Abstract
Context: In the course of searching hepatoprotective agents from natural sources, the protective effect of chemical constituents of the marine brown alga Spatoglossum variabile Figaro et DE Notar (Dictyoaceae) against CCl4-induced liver damage in Wistar rats was investigated. The compounds were first investigated for in vitro radical scavenging potential and were also tested for β-glucuronidase inhibition to further explore the relationship between hepatoprotection and antiradical potential.
Methods: The compounds cinnamic acid esters 1 and 2 and aurone derivatives 3 and 4 were first investigated for in vitro radical scavenging potential against 1,1-diphenyl-2-picrylhydrazyl radicals (DPPH), and superoxide anion radicals. In vivo hepatoprotective studies were performed in seven groups (n = 6) of Wistar rats. The test groups were pretreated with compounds (10 mg/kg body weight, po) orally for 30 min before the intraperitoneal administration of a dose of 20% CCl4 diluted with dietary cooking oil. Moreover, compounds were also tested for β-glucuronidase inhibition to explore the relationship between hepatoprotection and radical scavenging potential.
Results: The test compounds 1–4 were found to exhibit antiradical activity against 1,1-diphenyl-2-picrylhydrazyl radicals with IC50 values ranging between 54 and 138 µM, whereas aurone derivatives 3 and 4 additionally exhibited superoxide anion scavenging effects with IC50 values of 95 and 87 µM, respectively. In addition, these compounds were found to be weak inhibitors of xanthine oxidase (IC50 ≥1000 µM). In animal model, pretreatment with compounds 2–4 significantly blocked the CCl4-induced increase in the levels of the serum biochemical markers.
Conclusion: It appears that the hepatoprotection afforded by these compounds was mainly due to their radical scavenging activity that protected the cells from the free radicals generated by CCl4-induced hepatotoxicity.
Introduction
Liver plays critical role in the metabolism, detoxifying, secretion, and storage functions in the body and liver injury is mainly associated with disruption of these functions (CitationWolf, 1999). With many other factors involved in progression of liver diseases, production of large amounts of reactive oxygen species (ROS) has also been shown a strong relation to hepatotoxicity (CitationMehendale et al., 1994; CitationStohs, 1995). Efficient radical scavengers may prevent or alleviate many diseases associated with free radicals generation. In the course of searching for hepatoprotective agents from natural sources, we evaluated the chemical constituents of marine brown alga, Spatoglossum variable Figari et DE Notar (Dictyoaceae) for hepatoprotective activity in CCl4-induced liver damage in Wistar rats.
CCl4 has been widely used in animal models to investigate chemical toxin-induced liver damage (CitationHuang et al., 2010; CitationSrivastava & Shivanandappa, 2010). It has been accepted that CCl4 is converted into the trichloromethyl-free radicals (•CCl3) by the NADPH-cytochrome P-450 system, i.e., by transfer of an electron from NADPH to CCl4 (CitationZhu & Fung, 2000). Studies suggested that resulting radical species (•CCl3) react with the oxygen to form (trichloromethyl peroxyl radical) CCl3OO• (CitationConnor et al., 1986; CitationShyur et al., 2008). CCl3OO• is reactive free radical capable of initiating lipid peroxidation of cellular and organelle membranes, which damages the integrity of biomembranes. This leads to the release of large quantities of cytosolic enzymes into blood, causing swelling and necrosis of liver cells (CitationTakahashi et al., 1996). The estimation of serum aspartate aminotransferase (sAST), alanine aminotransferase (ALT), and bilirubins is largely used for the assessment of liver damage by free radicals generated by CCl4. Necrosis or membrane damage results in the release of these enzymes into blood circulation, which can be easily estimated (CitationFerreira et al., 2003). Based on this mechanism of liver cell necrosis by CCl4-induced liver toxicity, radical scavenging activities were evaluated before attempting to evaluate hepatoprotective effects of compounds from marine brown alga.
