Abstract
Context: A new caffeic acid derivative, named (7′Z)-3-O-(3, 4-dihydroxyphenylethenyl)-caffeic acid (CADP), extracted from Abacopteris penangiana (Hook.) Ching.
Objective: To elucidate the neuroprotective effect of CADP against H2O2-induced cytotoxicity in PC12 cells and d-galactose (d-gal)-induced neurotoxicity in mice brain.
Materials and methods: CADP was isolated from the methanol extract of the rhizomes of A. penangiana. In vitro, the protective effect of CADP (0.1–10 μM) against H2O2-induced oxidative damage on PC12 cells was investigated by a MTT assay. In vivo, behavioral tests and antioxidant enzymes measurements were performed to investigate the protective effect of intraperitoneal (i.p.) injection of CADP (5 or 10 mg/kg/day) for 2 weeks on d-gal-induced neurotoxicity in mice.
Results: The results showed that CADP significantly attenuated cell toxicity in a dose-dependent manner, and the EC50 value of CADP was 0.83 ± 0.02 μM. In vivo, it was found that CADP significantly improved the behavioral performance of d-gal-treated mice in both Morris water maze (MWM) test and step-down avoidance test. As compared with model group, CADP (5, 10 mg/kg/day) attenuated the decrease in superoxide dismutase (SOD) activities by 40.5 and 75.4%, respectively; attenuated the decrease in glutathione peroxidase (GPx) activities by 53.8 and 73.2%, respectively; attenuated the decrease in catalase activities by 12.0 and 53.3%, respectively; reduced the increased levels of malondialdehyde (MDA) by 38.6 and 79.9%, respectively.
Discussion and conclusion: The results suggested that CADP has significant neuroprotective effects which can be attributed to inhibiting the generation of free radical and enhancing the activity of endogenous antioxidant enzymes.
Introduction
(7′Z)-3-O-(3, 4-Dihydroxyphenylethenyl)-caffeic acid (CADP; ) is a new caffeic acid derivative extracted from Abacopteris penangiana (Hook.) Ching that has been widely used in Chinese folk medicine to treat inflammation and upper respiratory tract infection (CitationZhao et al., 2010). Recently studies showed that caffeic acid and its derivatives have potential antioxidation effect, suppressed cerebral lipid peroxidation, and reduced brain infarct after cerebral ischemia in rats (CitationIrmak et al., 2003; CitationTsai et al., 2006). It is suggested that caffeic acid derivatives have therapeutic potential in the treatment of neurodegenerative diseases.
Figure 1 . Structure of the caffeic acid derivative (7′Z)-3-O-(3, 4-dihydroxyphenylethenyl)-caffeic acid (CADP).
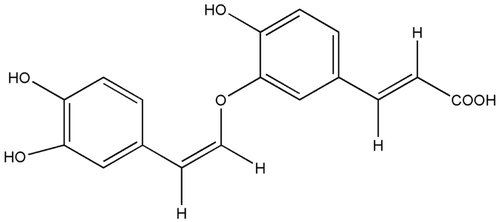
It is well known that formation of reactive oxygen species (ROS) has been proposed to be an important step leading to neuronal death in a variety of age-related neurodegenerative disorders such as Alzheimer’s disease and Parkinson’s disease (CitationCastegna et al., 2002). ROS can cause great damage to the structure and the function of the cell membranes and even lead to the oxidative damage of the tissues and organs. Furthermore, the hippocampus is considered as the most vulnerable area to oxidative stress in the brain. The damaged neuronal cells in hippocampus will finally trigger neurodegenerative disorders.
The PC12 cell line is derived from a tumor in the rat adrenal gland and is the most widely used neuronal cell line for studying mechanisms associated with neurodegenerative disorders (CitationMeng et al., 2007). Moreover, PC12 cells have many advantages over primary cultured neuronal cells, such as the cell population has good homology and can be obtained easily. When the cells treated with H2O2, the oxidative damage would cause cell death and depreciation. However, these effects can be blocked by the addition of antioxidants.
