacil N. (1990). Rapid chemosensitivity assay with human normal and tumor cells in vitro. In vitro Dev Cell Biol, 26, 1030–34.
- Bork PM, Schmitz ML, Kunt M, Escher C, Heinrich M. (1997). Sesquiterpene lactone containing Mexican Indian medicinal plants and pure sesquiterpene lactones as potent inhibitors of transcription factor NF-κB. FEBS Lett, 402, 85–90.
- Butaud JF, Raharivelomanana P, Bianchini JP, Faure R, Gaydou EM. (2006). Leaf C-glycosylflavones from Santalum insulare (Santalaceae). Biochem Syst Ecol, 34, 433–35.
- Djeddi S, Argyropoulou C, Skaltsa H. (2008). Secondary metabolites from Centaurea grisecachii ssp. grisebachii. Biochem Syst Ecol, 36, 336–39.
- Flamini G, Bulleri C, Morelli I. (2002). Secondary constituents from Centaurea horrida and their evolutionary meaning. Biochem Syst Ecol, 30, 1051–54.
- Flamini G, Stoppelli G, Morelli I, Ertugrul K, Dural H, Tugay O, Demirelma H. (2004). Secondary metabolites from Centaurea isaurica from Turkey and their chemotaxonomical significance. Biochem Syst Ecol, 32, 553–57.
- Fukuda K, Hibiya Y, Mutoh M, Ohno Y, Yamashita K, Akao, S, Fujiwara H. (2000). Inhibition by parthenolide of phorbol ester-induced transcriptional activation of inducible nitric oxide synthase gene in a human monocyte cell line THP-1. Biochem Pharmacol, 60, 595–600.
- Gobbo-Neto L, Lopes Norberto P. (2008). Online identification of chlorogenic acids, sesquiterpene lactones, and flavonoids in the Brazilian arnica Lychophora ericoides Mart. (Asteraceae) leaves by HPLC-DAD-MS and HPLC-DAD-MS/MS and validated HPLC-DAD method for their simultaneous analysis. J Agric Food Chem, 56, 1193–1204.
- Gonnet JF. (1992). Flavonoid glycoside variation in wild specimens of Centaurea montana (Compositae). Biochem Syst Ecol, 20, 149–61.
- Gousiadou C, Skaltsa H. (2003). Secondary metabolites from Centaurea orphendia. Biochem Syst Ecol, 31, 389–96.
- Hoffmann HMR, Rabe J. (1985), Synthesis and biological activity of α-methylene-γ-butyrolactones. Angew Chem Int Ed Engl, 24, 94–110.
- Hye-Jung Y, Hyun-Jung K, Hyun-Joo J, Kyunghoon K, Chang-Jin L, Eun-Hee P. (2008). Anti-inflammatory, anti-angiogenic and anti-nociceptive activities of Saururus Chinensis extract. J Ethnopharmacol, 120, 282–86.
- Kamanzi K, Raynaud J. (1976). Contribution to the study of the flavone glycosides of Centaurea solstitialis L. Plant Med Phytother, 10, 78–84.
- Kamanzi K, Raynaud J, Voirin B. (1983). The C-glycosyl flavonoids from flowers of Centaurea melitensis L. (Compositae). Plant Med Phytother, 17, 47–51.
- Karamenderes C, Khan S, Tekwani BL, Jacob MR, Khan IA. (2006). Antiprotozoal and antimicrobial activities of Centaurea L. species growing in Turkey. Pharm Biol, 44, 534–39.
- Karamenderes C, Konyalioglu S, Khan S, Khan IA. (2007a). Total phenolic contents, free radical scavenging activities and inhibitory effects on the activation of NF-κB of eight Centaurea L. species. Phytother Res, 21, 488–91.
- Karamenderes C, Bedir E, Pawar R, Baykan S, Khan IA. (2007b). Elemenolide sesquiterpenes and eudesmane sesquiterpene glycosides from Centaurea hierapolitana. Phytochemistry, 68, 609–15.
- Karamenderes C, Bedir E, Abou-Gazar H, Khan IA. (2007c). Chemical constituents of Centaurea cadmea. Chem Nat Comp, 43, 694–95.
- Karamenderes C, Demirci B, Baser KHC. (2008), Composition of essential oils of ten Centaurea L. taxa from Turkey. J Ess Oil Res, 20, 342–49.
- Kroschewski JR, Mabry TJ, Markham KR, Alston RE. (1969). Flavonoids from the genus Tragopogan (Compositae). Phytochemistry, 8, 1495–98.
- Morishita H, Iwahashi H, Osaka N, Kido R. (1984), Chromatographic separation and identification of naturally occuring chlorogenic acids by 1H nuclear magnetic resonance spectroscopy and mass spectrometry. J Chrom A, 315, 253–60.
