Abstract
Context and objectives: Artocarpus altilis (Parkinson) Fosberg (Moraceae) (breadfruit) leaves are used as an antihypertensive remedy. We investigated the possible mechanisms of action of its aqueous extract and its effect on cytochromes P450 (CYP) enzyme activities.
Materials and methods: Intravenous administration of an aqueous leaf extract (20.88–146.18 mg/kg) of A. altilis on mean arterial pressure and heart rate were recorded via cannulation of the carotid artery on anaesthetized normotensive Sprague–Dawley rats. Recordings of the contractile activity of the aortic rings to the extract (0.71–4.26 mg/mL) were studied using standard organ bath techniques. Inhibitions of human CYP3A4 and CYP2D6 enzyme activities were evaluated by means of a fluorometric assay in 96 well plates using heterologously expressed microsomes.
Results: A. altilis caused significant (p < 0.05) hypotensive and bradycardiac responses unaffected by atropine (2 mg/kg) and mepyramine (5 mg/kg), but attenuated by propranolol (1 mg/kg) and N(G)-nitro-l-arginine methyl ester (5 mg/kg). The extract (0.71–4.26 mg/mL) significantly (p < 0.05) relaxed phenylephrine (10−9–10−4 M) and 80 mM KCl-induced contractions in endothelium intact and denuded aortic rings; and caused a significant (p < 0.05) rightward shift of the Ca2+ dose-response curves in Ca2+-free Kreb’s solution. Moderate inhibitions of cytochrome P450s (CYP3A4 and CYP2D6) enzyme activities with IC50 values of 0.695 ± 0.187 and 0.512 ± 0.131 mg/mL, respectively, were produced.
Conclusion: A. altilis exhibits negative chronotropic and hypotensive effects through α-adrenoceptor and Ca2+ channel antagonism. Drug adversity effects are unlikely if the aqueous leaf extract is consumed with other medications reliant on CYP3A4 and CYP2D6 metabolism. This study thus provides scientific evidence for the use of the breadfruit in the treatment of hypertension.
Introduction
A. altilis (Parkinson) Fosberg (Moraceae), commonly called breadfruit, is widely distributed in the tropical regions of Africa, The Caribbean, and Pacific islands (CitationRagone, 2001). Breadfruit has been identified as an alternative source of carbohydrate (CitationDeivanai & Bhore, 2010), in The West Indies, it is classified as a medicinal plant that affects blood pressure and relieves asthma (CitationMitchell & Ahmad, 2006).
In traditional medicine, the stem and bark are employed in the treatment of bone pain, maternal postpartum infections, stomachaches and digestive tract problems (CitationWhistler, 1985), also for the treatment of inflammation, diabetes mellitus, diarrhea, and tapeworm infection (CitationAdewole & Ojewole, 2007). It is used as an antibacterial, antitubercular, antiviral, antimalarial and antifungal agent (CitationBoonphong et al., 2007; CitationJagtap & Bapat, 2010). It is also reported to have antiatherosclerotic and antiplatelet properties (CitationWeng et al., 2006) as well as cytotoxic property against human cancer cells (CitationWang et al., 2007).
Phytochemicals isolated from A. altilis include prenylated flavones namely; cycloartocarpin, artocarpin, chaplashin, morusin, cudraflavone B, cycloartobiloxanthone, artonin E, cudraflavone C, artobiloxanthone and artoindonesianin (CitationBoonphong et al., 2007; CitationShamaun et al., 2010). Stilbenoids, arylbenzofurons and jacalin (CitationJagtap & Bapat, 2010), saponins, glycosides, dihydrochalchones (CitationWang et al., 2006) has also been isolated from this plant.
The use of complementary and alternative medicine has increased globally; many persons in developing countries depend on traditional healing remedies for health maintenance and therapeutic management of diseases (CitationGardner et al., 2000; CitationKristoffersen et al., 2008), there is also a high prevalence of herb–drug concomitant use (CitationDelgoda et al., 2010).