Seaweeds belong to one of several groups of multicellular alga found throughout the world’s oceans and seas. These substances have long been used in food as well as traditional remedies in Asian countries such as China, Japan, and Korea. Early Chinese Pharmacopoeia and Materia Medica recommended seaweeds for diverse maladies as antigoitre, antioedema, antipyretic, antitumor, and diuretic agents and were regarded as valuable drugs for their expectorant functions (CitationKhan, 1994). They are sources of vitamins, minerals, and commercially valuable polysaccharides such as alginate, agar, and carrageen. Moreover, seaweed extracts have extensive industrial applications, for example, in the extraction of phycocolloids and certain biochemicals. They are also reported to be rich sources of bioactive natural substances with antioxidant and hepatoprotective potential. Some bioactive metabolites from seaweeds, such as sulfated polysaccharides, have antiviral activity, and other metabolites such as Kahalalide F from Bryopsis species are possible drug candidates for the treatment of lung cancer and tumors (CitationWong et al., 2000; CitationSmit, 2004; CitationKim et al., 2005; CitationIwaki, 2008). The hypolipidemic activities of ethanol extracts of Spatoglossum asperum J.Agardh (Dictyoaceae), Solieria robusta (Grev.) Kylin (Solieriaceae), Colpomenia sinuosa Mert. ex Roth (Scytosiphonaceae), and Caulerpa racemosa (Forsskal) J.Agardh (Caulerpaceae) have also been investigated (CitationAra et al., 2002). Moreover, the use of seaweeds as human food, industrial materials, and for the extraction of new bioactive components is occurring worldwide. So far, many bioactive metabolites have been isolated from marine alga, and others are under investigation. In our institutions, we are engaged in identifying and characterizing bioactive metabolites from marine alga that belong to different orders such as Dictyotales and Chlorophyta (CitationAhmad & Ali, 1990; CitationAli et al., 1999, 2002, 2003; CitationAli & Pervez, 2003). Dictyotales is the most thoroughly investigated order of brown alga (CitationKonig & Wridgt, 1993) in phytochemical terms, and investigation of the biological activities of marine brown alga belonging to Dictyotales has shown that these exhibit antibacterial, antiviral, and cytotoxic activities (CitationGerwick et al., 1981). Previously, our group reported new metabolites (cinnamic acid ester and aurone derivatives) from the marine brown alga Spatoglossum variabile (CitationAtta-Ur-Rahman et al., 2001; CitationHayat et al., 2002). In this study, we now report the hepatoprotective activities in relation to free radical scavenging potential as well as the β-glucuronidase inhibitory effects and cytotoxicity of the cinnamic acid ester derivatives n-butyl 3,5-dimethoxy-4-hydroxycinnamate 1 and isopropyl 3,5-dimethoxy-4-hydroxycinnamate 2 and the aurone derivatives 4′-chloro-2-hydroxyaurone 3 and 4′-chloroaurone 4 from the marine brown alga S. variabile.
Materials and methods
Chemicals and instruments
The following commercially available chemicals were all purchased from Sigma Chemical Co. (Steinheim, Germany): 1,1-diphenyl-2-picrylhydrazyl radical (DPPH), reduced β-nicotinamide adenine dinucleotide (NADH), 5-methylphenazium methyl sulfate (PMS), nitroblue tetrazolium salt (NBT), standard radical scavenger propyl gallate, Triton X-100, dimethylsulfoxide, carbon tetrachloride, ethanol, xanthine oxidase (from butter milk, EC 1.1.3.22), β-glucuronidase (Escherichia coli, EC 3.2.1.31), xanthine, p-nitrophenyl-β-d-glucuronide, allopurinol, and saccharic acid 1,4-lactone. 4-[3-(4-Iodophenyl)-2-(4-nitropheyl)-2H-5-tetrazolio]-1,3-benzene disulfonate (WST-1) was purchased from Dojindo Laboratories (Kumamoto, Japan), and ficoll-paque was obtained from Pharmacia Biotech (Dubendorf, Switzerland). Studies were performed in 96-well microtiter plates using Spectra Max-340 and Spectra Max-384 spectrophotometers (Molecular Devices, Sunnyvale, CA, USA).