- Mustafa J, Khan SI, Ma G, Walker LA, Khan IA. (2004). Synthesis and anticancer activities of fatty acid analogs of podophyllotoxin. Lipids, 39, 167–72.
- Oksuz S, Ayyildiz H, Johansson C. (1984). 6-Methoxylated and C-glycosyl flavonoids from Centaurea species. J Nat Prod, 47, 902–3.
- Osterdahl BG. (1978). Chemical studies on Bryophytes. 19. Application of 13C NMR in structural elucidation of flavonoid C-glucosides from Hedwigia ciliata. Acta Chem Scand B, 32, 93–97.
- Picman AK. (1986). Biological activities of sesquiterpene lactones. Biochem Syst Ecol, 14, 255–81.
- Putun AE, Ogretir C, Putun E. (1989). C-and O-Glycosyl flavonoids of Centaurea thracica (Janka) Hayek. Turkish J Chem, 13, 293–98.
- Quang DN, Harinantenaina L, Nishizawa T, Hashimoto T, Kohchi C, Soma G, Asakawa Y. (2006). Inhibition of nitric oxide production in RAW 264.7 cells by azaphilones from Xylariaceous fungi. Biol Pharm Bull, 29, 34–37.
- Reddy MK, Gupta SK, Jacob MR, Khan SI, Ferreira D. (2007). Antioxidant, antimalarial and antimicrobial activities of tannin-rich fractions, ellagitannins and phenolic acids from Punica granatum. Planta Med, 73, 461–67.
- Reynaud J, Couble A, Raynaud J. (1992). Flavonoids from Centaurea macrocephala Muss. Puschk. Ex Willd. (Compositae). Pharmazie, 47, 51–52.
- Ribeiro NL, Nahar L, Kumarasamy Y, Mir Babayev Y, Sarker SD. (2002). Flavonoid-C-glucosides and a lignan from Centaurea macrocephala. Biochem Syst Ecol, 30, 1097–100.
- Rustaiyan A, Niknejad A, Aynehchi Y. (1982). Chemical constituents of Centaurea brugeriana. Planta Med, 44, 185–86.
- Saleh NAM, El-Negoumy SI, Abd-Alla MF, Abou-Zaid MM, Dellamonica G, Chopin J. (1985). Occurence of ecdysteroids in the genus Centaurea (Compositae): 20-Hydroxyecdysone from Centaurea moschata. Phytochemistry, 24, 201–3.
- Saroglou V, Karioti A, Demetzos C, Dimas K, Skaltsa H. (2005). Sesquiterpene lactones from Centaurea spinosa and their antibacterial and cytotoxic activities. J Nat Prod, 68, 1404–7.
- Schneider G, Lachner I. (1987). Analysis and action of cnicin. Planta Med, 53, 247–51.
- Seikel MK, Mabry TJ. (1965). A new type of glycoflavonoid from Vitex lucens. Tet Lett, 16, 1105–9.
- Shoeb M, Jaspars M, Macmanus SM, Celik S, Nahar L, Kong-Thoo-Lin P, Sarker SD. (2007). Anti-colon cancer potential of phenolic compounds from the aerial parts of Centaurea gigantea (Asteraceae). J Nat Med, 61, 164–69.
- Sheehan M, Wong H R, Hake P W, Malhotra V, O’Connor M, Zingarelli B. (2002). Parthenolide, an inhibitor of the nuclear factor-κB pathway, ameliorates cardiovascular derangement and outcome in endotoxic shock in rodents. Mol Pharmacol, 61, 953–63.
- Tesevic V, Milosavljevic S, Vajs V, Janackovicp Djordjevic, I, Jadranin M, Vuckovic I. (2007). Quantitative analysis of sesquiterpene lactone cnicin in seven Centaurea species wild-growing in Serbia and Montenegro using 1H-NMR spectroscopy. J Serb Chem Soc, 72, 1275–80.
- Uysal T, Demirelma H, Ertugrul K, Garcia-Jacas N, Alfonso S. (2007). Centaurea glabro-auriculata (Asteraceae), a new species from Turkey. Ann Bot Fennici, 44, 219–22.
- Uysal T. (2008). Centaurea ertugruliana (Asteraceae), a new species from Turkey. Ann Bot Fennici, 45, 137–40.
- Wagenitz G. (1975). Centaurea L. In: Davis PH ed. Flora of Turkey and the East Aegean Islands, vol 5. Edinburgh, Edinburgh University Press, 465–585
- Xie C, Veitch NC, Houghton PJ, Simmonds MSJ. (2003). Flavone C-glycosides from Viola yedoensis Makino. Chem Pharm Bull, 51, 1204–7.