Given the ethnomedicinal use of the breadfruit plant and in particular its reported concomitant use with known hypertensive medications (CitationPicking et al., 2011), it was important to investigate its impact on drug metabolizing cytochrome P450s (CYPs) (a heme containing superfamily of enzymes responsible for the metabolism) (CitationClarke & Jones, 2008; CitationDelgoda & Westlake, 2004). Herbal remedies are known to affect the dynamics of drug and chemical interactions (CitationNwokocha et al., 2011, Citation2012) and these enzymes are of significant concern to drug–drug interactions.
There is therefore need for pharmacological validation of these medicinal plants to justify the bases of its usage. The study was undertaken to examine the effect of the aqueous leaf extract of A. altilis on blood pressure and determine the possible mechanisms of action in normotensive Sprague–Dawley rats. The study also evaluated the inhibitory impact of A. altilis extract on CYPs3A4 and 2D6 enzyme activities.
Materials and methods
Chemicals and reagents
All chemicals except those noted below were purchased from Sigma-Aldrich (St. Louis, MO). All CYP substrates and metabolites were purchased from Gentest Corporation (Worburn, MA). Escherichia coli membranes expressing human CYP2D6 and CYP3A4 co-expressed with CYP reductase were purchased from Cypex Ltd. (Dundee, UK).
Experimental animals
Male Sprague–Dawley rats, aged 12 weeks and weighing 300–350 g were obtained from the Animal House, Basic Medical Sciences, University of the West Indies, Mona Campus, Jamaica. They were housed in plastic cages (12 h light/dark cycles at 26 ± 2°C) and fed standard rat chow and tap water ad libitum. Ethical approval for the study was sought and obtained from the FMS/UHWI/UWI Ethics committee.
Plant material and extraction
Leaves of A. altilis (2 kg) were collected in January 2010 from the Parish of St. Thomas, Jamaica. Botanical identification of the plant was made by Mr. Patrick Lewis, Department of Botany, University of the West Indies, Mona Campus and a voucher specimen (AN 08, 10/11) of the plant material deposited in the department. The powdered leaves were macerated in distilled water and left overnight. It was filtered using Whatman No. 1 filter paper and the filtrate was evaporated to dryness by freeze drying. The dark brown solid residue of 7.13 g was stored in a capped container in a refrigerator at −4°C until ready for use.
Measurement of blood pressure and heart rate
Rats were anaesthetized with 15% urethane (8 mL/kg) intraperitoneally (i.p). The trachea was exposed and cannulated to facilitate easy respiration. A polyethylene catheter (PE 50) was inserted into the right jugular vein and another catheter was inserted into the left carotid aorta and connected to a pressure transducer (Statham P23XL) and a Grass Polygraph (Model 7D, Quincy, MA) for blood pressure and heart rate (HR) measurement. Soon after the cannulation, 500 IU/kg of heparin (Upjohn) was injected to prevent intravascular blood clotting. The animals were allowed to stabilize for at least 30 min before recording and administration of any test substances. The test substances were injected through a cannula inserted into the jugular vein.
Effects of A. altilis extract on blood pressure and HR
The tracheal tube was cannulated with a polyethylene tube to facilitate spontaneous respiration. The systemic blood pressure was recorded from the right common carotid artery via an arterial cannula connected to a pressure transducer (P23XL) that was connected to a Grass polygraph (Model 7D, Grass Instrument, Quincy, MA). After the equilibration period, the dose-response relationship to A. altilis leaf extract were determined by intravenous injection of each dose (20.88–146.18 mg/kg) into the left jugular vein and flushed with 0.1 mL saline. Each dose was separated by 10 min before the injection of the next dose. The blood pressure was recorded at a chart speed of 10 mm/s and the HR was measured by increasing the chart speed on the machine to 50 mm/min. The mean arterial pressure (MAP) was calculated as the sum of the diastolic pressure and 1/3 pulse pressure.