Animals
Adult male Wistar rats (150–220 g) were bred in the animal house of the International Center for Chemical and Biological Sciences (ICCBS), University of Karachi, Karachi. The animals were kept on a 12-h light/12-h dark cycle and fed a standard laboratory diet and tap water ad libitum. All animal experimental procedures were carried out in accordance with the international guidelines for the care and use of laboratory animals and with local ethical committee approval (Approval No. 1106001).
Antiradical activities
DPPH radicals scavenging studies
The reaction mixture contained a test sample in DMSO (5 µL) and DPPH in ethanol (95 µL). Different concentrations (1000–10 µM) of test samples were taken, while the DPPH concentration was kept constant at 300 µM. The reaction was then incubated at 37°C for 30 min, and the absorbance was measured at 515 nm (CitationLee et al., 1998).
Superoxide anion scavenging studies
The reaction mixture contained reduced NADH (280 µM, 40 µL), NBT (80 µM, 40 µL), PMS (8 µM, 20 µL), and 0.1 M phosphate buffer (pH 7.5, 90 µL). The concentration of the test samples (10 µL) ranged from 1000–10 µM. NBT, NADH, and PMS were prepared in 0.1 M phosphate buffer (pH 7.5), and the test samples were dissolved in DMSO. The reaction was performed in 96-well microtiter plates at 28°C, and the absorbance was measured at 560 nm (CitationSaint-Cricq de Gualejac et al., 1999).
The DPPH radical scavenging and superoxide scavenging activities (%) were calculated using the formula: % RSA = [100 – (AS/AC) × 100], where RSA represents the radical scavenging activity, AS is the absorbance of DPPH radicals and formazan dye in the presence of the test sample, and AC is the absorbance of the DPPH radicals and formazan dye without sample (control). The IC50 values of the compounds were calculated using the EZ-Fit Enzyme Kinetic Program (Perrella Scientific Inc., Amherst, MA, USA).
Xanthine oxidase inhibition studies
The reaction mixture contained different concentrations (1000–200 µM) of the test samples (10 µL), xanthine (100 µM, 20 µL), xanthine oxidase (0.003 U, 20 µL), and 0.1 M sodium phosphate buffer (pH 7.5, 150 µL). The test samples were dissolved in DMSO, whereas xanthine was initially dissolved in a minimum volume of 0.1 N NaOH, and the volume was made up to the required volume with sodium phosphate buffer (pH 7.8). Xanthine oxidase was prepared in the same buffer. The reaction was performed in 96-well microtiter plates at 28°C for 10 min, and the absorbance was measured at 295 nm (CitationLee et al., 1998).
Hepatoprotective studies by CCl4-induced toxicity
Hepatoprotective studies were performed by the method of Kapil with slight modifications (CitationKapil et al., 1995). Wistar rats were divided into seven groups of six animals. Group 1 was the normal control group, group 2 was the pathological control group, and groups 3–6 were the test groups. Group 1 served as the vehicle control and received only normal saline. Group 2 received an ip dose of 20% CCl4 diluted with dietary cooking oil (1 mL/100 g body weight). The test groups 3–6 received test compounds at 10 mg/kg body weight, po. Wistar rats were pretreated with test compounds orally for 30 min before the intraperitoneal administration of a single dose of 20% CCl4 diluted with dietary cooking oil (1 mL/100 g body weight). Group 7 served as the positive control and received propyl gallate in the same manner as the test groups. All the animals were sacrificed 48 h after CCl4 administration, and their blood was collected in test tubes. The blood samples were allowed to clot for 45 min at room temperature. The serum was then separated by centrifugation at 3000 rpm at room temperature for 15 min, and various biochemical parameters were measured. Propyl gallate was used to compare the activities of the test compounds. The levels of the sAST and sALT enzymes together with those of total and direct bilirubin were analyzed by biochemical analysis of the serum using a standard kit (Boehringer Mannheim) (CitationJendrassik & Grof, 1938; CitationSherlock, 1951; CitationBergmeyer et al., 1980; 1986).