Rodent chronically injected with d-galactose (d-gal) has been used as an animal model for brain aging or neuroprotective pharmacology research (CitationWei et al., 2005; CitationZhang et al., 2005). Although d-gal is a normal reducing sugar in the body at the normal level, at the high level it can form advanced glycation endproducts that are not metabolized further which accumulate in neurons and cause oxidative stress. Recent studies showed that d-gal-induced aging-related changes included increased production of free radicals and decreased antioxidant enzyme activities (CitationLei et al., 2011).
In our previous research (CitationZhao, 2006), it was found that CADP showed a marked antioxidative effect against ABTS and DPPH. In order to further validate the neuroprotective effect of CADP via antioxidant, we investigated the protective effect of CADP against H2O2-induced cytotoxicity in PC12 cells and d-gal-induced neurotoxicity in mice brain.
Materials and methods
Materials
Rhizomes of A. penangiana were collected from Wufeng City, Hubei Province, PR China, in August 2009 and authenticated by Prof Jinlan Ruan, College of Pharmacy, Tongji Medical Center, Huazhong University of Science and Technology. A specimen (NO. PZ0609) has been deposited in College of Pharmacy, Tongji Medical Center, Huazhong University of Science and Technology.
Silica gel (200–300 mesh) was obtained from Qingdao Marine Chemical Co. Ltd., Qingdao, China. Sephadex LH-20 was obtained from Amersham Pharmacia Biotech Co. Ltd., Uppsala, Sweden. RP-18 silica gel (40–63 μm) was purchased from Merck, Darmstadt, Germany. d-gal was purchased from Sigma-Aldrich (St Louis, MO, USA), and dissolved in physiological saline. Commercial kits used for the determination of malondialdehyde (MDA), superoxide dismutase (SOD), glutathione peroxidase (GPx) and catalase were purchased from Jiancheng Institute of Biotechnology (Nanjing, Jiangsu Province, PR China).
Preparation of CADP
The air-dried rhizomes of A. penangiana (5.0 kg) were extracted three times at room temperature with MeOH, and the extract (380 g) was suspended in water and partitioned with CHCl3, EtOAc, and n-BuOH, respectively. The EtOAc extract (50 g) was chromatographed on silica gel (200–300 mesh) column and eluted with CHCl3-MeOH (from 50:1 to 1:1) to provide five subfractions, B1–B5. Subfraction B2 (3.46 g) was subjected to Sephadex LH-20 eluted with CHCl3-MeOH (2:3), and then subfraction B2b (1.12 g) was chromatographed on reversed C18 column eluted with MeOH-H2O (from 2:3 to 3:2) to afford CADP (0.16 g). The purity of the compound was more than 98% by high-performance liquid chromatography (HPLC) analysis.
Cell culture and treatment
PC12 cells were grown in Dulbecco’s modified Eagle’s medium (DMEM) supplemented with 10% fetal bovine serum, 5% heat-inactivated horse serum, 100 U/ml penicillin, and 100 μg/ml streptomycin at 37°C in an incubator aerated with 95% air and 5% CO2. Concentrations of 200 μM H2O2 and vehicle as control, CADP was dissolved and diluted with serum-free medium.
Viability assay
PC12 cells were plated at the density of 1 × 104 cells/well in 96-well plates and measured by MTT assay (CitationMosmann, 1983). Briefly, the CADP (0.1, 0.5, 1, 5, and 10 μM) was preincubated for 24 h before 200 μM H2O2 was added. After 4-h exposure to H2O2, MTT solution in phosphate-buffered saline (PBS) was added to obtain a final concentration of 0.5 mg/ml, and the incubation continued for 4 h. Finally, MTT was removed and the colored formazan was dissolved in dimethyl sulfoxide. The absorption values were measured at 570 nm using a Digiscan Microplate Reader (Assys Hitech Kornenburg, Austria). The viability of PC12 cells in each well was presented as the percentage of control cells.