Effect of A. altilis on atropine, propranolol, mepyramine and eNOS blockade
The effect of Artocarpus altilis aqueous extract was examined after administration of the muscarinic receptor antagonist atropine (2 mg/kg), the β-adrenoceptor antagonist, propranolol (1 mg/kg), mepyramine (5 mg/kg) or N(G)-nitro-l-arginine methyl ester (l-NAME, 5 mg/kg). Each drug was given intravenously and allowed to incubate for 5–10 min before a bolus injection of the A. altilis extract (41.76 mg/kg) and the corresponding blood pressure and HR changes were recorded.
Isolated organ bath studies
To study the effect of the aqueous extracts on vascular reactivity, thoracic aorta were isolated from the rats and placed in cold (4°C) physiological salt solution of the following composition (mmol/L): NaCl, 112; KCl 5; CaCl2 1.8; MgCl2 1, NaHCO3 25; KH2PO4 0.5; NaH2PO4 0.5; Glucose 10; pH 7.4. Each aorta was cleaned of connective tissues under the dissecting microscope and cut into segments (~3 mm long) and mounted in thermostated 10 mL organ baths (37°C) containing physiological salt solution gassed with 95% O2–5% CO2. The aortic rings were hooked to an isometric force transducer (SS12LA, Biopac Systems Inc, Goleta, CA), connected to a data acquisition unit (Biopac Student Lab MP36 systems) and isometric contraction was recorded using Biopac BSL PRO computer software. A passive tension of 1 g was applied to the tissue using a movable device. The rings were equilibrated for 90 min while being rinsed every 15 min. During the equilibration period, the rings were challenged with 1 µMol/L phenylephrine and the aorta was relaxed with 10 µMol/L acetylcholine to test the endothelial integrity.
After 90 min equilibration period, aortic rings were precontracted with 1 µM phenylephrine (PE) and when the contraction had reached the plateau, graded doses of A. altilis extract was added to the rings with or without endothelium. Endothelium was removed mechanically by gently rubbing the intimal surface of aortic rings with glass rod and removal was confirmed by the absence of relaxation to 10−7 M acetylcholine (CitationFurchgott & Zawadzki, 1980). To observe the effects of pre-incubation with the extracts, aortic rings with intact endothelium were pre-incubated with the A. altilis extract (0.71 mg/mL) for 30 min following which cumulative dose-response curves were generated for phenylephrine.
To further elucidate the mechanism of action, the calcium channel blocking effect was assessed by testing on high K+ (80 mM)-induced contraction. The aortic ring was allowed to stabilize in normal Kreb’s solution, which was then replaced with Ca2+-free Kreb’s solution containing EGTA (0.1 mM) for 30 min in order to remove calcium from the tissues. This solution was further replaced with K+-rich and Ca2+-free Kreb’s solution, with the following composition (mMol/L): KCl 50, NaCl 91.04, MgSO4 1.05, NaHCO3 11.90, glucose 5.55 and EGTA 0.1 mM. After an incubation period of 30 min, control dose-response curves of Ca2+ were obtained and then re-determined after pretreating the aortic rings for 20 min with the aqueous extract.
CYP inhibition assays
The test compounds were evaluated for their ability to inhibit the catalytic activity of human CYP enzymes by means of fluorometric detection assays conducted in 96-well microtitre plates using the substrate; 7-benzyloxy-4-trifluoromethyl-coumarin for detecting CYP3A4 activity and the substrate; 3-[2-(N,N-diethyl-N-methylamino)ethyl]-7-methoxy-4-methylcoumarin for detecting CYP2D6 activity, as described elsewhere (CitationCrespi et al. 1997; CitationBadal et al. 2011). The reactions were monitored fluorometrically at 37°C, using a Varian Cary Eclipse Fluorescence spectrophotometer. All standards were dissolved in 20% acetonitrile and less than 0.3% of acetonitrile was used in the final assay.
The accuracy of experimental techniques employed to detect CYPs3A4 and 2D6 inhibition assays were verified with known inhibitors ketoconozole and quinidine, respectively and the obtained IC50 values (0.06 ± 0.01 and 0.03 ± 0.01 µM) compared well with published values (0.06 and 0.01 µM) (Gerhauser et al., 2003). Michaelis constant, Km, was determined for the marker substrate under the specified experimental conditions, in order to determine suitable substrate concentrations for assessing inhibitory potential of the test extract. Control experiments included the intrinsic fluorescence of the A. altilis extract and its effect on the metabolite’s fluorescence at the respective excitation and emission wavelengths.