β-Glucuronidase inhibition studies
The reaction mixture contained test sample (10 µL), β-glucuronidase (1 U, 10 µL), p-nitrophenol-β-glucuronide (0.4 mM, 50 µL), and Na-acetate buffer (pH 7.0, 180 µL). p-Nitrophenol release was continuously measured at 405 nm for 30 min (CitationCollins et al., 1997). The IC50 of the compounds was determined by monitoring the inhibitory effect of compounds at different concentrations. For studying the kinetics, the enzyme (1 U/300 µL) was incubated with different concentrations of the inhibitor for 30 min at 37°C. The reaction was then initiated by adding four different concentrations (0.1333–0.333 mM) of the substrate p-nitrophenol-β-glucuronide, and product formation was continuously measured at 405 nm for 15 min on a multiplate reader. Each experiment was performed in triplicate. The type of inhibition was determined by plotting Lineweaver-Burk and Dixon plots as well as their secondary plots. The Ki values were determined by interpreting the Dixon plot and the secondary plots of the Lineweaver-Burk plots. In the case of uncompetitive inhibition, the secondary plots of the Lineweaver-Burk plot were constructed by plotting the 1/Vmaxapp and 1/Kmapp against the inhibitor concentrations. However, the values of Kmapp were determined from the line intersection on the x axis, whereas the values of 1/Vmaxapp were determined by the intersection point of each line on the y axis of the Lineweaver-Burk plot. For mixed-type inhibition, the secondary plots of the Lineweaver-Burk and Dixon plots were drawn by plotting the slope and 1/Vmaxapp and slope, respectively, against the concentrations of the inhibitor. All graphs were plotted using the GraFit program (Leatherbarrow, R. J. GraFit; 4.09 ed.; Erithacus Software Ltd., Staines, UK).
Cytotoxicity studies
Cytotoxicity studies were performed by the method of Tan and Berridge with modifications (CitationTan & Berridge, 2000). Heparinized fresh venous blood was drawn from healthy volunteers in a blood bank (Fatmid Foundation, Karachi, Pakistan). Neutrophils were isolated by the method of CitationSiddiqui et al. (1995) by mixing blood with ficoll-paque in a ratio of 1:3. The pellets that settled to the bottom of the test tube were collected. The RBCs were lysed with an ammonium chloride solution. Finally, the pellets were centrifuged for 30 min and washed with the same PBS (pH 7.4). Various concentrations (12.5–200 µg/mL, 25 µL) of the test compounds were incubated with the neutrophils (1 × 106 cells/mL, 150 µL) at 37°C for 30 min. The WST-1 tetrazolium salt (200 µM, 25 µL) was added to the reaction mixture, and this was incubated for 3 h at 37°C in a shaking water bath. After incubation, the final absorbance was measured at 450 nm. The sample solutions were initially dissolved in DMSO, and the required concentration was maintained using modified Hank’s solution (pH 7.4).
Statistical analysis
Numerical data from in vitro studies were expressed as mean ± SEM. The data obtained from the results of in vivo experiments were processed with the statistical software analysis of variance to evaluate the statistical differences between the treatments and the normal and pathological control groups. A confidence level of p < 0.05 was considered to be significant.