Animals and administration
Kunming mice, weighing 18–22 g, were obtained from Experimental Animal Center, Tongji Medical Center, Huazhong University of Science and Technology, China. The animals were adapted to the feeding condition for one week, with controlled temperature (22 ± 2°C) and humidity (60%). They were fed with standard diet and water ad libitum. After acclimatization to the laboratory conditions, the mice were randomly divided into four groups (14 in each group): vehicle control group, d-gal model group, low and high dose of CADP treatment groups. The mice of d-gal model group and CADP treatment groups were daily subcutaneously injected with d-gal at the dose of 150 mg/kg once daily for 7 weeks while those of control group were treated with same volume of physiological saline. From the sixth week, CADP treatment groups mice were daily intraperitoneal (i.p.) injected with CADP at the dose of 5 or 10 mg/kg after the injection of d-gal. Vehicle control group and d-gal model group mice were administered with same volume of physiological saline. At the end of the treatment period, behavioral tests and biochemical measurements were performed as mentioned below. All experiments were performed in compliance with the Chinese legislation on the use and care of laboratory animals and were approved by the Huazhong University of Science and Technology Committee on Animal Care and Use.
Morris water maze (MWM) test
The MWM test was performed as previously described (CitationMorris, 1984). The experimental apparatus consisted of a circular water tank, containing water (22 ± 1°C) to a depth of 30 cm, which was rendered opaque by adding black nontoxic carbon ink. The tank was divided into four equal quadrants; an escape platform was hidden 1 cm below the surface of the water and placed at the midpoint of one quadrant. During the test trial, the mouse was placed in the water facing the wall at one of three randomized starting positions (in three different quadrants that did not contain the platform). It was given 90 s to find the platform and was allowed to rest on it for 10 s. Animals which failed to find the location within the given time were gently guided to the platform and were allowed to stay on it for 10 s. Each mouse was given four trials daily for five consecutive days, with an inter-trial interval of about 60 s. The escape latency to locate the platform, and the swimming distance were recorded. To determine whether the animal would take a spatial learning strategy to locate the platform, a single-spatial probe trial was assessed on day 6. The platform was removed from the water tank, and the mice were allowed to swim freely for 60 s. The time spent in the target quadrant, which had previously contained the hidden platform, was recorded. The numbers of crossing the non-exits were recorded for each trial. All data were recorded and analyzed by a computerized video imaging analysis system (EthoVision; Noldus Information Technology BV, Wageningen, the Netherlands).
Step-down avoidance test
The step-down avoidance test was performed as previously described (CitationRubio et al., 2007). The test was carried out in an instrument, which consisted of a plastic box divided into five equal cabins with grid floor made of stainless steel bar spaced 1 cm apart. The rubber platform (4.5 cm in diameter and 4.5 cm in height) was placed at a corner on the bottom of the cabins. Before normal test, mice were trained to stand on the safe platform. When it stepped down with all the four paws onto the floor, it would receive a foot shock (36 V, 0.3 mA, 2 s,) and it was normal reaction to escape and be back onto the platform. At the beginning of training, the mice were placed in the box to adapt for 5 min. Next, electric currents were delivered and the mice would jump onto the platform to avoid the electric stroke, and the electric currents were maintained for 3 min. After a 24-h interval, the mice were again placed on the platform, step-down latency (duration of staying on the platform) in seconds and the number of errors (the mouse steps down from the platform and gets a shock) within 3 min were recorded as learning performances.
Biochemical analysis
For biochemical studies, animals were deeply anesthetized by chloral hydrate and sacrificed by decapitation after behavioral tests. Brains were removed and dissected on ice to obtain the hippocampi. Before detection, the hippocampus was homogenized in cold saline. The homogenate (10%) was centrifuged at 4000g at 4°C for 10 min, and the supernatant were used for assay. Protein concentration was determined by the Coomassie blue protein-binding (CitationBradford, 1976) using bovine serum albumin as reference. The oxidant–antioxidant status of the hippocampus was determined by measuring the levels of lipid peroxidation, SOD activity, GPx activity, and catalase activity. Lipid peroxidation was determined by measuring levels of MDA, a byproduct of lipid peroxidation (CitationOhkawa et al., 1979).