Statistical analysis
The results are presented as mean ± standard error of mean (SEM). The results were analyzed using GraphPad Prism software version 5 (GraphPad Software, San Diego, CA). Student’s t-test was used to compare the means. p Value of 0.05 was considered statistically significant. IC50 values were determined by fitting the data in Sigma Plot (version 10.0) and enzyme kinetics module, using non linear regression analysis. IC50 data listed represent the average values from three different determinations.
Results
Effect of graded doses of A. altilis on blood pressure and heart rates
Intravenous administration of A. altilis leaf extract caused a dose-dependent reduction in systolic blood pressure (SBP), diastolic blood pressure (DBP) and MAP. HR showed a similar reduction at increasing dose of the A. altilis extract ().
Table 1. Effect of various doses of Artocarpus altilis on blood pressure in rats.
Effect of A. altilis on atropine, propranolol, histamine and eNOS blockade
The effects of atropine, propranolol, mepyramine and l-NAME on the hypotensive action of the aqueous extract of A. altilis (41.76 mg/kg) were investigated. As shown in , the pre-treatment of anaesthetized Sprague–Dawley normotensive rats with atropine sulphate (2 mg/kg) or mepyramine (5 mg/kg) did not significantly affect the hypotensive effect of the plant extract, but the plant extract caused a significant (p < 0.05) further reduction of MAP. By contrast, pre-treatment with l-NAME and propranolol (1 mg/kg) significantly (p < 0.01) reduced the hypotensive effect of the extract in the rats.
Figure 1. The maximal immediate changes after extract injection in mean arterial pressure (MAP) in anaesthetized rats that received intravenous injection of A. altilis aqueous extract (41.76 mg/kg) before and after pre-treatment with atropine (2 mg/kg), propranolol, mepyramine (5 mg/kg), or l-NAME (5 mg/kg). Each point represents the mean ± SEM. n = 5. *p < 0.05 vs. the value without antagonisms
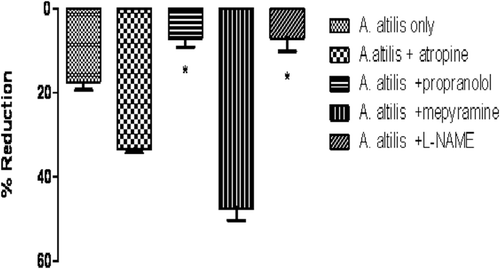
Effect of A. altilis on phenylephrine-induced contraction
The aqueous leaf extract of A. altilis (0.7–4.3 mg/mL) did not have any vasoconstrictor effect when incubated in aortic rings. However, the extract caused a significant (p < 0.05) reduction in phenylephrine-induced contraction of aortic rings with a maximum contraction of 75 ± 5% and a rightward shift of the dose-response curve (). The sensitivity (pD2) to phenylephrine in the presence of A. altilis was 5.73 which was significantly (p < 0.05) reduced when compared with the control (6.64).
Effect of A. altilis on relaxation of aorta
The extract of A. altilis (0.7–4.3 mg/mL) caused a dose-dependent relaxation of aortic rings precontracted with phenylephrine (). The maximum relaxation to phenylephrine-induced contraction was 59.9 ± 2% in aorta with intact endothelium. In endothelium-denuded aortic rings, the extract also caused vasorelaxation in a dose-dependent manner with a maximum relaxation of 45.9 ± 3%. However, relaxation of aortic rings with intact endothelium was significantly (p < 0.05) higher when compared with aortic rings without endothelium. Endothelium denudation resulted in partial blockade of the responses shifting the inhibitory dose-response curve of A. altilis to the right with pD2 value of 4.07 mg/mL compared with 3.22 mg/mL in aortic rings with intact endothelium. The sensitivity (pD2) to A. altilis in aortic rings with intact endothelium was 2.93 that was significantly (p < 0.05) reduced when compared with the sensitivity in endothelium-denuded aortic rings (2.38).