Results and discussion
Antioxidants are compounds that inhibit or slow down the oxidation processes promoted by ROS. Compounds possessing antioxidant properties may act by two basic mechanisms: a chain-breaking mechanism in which the compounds scavenge free radicals by donating electrons and another mechanism in which the compounds remove the sources of free radical generation either by inhibiting certain enzymes, e.g., xanthine oxidase, cyclooxygenase, or lipoxygenase, or by chelating transition metal ions (CitationVaya & Aviram, 2001). To determine the radical scavenging potential, cinnamic acid esters 1 and 2 and aurone derivatives 3 and 4 were studied by in vitro and in vivo methods. The in vitro radical scavenging potential was evaluated by monitoring the scavenging of DPPH and superoxide anion radicals. The DPPH scavenging potential was determined by measuring the decrease in the absorption of DPPH radicals at 517 nm (CitationLee et al., 1998). The radical scavenging potential was assessed in terms of the complete conversion of the DPPH radical to its corresponding reduced form by the test compounds at a concentration of 1 mM and subsequent calculation of the IC50 values, i.e., the concentration of the compound at which 50% of the radicals are scavenged or inhibited. The results showed that cinnamic acid esters 1 and 2 and aurone derivatives 3 and 4 had effective radical scavenging potential that was indicated by the lower IC50 values of these compounds (). Moreover, aurone derivative 3 exhibited significant radical scavenging potential and had the lowest IC50 value. The strong radical scavenging potential of these compounds was significant in comparison to that of the commonly used reference antioxidant propyl gallate, which is extensively used as a preservative. It is well known that the free radical scavenging activity mainly depends on the presence of easily removable hydrogen atoms in the molecule. The significance of the activity of propyl gallate is therefore due to the presence of three aromatic hydroxyl groups in its structure that can effectively donate hydrogen atoms to DPPH radicals. Comparison of the structures of the test compounds with that of propyl gallate provides an explanation for the IC50 values of the test compounds. Cinnamic acid ester derivatives 1 and 2 contain only one aromatic hydroxyl group that was probably the reason for the relatively higher IC50 values of these compounds in comparison to propyl gallate (). On the other hand, aurone derivatives 3 and 4 do not contain any aromatic hydroxyl functionality but still exhibit significant radical scavenging activity that might arise from the removal of the hydrogen atom from C-10. The proposed mechanism of action of the removal of a hydrogen atom from the aurone derivative is shown in . To further explore the radical scavenging potential, these compounds were assessed for their superoxide anion scavenging capability by measuring the rate of formation of formazan dye at 560 nm in the nonenzymatically generated superoxide anion system of β-nicotinadeninedinucleotide/phenazine methosulfate/NBT (NADH/PMS/NBT) (CitationSaint-Cricq de Gualejac et al., 1999). Superoxide anions (O2•–) are a reduced form of oxygen and play an important role in the formation of ROS in biological systems. Compounds with radical scavenging activity may neutralize O2•–. In this study, aurone derivatives 3 and 4 exhibited significant superoxide anion scavenging potential with lower IC50 values than propyl gallate, whereas cinnamic acid esters 1 and 2 were found to be completely inactive in this system (). These studies also revealed the peculiar efficacy of aurone derivatives 3 and 4 as both DPPH radicals and O2•– scavengers.
Table 1. In vitro radical scavenging activities.
Figure 1 . Cinnamic acid ester and aurone derivatives from Spatoglossum variabile: (1) n-Butyl 3,5-dimethoxy-4-hydroxycinnamate; (2) isopropyl 3,5-dimethoxy-4-hydroxycinnamate; (3) 4′-chloro-2-hydroxyaurone; (4) 4′-chloroaurone.
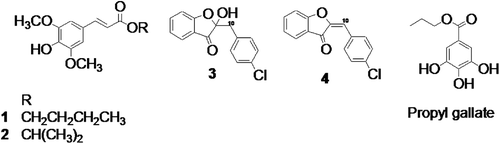
We have discussed the results based on the first mechanism related to the chain-breaking ability of compounds. The second mechanism involves the removal of ROS, and these compounds were evaluated for their xanthine oxidase inhibition potential. Xanthine oxidase is one of the major sources of O2•– production in the biological environment (CitationChung et al., 1997), and compounds that inhibit this enzyme may stop the overproduction of O2•–. To assess xanthine oxidase inhibition, potential compounds were incubated with the enzyme, and the rate of hydroxylation of the substrate xanthine was measured by the formation of uric acid at 295 nm (CitationLee et al., 1998). Compounds 1–4 showed weak inhibition of xanthine oxidase, i.e., <50% inhibition at concentrations of 1 mM. This indicated that these compounds may act as effective chain-breaking antioxidants with relatively weak inhibition potential of the xanthine oxidase enzyme.