Statistical analysis
Results were presented as mean ± SEM. Group differences in the escape latency and the swimming distance in the MWM training task were analyzed with two-way analysis of variance (ANOVA) with repeated measures, the factors being treatment and training day. The other data were analyzed using one-way ANOVA followed by the Student–Newman–Keuls test. p < 0.05 was accepted as statistically significant.
Results
Effect of CADP against H2O2-induced cytotoxicity in PC12 cells
H2O2-induced cytotoxicity in PC12 cells was assessed by measuring reduced MTT activity. PC12 viability was reduced when treated with H2O2. The results in show that the survival rate of PC12 cells was about 49.5 ± 7.6% when the cells were treated with 200 μM of H2O2 for 4 h. The treatment with 0.1, 0.5, 1, 5, and 10 μM of CADP increased the viability of PC12 cells significantly to 62.4 ± 9.6, 66.2 ± 7.5, 79.1 ± 10.7, 83.2 ± 10.3, and 87.1 ± 8.1%, respectively. The EC50 value for CADP was found to be 0.83 ± 0.02 μM.
Figure 2 . Protective effect of (7′Z)-3-O-(3, 4-dihydroxyphenyl-ethenyl)-caffeic acid (CADP) against H2O2-induced PC12 cell injury. Cells were incubated with 200 μM H2O2 for 4 h for MTT assay. CADP was added to the culture 24 h before H2O2 addition. The data are presented as means ± SEM (n = 6). #p < 0.05 and ##p < 0.01 compared with H2O2 group.
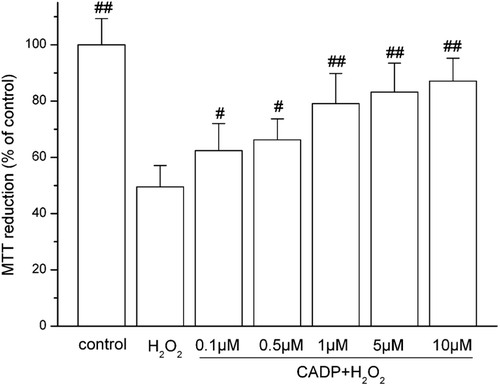
MWM test
MWM test was used to assess the effects of CADP on impairment of spatial learning and memory. The performances of all treated groups were improved with training. shows the mean latency of the animal on training days [F(4, 164) = 31.770, p < 0.001] and between treatments [F(3, 164) = 44.309, p < 0.001], but no interaction between the factors day and treatment [F(12, 164) = 0.561, p > 0.05]. Compared with the vehicle control group, animals in the d-gal model group took longer to find the hidden platform on all training days (p < 0.001). This result revealed that the d-gal model mice had significant cognitive impairment. Meanwhile, the prolonged escape latency in the d-gal model group was significantly reduced by long-term administration of CADP at 5 or 10 mg/kg. shows the swimming distance shortened progressively in five training days closely paralleled to the difference in escape latency: day, F(4, 164) = 54.749, p < 0.01; treatment, F(3, 164) = 46.450, p < 0.01; day by treatment interaction, F(12, 164) = 1.780, p > 0.05. The d-gal model group had significantly longer swimming distance than the vehicle control group (p < 0.01) and the CADP groups substantially shortened the swimming distance.
Figure 3 . Effects of (7′Z)-3-O-(3, 4-dihydroxyphenylethenyl)-caffeic acid (CADP) on the spatial learning and memory of mice in the Morris water maze test. (A) Mean latency in the hidden platform test during five consecutive days training. (B) Swimming distance moved in the hidden platform test during five consecutive days training. (C) The number of crossings over the exact location of the former platform. (D) Time spent in the target quadrant. All values are expressed as means ± SEM (n = 6–7). #p < 0.05 and ##p < 0.01 compared with the d-galactose (d-gal) model group.