Effect of A. altilis aqueous extract on calcium induced contraction
In the presence of A. altilis, the calcium ion concentration-response curve constructed in a calcium ion free medium on rat aorta was shifted to the right (). The shift was followed by a suppression of the maximum effect. The extract reduced the maximum contraction to 65.4 ± 4% in the calcium ion induced contraction which was significantly (p < 0.05) lower than control.
Effect of A. altilis extract on CYP enzyme activities
The inhibitory impact of A. altilis extract on the activities of CYPs3A4 and 2D6 enzymes is displayed in , using heterologously expressed microsomes, and used to generate IC50 values that were calculated to be 0.695 ± 0.187 mg/mL and 0.512 ± 0.131 mg/mL, respectively.
Figure 5. Inhibition of CYPs 3A4 and 2D6 activities by A. altilis using heterologously expressed human enzymes. Human CYP3A4 catalyzed 7-benzyloxy-4-trifluoromethyl-coumarin (50 µM) activity and CYP2D6-catalyzed 3-[2-(N,N-diethyl-N-methylammonium)-ethyl]-7-methoxy-4-methylcoumarin (1.5 M) were determined in the presence of varying concentrations of the aqueous A. altilis extract (between 0 and 10 mg/mL) as described in ‘Materials and Methods’. Control enzyme activities (mean ± SEM) were 0.09 ± 0.01 µM/min/pmol of CYP3A4 and 0.10 ± 0.003 µM/min/pmol of CYP2D6, respectively. Data are expressed as mean percentage of control enzyme activity for three independent experiments.
![Figure 5. Inhibition of CYPs 3A4 and 2D6 activities by A. altilis using heterologously expressed human enzymes. Human CYP3A4 catalyzed 7-benzyloxy-4-trifluoromethyl-coumarin (50 µM) activity and CYP2D6-catalyzed 3-[2-(N,N-diethyl-N-methylammonium)-ethyl]-7-methoxy-4-methylcoumarin (1.5 M) were determined in the presence of varying concentrations of the aqueous A. altilis extract (between 0 and 10 mg/mL) as described in ‘Materials and Methods’. Control enzyme activities (mean ± SEM) were 0.09 ± 0.01 µM/min/pmol of CYP3A4 and 0.10 ± 0.003 µM/min/pmol of CYP2D6, respectively. Data are expressed as mean percentage of control enzyme activity for three independent experiments.](/cms/asset/23c2c530-75c4-4a45-a72b-24d858b3e9dd/iphb_a_658113_f0005_b.gif)
Discussion
The main finding from this study is that the aqueous extract of A. altilis produced a dose-dependent decrease in SBP, DBP, MAP and HR in normotensive rats. The present results suggest that the extract had a negative chronotropic effect on the heart. To determine the mechanisms involved in the acute effect of the extract, we pre-treated rats with different drugs. Our results reveal that neither the muscarinic receptor antagonist atropine nor the histaminergic receptor antagonist, mepyramine reduced the hypotensive effect of the plant extract, but the nitric oxide synthase inhibitor, l-NAME and β-blocker propranolol inhibited the hypotensive effect of the A. altilis extract. Since the hypotensive response induced by A. altilis was attenuated by l-NAME, we suggest that this effect could be due to a decrease of the total peripheral resistance through direct activation of endothelial NO. The mechanism of the hypotensive activity is probably through endothelium and adrenergic mechanisms. However, there is a possibility that the A. altilis extract might have a direct effect on the heart to cause a reduction in MAP. A previous study reported that the leaf extract has a negative inotropic effect on rat myocardium through a decoupling of excitation and contraction (CitationYoung et al., 1993).
Part of the inhibitory actions of the extract on the blood pressure could be explained by myocardial mechanisms as blood pressure is known to be influenced by changes in cardiac contractility when total peripheral vascular resistance remains fairly unchanged. It is likely that the negative chronotropic effect of aqueous A. altilis extract is due to other mechanisms such as direct effect on the heart and blood vessels. CitationJanssen et al. (2000) had reported that MAP and HRs are influenced by cholinergic function, although the blood vessels contain muscarinic receptors, M2 receptors in the heart mediate reduced HR but more profoundly affected by adrenergic β1 stimulation. The hypotensive action of A. altilis was unaffected in animals treated with atropine, an anticholinergic agent, indicating lack of cholinergic influence.