Based on their radical scavenging activities, cinnamic acid esters 1 and 2 and aurone derivatives 3 and 4 were tested for their hepatoprotective activity in a CCl4-induced hepatotoxicity model in Wistar rats. The effect of CCl4 on sAST, serum ALT (sALT), and bilirubins was measured. It is well known that CCl4 is metabolized into the trichloromethyl-free radical (•CCl3) by the NADPH-cytochrome P-450 system through the transfer of an electron from NADPH to CCl4 (CitationZhu & Fung, 2000). Previous studies have suggested that •CCl3 reacts with oxygen to form the trichloromethyl peroxyl radical (CCl3OO•) (CitationConnor et al., 1986; CitationShyur et al., 2008). CCl3OO• and •CCl3 are reactive free radical species that initiate lipid peroxidation of cellular and organelle membranes and damage the integrity of biomembranes. As a result, large quantities of cytosolic enzymes are released into the blood resulting in swelling and necrosis of the liver cells (CitationTakahashi et al., 1996). Estimation of the levels of the sAST and sALT enzymes as well as those of total, direct, and indirect bilirubins is widely used to assess liver damage by free radicals (CitationFerreira et al., 2003). The effect of cinnamic acid esters 1 and 2 and aurone derivatives 3 and 4 on CCl4-induced hepatotoxicity was studied by pretreating the animals at a dose of 10 mg/kg body weight. The normal control group received only saline solution, whereas the pathological control was administered an intraperitoneal dose of CCl4 (20% in edible oil, 1 mL/100 g body weight). The effect of saline solution and CCl4 was monitored by estimating the levels of sAST, sALT, and bilirubins. There were significant differences between the pathological and normal control groups in terms of the levels of hepatic transaminases and bilirubins. A significant increase was observed in the levels of serum bilirubins, sAST, and sALT in the case of the pathological control. The hepatoprotective effects of cinnamic acid esters 1 and 2 and aurone derivatives 3 and 4 were compared with those of the pathological control group (). Among these compounds, cinnamic acid ester 2 was found to be the most hepatoprotective as indicated by the lower values of serum biochemical parameters, sAST, and sALT as well as total, direct, and indirect bilirubins. Aurone derivatives 3 and 4 also protected the hepatic cells as evidenced by the values of sAST, sALT, and bilirubins, which were lower than those of the pathological control. On the other hand, cinnamic acid ester 1 was not as effective as compounds 3 and 4.
Table 2. Effects of pretreatment with chemical constituents of marine brown alga Spatoglossum variabile on serum AST and ALT and bilirubin in CCl4-intoxicated Wistar rats (n = 6).
In the liver, both glucuronidation and deglucuronidation are highly regulated processes. Xenobiotics and endogenous metabolites are excreted after conjugation, and some are recovered upon deglucuronidation by the action of β-glucuronidase that catalyzes the hydrolysis of β-glucuronide conjugates of exogenous and endogenous compounds (CitationBohnenstengel et al., 1999). Since this enzyme is present in liver microsomes, any damage to liver cells increases the β-glucuronidase concentration in blood, which may lead to liver cancer. Therefore, damage to the structural integrity of the liver may be reflected by the elevated concentration of β-glucuronidase, and inhibition of the enzyme at this stage may protect the liver (CitationShim et al., 2000). To investigate whether the hepatic protection extended by the tested compounds was due to the radical scavenging capability of compounds 1–4 or due to their β-glucuronidase inhibition potential, the in vitro β-glucuronidase inhibition potential was determined. For this, the rate of hydrolysis of the substrate p-nitrophenyl-β-d-glucuronide was measured based on the formation of the p-nitrophenolate anion at 405 nm (CitationCollins et al., 1997). As a result, cinnamic acid ester derivatives 1 and 2 were found to be a new class of β-glucuronidase inhibitors, whereas aurone derivate 3 did not show any inhibition and compound 4 showed weak inhibitory potential (). These results proved that hepatic protection was mainly due to the radical scavenging abilities of these compounds because compound 1, which exhibited β-glucuronidase inhibition potential with the lowest IC50 value of 25.3 µM, could not provide effective hepatic protection (). On the other hand, compound 3, which was completely inactive against the β-glucuronidase enzyme, could successfully provide hepatic protection (). As mentioned earlier, compounds 1 and 2 belong to a new class of β-glucuronidase inhibitors, and these were further investigated by kinetic studies. Kinetic studies of cinnamic acid ester 1 showed that it was a mixed-type inhibitor of β-glucuronidase with a Ki value (the dissociation constant of the enzyme-inhibitor complex into free enzyme and inhibitor) of 28.0 µM (). Cinnamic acid ester 2 showed an uncompetitive mode of inhibition with a Ki value of 680 µM (). Aurone derivative 4 showed weak inhibition of β-glucuronidase in comparison to cinnamic acid esters 1 and 2, whereas aurone derivative 3 was found to be completely inactive. The results of β-glucuronidase inhibition are shown in .