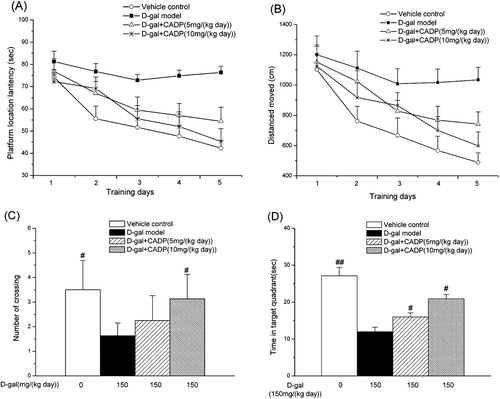
In the probe trial, the d-gal model group made fewer platform crossings than the vehicle control group (p < 0.05) and the CADP treatments increased the number of times of crossing over the platform site (). Furthermore, the d-gal model group had a decreased spatial preference for the target quadrant compared with the vehicle group. On the other hand, mice in both vehicle control and CADP groups mice spent most of their time in the target quadrant (). Taken together, these results indicated that CADP improved spatial learning and memory in d-gal-treated mice.
Step-down avoidance test
Step-down avoidance test is a behavioral task to evaluate the memory ability. As results showed (), the d-gal model mice exhibited reduced latency (p < 0.01) and increased number of errors (p < 0.01) than the vehicle control mice. CADP high dose treatment significantly prolonged the latency (p < 0.05 vs. the d-gal model) and decreased the number of errors (p < 0.01 vs. the d-gal model). However, CADP low dose treatment displayed no significant effects on latency (p > 0.05 vs. the d-gal model). Taken together, these data indicated that CADP improved the ability of spatial learning and memory in d-gal model mice.
Effects of CADP on MDA content and SOD, GPx, and catalase activities in hippocampus of senescent mice induced by d-gal
shows the effects of CADP on MDA levels and SOD, GPx, catalase activities in hippocampus. MDA levels in hippocampus of d-gal model group increased by 45.8% compared with vehicle control group. This increase was attenuated by treatment of both CADP at a dose of 5 mg/kg/day (p < 0.05 vs. d-gal model) and 10 mg/kg/day (p < 0.001 vs. d-gal model).
Table 1. Effect of CADP on MDA levels and activities of SOD, Gpx, and catalase in mouse brain.
As compared with vehicle control group, the SOD activity in hippocampus decreased significantly, by 23.7% in the d-gal model group. This decrease was attenuated by treatment of CADP at a dose of 10 mg/kg/day (p < 0.01 vs. the d-gal model), but CADP at the dose of 5 mg/kg/day did not show an obvious effect (p > 0.05 vs. the d-gal model).
Gpx decreased remarkably by 29.0% in hippocampus of d-gal model group mice as compared to the vehicle control group (p < 0.01). CADP treatment (5 mg/kg/day) treatment significantly increased the GPx level (p < 0.01 vs. d-gal model) in the hippocampus of d-gal-treated mice. Furthermore, as compared with the CADP low dose group, CADP at a dose of 10 mg/kg/day treatment displayed more pronounced effects on increasing the GPx level (p < 0.05).
The catalase activity in hippocampus declined by 46.2% in the d-gal model group as compared with the vehicle control group, CADP (5 or 10 mg/kg) could increase the activities of catalase to 62.7 or 78.4% of the vehicle control group, respectively.
Discussion
The increase in life expectancy in the 21st century has resulted in an increase in the prevalence of neurodegenerative diseases such as depression, Alzheimer’s disease, Parkinson’s disease and other dementias (CitationFernández-Segura et al., 1999). Neurodegenerative disease is a complex multifactorial process in which ROS plays a key role. ROS in response to the apoptotic process include activation of caspases, activation of nucleases and inactivation of nuclear repair polymerases, leading to the degradation of chromosomal DNA and cell death (CitationEnari et al., 1998). In an effort to better understand oxidative stress-related mechanisms, many studies have investigated the effects of natural products as antioxidants in vitro and in vivo (CitationPiga et al., 2007; CitationHalliwell, 2008). The present study reports the protective effect of CADP against H2O2-induced oxidative damage in PC12 cells and d-gal-induced neurotoxicity in mice.