In order to determine the mechanism of action of the aqueous extract of this plant we performed experiments in vitro, using isolated thoracic aortic rings. Our results showed that A. altilis extract decreased the phenylephrine-induced contraction in aorta suggesting the involvement of calcium ion mechanism. Since the vascular endothelium is very important in modulating the contractility of vascular smooth muscle through the release of vasodilator and constrictor factors (CitationFurchgott & Zawadzki, 1980; CitationVanhoutte et al., 1986), the vasodilator effect of A. altilis was done in both endothelium-intact and denuded aorta. Our results showed that A. altilis extract decreased the phenylephrine-induced contraction in both endothelium intact and denuded rings. This suggested that the vasodilator effect of A. altilis is mediated via a combination of endothelium-dependent and independent pathways with the latter likely being via Ca2+ antagonism, as the compound was able to relax K+-induced contractions.
In order to confirm the involvement of Ca2+ in the observed hypotensive effect, the aortic ring contractility to K+ and Ca2+ was studied in Ca2+ free Kreb’s solution containing EGTA (0.1 mM). A. altilis extract inhibited Ca2+ induced contraction and high KCl-induced contractions. High K+ and PE-induced contractions are the result of an increased Ca2+ influx through voltage-stimulated type-L Ca2+ channels (CitationBolton, 1979; CitationKaraki & Weiss, 1984), and are specifically inhibited by Ca2+ antagonists (CitationGodfraind et al., 1986).The inhibitory effect of A. altilis on contractile response induced by Ca2+ could be attributed, at least partly, to a blockade of Ca2+ entry through voltage-stimulated Ca2+ channels.
The A. altilis extract is known to contain flavonoids, saponins and cardiac glycosides (CitationWang et al., 2006; CitationBoonphong et al., 2007; CitationShamaun et al., 2010). Flavonoids and saponins present in other plants have been reported to exhibit hypotensive and vasodilator activities (CitationGilani et al., 1994; CitationAjay et al., 2003; CitationHodgson & Croft, 2006). The presence of such compounds in A. altilis might possibly contribute to the hypotensive effects in the present study.
CYP3A4 is responsible for the metabolism of numerous hypertension therapeutics, including calcium channel blockers, such as diltiazem, felodipine, verapamil amlodipine, lercanidipine, nifedipine, nisoldipine, nitrendipine; HMG-CoA reductase inhibitors such as simvastatin, atorvastatin, cerivastatin, lovastatin and other important classes of drugs including antiretrovirals, immunosuppresants and antibiotics (CitationCheng, 2000). CYP2D6 also metabolizes important cardiovascular therapeutics such as β blockers S-metoprolol, timolol, carvedilol and propafenone. Potent inhibition of these enzymes could result in clinically relevant drug adversities. The inhibitory properties of A. altilis on CYP activity have not been reported previously and this study is particularly useful, given the concomitant use of breadfruit with hypertensives such as simvastatin, nefidipine and captopril (CitationPicking et al., 2011), which are known CYP3A4 and CYP2D6 substrates. The aqueous extract of A. altilis inhibited CYPs3A4 and 2D6 enzyme activities in the sub mg/mL levels, which appear to be poor in potency. Other medicinal plant extracts have been noted to have IC50 values ranging between 0.1 mg/mL (CitationShields et al., 2009) and more potent values of less than 0.01 mg/mL (CitationTam et al., 2009; CitationAgbonon et al., 2010). Although conclusive determinations can only be drawn from clinical studies, the weak inhibition displayed by the A. altilis extract in this in vitro investigation is indicative of a fairly low likelihood of clinically observable adversities through CYPs3A4 and 2D6 mediated metabolism.