Table 3. In vitro β-glucuronidase inhibitory activities.
Figure 3 . Steady-state inhibition of β-glucuronidase by n-butyl 3,5-dimethoxy 4-hydroxyl cinnamate 1: (A) Lineweaver-Burk plot of reciprocal of the initial velocities versus reciprocal of p-nitrophenyl-β-d-glucuronide concentrations. (B) Dixon plot of reciprocal of the initial velocities versus various concentrations of inhibitor. Each point in the graphs represents the mean of three experiments.
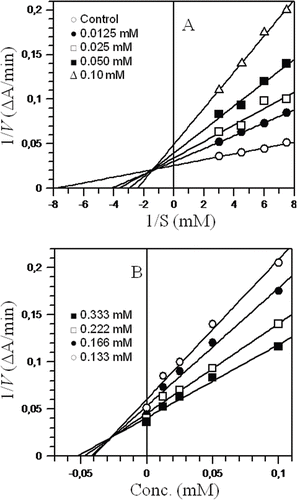
Figure 4 . Steady-state inhibition of β-glucuronidase isopropyl 3,5-dimethoxy 4-hydroxyl cinnamate 2: (A) Lineweaver-Burk plot of reciprocal of the initial velocities versus reciprocal of p-nitrophenyl-β-d-glucuronide concentrations. (B) Dixon plot of reciprocal of the initial velocities versus various concentrations of inhibitor. Each point in the graphs represents the mean of three experiments.
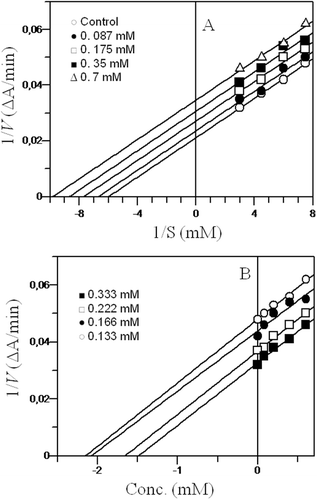
All of these compounds were also evaluated for their cytotoxicity using freshly isolated human neutrophils. An in vitro spectrophotometric method was used, which measures cell viability (%) by monitoring (at 450 nm) the reduction of the tetrazolium salt 4-[3-(4-iodophenyl)-2-(4-nitrophenyl)-2H-5-tetrazolio]-1,3-benzene disulfonate (WST-1) to formazan dye by the mitochondrial dehydrogenases of viable cells after incubation of the test samples with neutrophils (CitationTan & Berridge, 2000). The cytotoxic effects of cinnamic acid esters 1 and 2 and aurone derivatives 3 and 4 on the viability of neutrophils was studied at different doses of these metabolites in the range 25–200 µg/mL. The cell viability was found to range from 99.4% to 65.27%. Measurement of the effective dose at which 50% of the cells are viable (known as the ED50 value) showed that aurone derivatives 3 and 4 were nontoxic up to a dose of 200 µg/mL, whereas cinnamic acid ester 2 showed less cytotoxicity, as indicated by its ED50 value that was relatively lower than that of the aurone derivatives. On the other hand, cinnamic acid ester 1 showed cytotoxicity with a much lower ED50 value ().
Table 4. Cytotoxicity against human neutrophils.