Firstly, in vitro neuroprotective activity of CADP against H2O2-induced cell injury in PC12 cells was investigated with the MTT assay. H2O2 has been previously shown to induce apoptosis in PC12 cells, and antioxidant treatments protect the cells against H2O2-induced cell injury (CitationFujita et al., 2006). Thus, antioxidant strategies may provide useful intervention strategies to reduce oxidative stress-induced cell injury. In this study, PC12 cells exposed to H2O2 displayed a significant reduction in cell viability; pretreatment with CADP exhibited potent protection against H2O2-induced cytotoxicity.
Observing the distinct protective of CADP against H2O2-induced oxidative damage in PC12 cells, the study was further extended to in vivo conditions using d-gal induced neurotoxicity in mice. Previous studies demonstrated that d-gal-treated mice showed age-related deficits with various behavioral tests (CitationIda et al., 2004; CitationTian et al., 2005). In this study, the effects of CADP on the d-gal-induced neurotoxicity were investigated by the behavioral tests. Significant differences between the vehicle control mice and the d-gal model mice were observed in both MWM test and step-down avoidance test, suggesting that the injection of d-gal caused motor abnormalities and impairments of novelty-induced exploratory behaviors. The data also indicated that CADP could ameliorate the cognitive impairment induced by d-gal.
Finally, in order to investigate the neuroprotective mechanism of CADP in d-gal-treated mice, we used biochemical assays to investigate the antioxidant activity in the hippocampus, one part of the mammalian brain believe to be involved most directly in the process of learning and memory. The human body has a complex antioxidant defense system which contains the antioxidant enzymes SOD, GPx, and catalase. Any factors which can damage the activities of antioxidant enzymes may lead to accumulation of ROS and subsequently bring about oxidative damage (CitationHalliwell & Gutteridge, 1989). Meanwhile, ROS production can be indirectly estimated by measuring MDA, a product of lipid peroxidation (CitationYagi & Rastogi, 1979). In present study, lowered SOD, GPx, catalase, and elevated MDA content in the hippocampus of the d-gal model mice were observed as compared with the vehicle control mice. CADP significantly increased the activities of all these antioxidative enzymes and decreased the level of MDA in the hippocampus of d-gal-treated mice.
Conclusion
In conclusion, the present study revealed that CADP exhibited a potent protective effect against H2O2-induced cytotoxicity in PC12 cells and d-gal-induced neurotoxicity in mouse brain; its neuroprotective function may be mediated through its antioxidant activities. Therefore, CADP has the potential of being used as an effective neuroprotective agent against oxidative stress-induced neurotoxicity.
Declaration of interest
This work is supported by the following grants: (1) National Science Foundation of China (30973864); (2) Natural Science Foundation of Hubei Province of China (2009CDA067).
References
- Bradford MM. (1976). A rapid and sensitive method for the quantitation of microgram quantities of protein utilizing the principle of protein-dye binding. Anal Biochem, 72, 248–254.
- Castegna A, Aksenov M, Aksenova M, Thongboonkerd V, Klein JB, Pierce WM, Booze R, Markesbery WR, Butterfield DA. (2002). Proteomic identification of oxidatively modified proteins in Alzheimer’s disease brain. Part I: Creatine kinase BB, glutamine synthase, and ubiquitin carboxy-terminal hydrolase L-1. Free Radic Biol Med, 33, 562–571.
- Enari M, Sakahira H, Yokoyama H, Okawa K, Iwamatsu A, Nagata S. (1998). A caspase-activated DNase that degrades DNA during apoptosis, and its inhibitor ICAD. Nature, 391, 43–50.
- Fernández-Segura E, Cañizares FJ, Cubero MA, Warley A, Campos A. (1999). Changes in elemental content during apoptotic cell death studied by electron probe X-ray microanalysis. Exp Cell Res, 253, 454–462.
- Fujita Y, Izawa Y, Ali N, Kanematsu Y, Tsuchiya K, Hamano S, Tamaki T, Yoshizumi M. (2006). Pramipexole protects against H2O2-induced PC12 cell death. Naunyn Schmiedebergs Arch Pharmacol, 372, 257–266.