Conclusion
In conclusion, the results obtained from this study show that the aqueous leaf extract of A. altilis induced hypotension and bradycardia in normotensive rats that could be due to direct cardiac adrenegic activation, activation of vascular endothelium and spasmolytic effect on vascular smooth muscle via Ca2+ channel antagonism. Our data supports the use of this plant extract as a traditional medicine against hypertension as the aqueous leaf extract of A. altilis displayed poor in vitro inhibition on CYPs3A4 and 2D6 enzyme activities making it unlikely to impart clinically significant pharmacokinetic drug interactions via the inhibition of these enzymes.
Declaration of interest
This research was funded by the University of West Indies new initiative grant No: 15053P to Dr. C. R. Nwokocha and by the Forest Conservation Fund and International Foundation for Science.
References
- Agbonon A, Eklu-Gadegbeku K, Aklikokou K, Gbeassor M, Akpagana K, Tam TW, Arnason JT, Foster BC. (2010). In vitro inhibitory effect of West African medicinal and food plants on human cytochrome P450 3A subfamily. J Ethnopharmacol, 128, 390–394.
- Adewole SO, Ojewole JO. (2007). Hyperglycaemic effect of Artocarpus communis Forst (Moraceae) root bark aqueous extract in Wistar rats. Cardiovasc J Afr, 18, 221–227.
- Ajay M, Gilani AU, Mustafa MR. (2003). Effects of flavonoids on vascular smooth muscle of the isolated rat thoracic aorta. Life Sci, 74, 603–612.
- Badal S, Williams SA, Huang G, Francis S, Vedantam P, Vendantam P, Dunbar O, Jacobs H, Tzeng TJ, Gangemi J, Delgoda R. (2011). Cytochrome P450 1 enzyme inhibition and anticancer potential of chromene amides from Amyris plumieri. Fitoterapia, 82, 230–236.
- Bolton TB. (1979). Cholinergic mechanisms in smooth muscle. Br Med Bull, 35, 275–283.
- Boonphong S, Baramee A, Kittakoop P, Puangsombat P. (2007). Antitubercular and antiplasmodial prenylated flavones from the roots of Artocarpus altilis. Chiang Mai J Sci, 34, 339–344.
- Cheng JW. (2000). Cytochrome p450-mediated cardiovascular drug interactions. Heart Dis, 2, 254–258.
- Clarke SE, Jones BC. (2008). Human cytochromes P450 and their role in metabolism based drug-drug interactions. In: Rodrigues AD. (ed). Drug Drug Interactions (2nd Edn). New York: Informa Health Care. pp 53–86.
- Crespi CL, Miller VP, Penman BW. (1997). Microtiter plate assays for inhibition of human, drug-metabolizing cytochromes P450. Anal Biochem, 248, 188–190.
- Delgoda R, Westlake AC. (2004). Herbal interactions involving cytochrome p450 enzymes: A mini review. Toxicol Rev, 23, 239–249.
- Deivanai S, Bhore SJ. (2010). Breadfruit (Artocarpus altilis Fosb.) – An underutilized and neglected fruit plant species. Middle-East J Sci Res, 6, 418–428.
- Delgoda R, Younger N, Barrett C, Braithwaite J, Davis D. (2010). The prevalence of herbs use in conjunction with conventional medicines in Jamaica. Complement Ther Med, 18, 13–20.
- Furchgott RF, Zawadzki JV. (1980). The obligatory role of endothelial cells in the relaxation of arterial smooth muscle by acetylcholine. Nature, 288, 373–376.
- Gardner JM, Grant D, Hutchinson S, Wilks R. (2000). The use of herbal teas and remedies in Jamaica. West Indian Med J, 49, 331–335.
- Gerhäuser C, Klimo K, Heiss E, Neumann I, Gamal-Eldeen A, Knauft J, Liu GY, Sitthimonchai S, Frank N. (2003). Mechanism-based in vitro screening of potential cancer chemopreventive agents. Mutat Res, 523–524, 163–172.
- Gilani AH, Shaheen F, Saeed SA. (1994). Cardiovascular actions of Daucus carota. Arch Pharm Res, 17, 150–153.