- Halliwell B. (2008). Are polyphenols antioxidants or pro-oxidants? What do we learn from cell culture and in vivo studies? Arch Biochem Biophys, 476, 107–112.
- Halliwell B, Gutteridge JMC. (1989). Free radicals in biology and medicine. 2nd ed. Oxford: Clarendron Press.
- Ida H, Ishibashi K, Reiser K, Hjelmeland LM, Handa JT. (2004). Ultrastructural aging of the RPE-Bruch’s membrane-choriocapillaris complex in the d-galactose-treated mouse. Invest Ophthalmol Vis Sci, 45, 2348–2354.
- Irmak MK, Fadillioglu E, Sogut S, Erdogan H, Gulec M, Ozer M, Yagmurca M, Gozukara ME. (2003). Effects of caffeic acid phenethyl ester and alpha-tocopherol on reperfusion injury in rat brain. Cell Biochem Funct, 21, 283–289.
- Lei Y, Fu W, Chen J, Xiong C, Wu G, Wei H, Ruan J. (2011). Neuroprotective effects of abacopterin E from Abacopteris penangiana against oxidative stress-induced neurotoxicity. J Ethnopharmacol, 134, 275–280.
- Meng H, Li C, Feng L, Cheng B, Wu F, Wang X, Li Z, Liu S. (2007). Effects of ginkgolide B on 6-OHDA-induced apoptosis and calcium over load in cultured PC12. Int J Dev Neurosci, 25, 509–514.
- Morris R. (1984). Developments of a water-maze procedure for studying spatial learning in the rat. J Neurosci Methods, 11, 47–60.
- Mosmann T. (1983). Rapid colorimetric assay for cellular growth and survival: Application to proliferation and cytotoxicity assays. J Immunol Methods, 65, 55–63.
- Ohkawa H, Ohishi N, Yagi K. (1979). Assay for lipid peroxides in animal tissues by thiobarbituric acid reaction. Anal Biochem, 95, 351–358.
- Piga R, Saito Y, Yoshida Y, Niki E. (2007). Cytotoxic effects of various stressors on PC12 cells: Involvement of oxidative stress and effect of antioxidants. Neurotoxicology, 28, 67–75.
- Rubio J, Dang H, Gong M, Liu X, Chen SL, Gonzales GF. (2007). Aqueous and hydroalcoholic extracts of Black Maca (Lepidium meyenii) improve scopolamine-induced memory impairment in mice. Food Chem Toxicol, 45, 1882–1890.
- Tian J, Ishibashi K, Ishibashi K, Reiser K, Grebe R, Biswal S, Gehlbach P, Handa JT. (2005). Advanced glycation endproduct-induced aging of the retinal pigment epithelium and choroid: A comprehensive transcriptional response. Proc Natl Acad Sci USA, 102, 11846–11851.
- Tsai SK, Lin MJ, Liao PH, Yang CY, Lin SM, Liu SM, Lin RH, Chih CL, Huang SS. (2006). Caffeic acid phenethyl ester ameliorates cerebral infarction in rats subjected to focal cerebral ischemia. Life Sci, 78, 2758–2762.
- Wei H, Li L, Song Q, Ai H, Chu J, Li W. (2005). Behavioural study of the D-galactose induced aging model in C57BL/6J mice. Behav Brain Res, 157, 245–251.
- Yagi K, Rastogi R. (1979). Assay for lipid peroxides in animal tissue by thiobarbituric acid reaction. Anal Biochem, 95, 351–358.
- Zhang Q, Li X, Cui X, Zuo P. (2005). d-Galactose injured neurogenesis in the hippocampus of adult mice. Neurol Res, 27, 552–556.
- Zhao ZX. (2006). Study on the chemical constituents and bioactivities of Abacopteris penangiana and Smilax china. Thesis of Ph.D. Degree, Huazhong University of Science and Technology, 163–167.
- Zhao ZX, Ruan JL, Jin J, Cai YL, Zhu CC, Yu Y. (2010). A new phenolic acid from rhizomes of Abacopteris penangiana. Chin Herb Med, 2, 165–167.