- Godfraind T, Miller R, Wibo M. (1986). Calcium antagonism and calcium entry blockade. Pharmacol Rev, 38, 321–416.
- Hodgson JM, Croft KD. (2006). Dietary flavonoids: Effects on endothelial function and blood pressures. J Sci Food Agric, 86, 2492–2498.
- Jagtap UB, Bapat VA. (2010). Artocarpus: A review of its traditional uses, phytochemistry and pharmacology. J Ethnopharmacol, 129, 142–166.
- Janssen BJ, Leenders PJ, Smits JF. (2000). Short-term and long-term blood pressure and heart rate variability in the mouse. Am J Physiol Regul Integr Comp Physiol, 278, R215–R225.
- Karaki H, Weiss GB. (1984). Calcium channels in smooth muscle. Gastroenterology, 87, 960–970.
- Kristoffersen AE, Fønnebø V, Norheim AJ. (2008). Use of complementary and alternative medicine among patients: Classification criteria determine level of use. J Altern Complement Med, 14, 911–919.
- Mitchell SA, Ahmad MH. (2006). A review of medicinal plant research at the University of the West Indies, Jamaica, 1948-2001. West Indian Med J, 55, 243–269.
- Nwokocha CR, Owu DU, Ufearo CS, Iwuala MO. (2011). Comparative study on the efficacy of Garcinia kola in reducing some heavy metal accumulation in liver of Wistar rats. J Ethnopharmacol, 135, 488–491.
- Nwokocha, CR, Owu DU, Ufearo CS, Iwuala MOE. (2012). Comparative study on the efficacy of Allium sativum (garlic) in reducing some heavy metal accumulation in liver of Wistar rats. Food Chem Toxicol, 50, 222–226.
- Picking D, Younger N, Mitchell S, Delgoda R. (2011). The prevalence of herbal medicine home use and concomitant use with pharmaceutical medicines in Jamaica. J Ethnopharmacol, 137, 305–311.
- Ragone D. (2001). Chromosome numbers and pollen stainability of three species of Pacific Island breadfruit (Artocarpus, Moraceae). Am J Bot, 88, 693–696.
- Shamaun SS, Rahmani M, Hashim NM, Ismail HB, Sukari MA, Lian GE, Go R. (2010). Prenylated flavones from Artocarpus altilis. J Nat Med, 64, 478–481.
- Shields M, Niazi U, Badal S, Yee T, Sutcliffe MJ, Delgoda R. (2009). Inhibition of CYP1A1 by quassinoids found in Picrasma excelsa. Planta Med, 75, 137–141.
- Tam TW, Liu R, Arnason JT, Krantis A, Staines WA, Haddad PS, Foster BC. (2009). Actions of ethnobotanically selected Cree anti-diabetic plants on human cytochrome P450 isoforms and flavin-containing monooxygenase 3. J Ethnopharmacol, 126, 119–126.
- Vanhoutte PM, Rubanyi GM, Miller VM, Houston DS. (1986). Modulation of vascular smooth muscle contraction by the endothelium. Annu Rev Physiol, 48, 307–320.
- Wang Y, Xu K, Lin L, Pan Y, Zheng X. (2007). Geranyl flavonoids from the leaves of Artocarpus altilis. Phytochemistry, 68, 1300–1306.
- Wang Y, Deng T, Lin L, Pan Y, Zheng X. (2006). Bioassay-guided isolation of antiatherosclerotic phytochemicals from Artocarpus altilis. Phytother Res, 20, 1052–1055.
- Weng JR, Chan SC, Lu YH, Lin HC, Ko HH, Lin CN. (2006). Antiplatelet prenylflavonoids from Artocarpus communis. Phytochemistry, 67, 824–829.
- Whistler WAJ. (1985). Traditional and herbal medicine in the Cook Islands. J Ethnopharmacol, 13, 239–280.
- Young RE, Williams LAD, Gardner MT, Fletcher CK. (1993). An extract of the leaves of the breadfruit Artocarpus altilis (Parkinson) Fosberg exerts a negative inotropic effect on rat myocardium. Phytother Res, 7, 190–193.