Conclusion
The results of this study showed that aurone derivatives 3 and 4 possessed antiradical potential as evidenced by the scavenging of DPPH radicals and superoxide anion, while they were found to be only weak inhibitors of xanthine oxidase. On the other hand, cinnamic acid ester derivatives 1 and 2 showed DPPH radicals scavenging activities lower than those of aurone derivatives 3 and 4 and did not possess any superoxide anion scavenging activity. An in vivo study indicated that the levels of sAST and sALT as well as those of total, direct, and indirect bilirubins were significantly maintained (p < 0.05) by pretreatment with cinnamic acid ester 2 and aurone derivatives 3 and 4. Among these compounds, the most hepatoprotective molecule was cinnamic acid ester derivative 2. The reduction in the elevation of cytosolic enzymes is probably due to the interaction of CCl4-generated free radicals with hepatic cells. Moreover, these compounds exhibited significant free radical scavenging activity in an in vitro study; therefore, it was deduced that these compounds may provide hepatoprotection by acting as free radical scavengers. This was supported by the results of inhibition studies of the β-glucuronidase enzyme that might be involved in liver damage when its levels are elevated in the blood. It was shown that cinnamic acid ester derivative 1, the most potent inhibitor of β-glucuronidase, did not show significant hepatoprotection. However, further investigations are needed to understand the exact mechanism of action behind the hepatoprotective effect of these compounds. Moreover, the noncytotoxic and hepatoprotective effect of aurone derivatives 3 and 4 and the hepatoprotective effect of cinnamic acid ester derivative 2 suggests that these compounds should be further investigated for their beneficial effects on certain pathological conditions that arise due to the damaging effect of free radicals.
Declaration of interest
The authors declared no conflict of interest.
References
- Ahmad VU, Ali MS. (1990). Marine natural products XII: Laurol, a new metabolite from the red alga Laurencia pinnatifida. Pol J Chem, 58, 299–301.
- Ali MS, Ahmad UV, Mazhar F, Azhar I, Usman Ghani KA. (1999). Some chemical constituents from marine algae of Karachi (Arabian Sea). Turk J Chem, 23, 181–84.
- Ali MS, Pervez MK. (2003). Ring-A hydroxylated dolastanes from the marine brown alga Dictyota dichotoma (Huds.) Lamour. Nat Prod Res, 17, 281–286.
- Ali MS, Pervez MK, Saleem M, Ahmed F. (2003). Dichotenone-a and -b: Two new enones from the marine brown alga Dictyota dichotoma (Hudson) Lamour. Nat Prod Res, 17, 301–306.
- Ali MS, Saleem M, Yamdagni R, Ali MA. (2002). Steroid and antibacterial steroidal glycosides from marine green alga Codium iyengarii Borgesen. Nat Prod Lett, 16, 407–413.
- Ara J, Sultana V, Qasim R, Ahmad VU. (2002). Hypolipidaemic activity of seaweed from Karachi coast. Phytother Res, 16, 479–483.
- Atta-Ur-Rahman, Choudhary MI, Hayat S, Khan AM, Ahmad A. (2001). Two new aurones from marine brown alga Spatoglossum variabile. Chem Pharm Bull, 49, 105–107.
- Bergmeyer HU, Hørder M, Rej R. (1986). International Federation of Clinical Chemistry (IFCC) Scientific Committee, Analytical Section: Approved recommendation (1985) on IFCC methods for the measurement of catalytic concentration of enzymes. Part 2. IFCC method for aspartate aminotransferase (l-aspartate: 2-oxoglutarate aminotransferase, EC 2.6.1.1). J Clin Chem Clin Biochem, 24, 497–510.
- Bergmeyer HU, Horder M, Rej R. (1980). IFCC methods for the measurement of catalytic concentrations of enzymes. Part 3, IFCC. Method for alanine aminotransferase (l-alanine: 2-oxoglutarate aminotransferase, EC 2.6.1.2). J Clin Chim Acta, 105, 145F–172F.
- Bohnenstengel F, Kroemer HK, Sperker B. (1999). In vitro cleavage of paracetamol glucuronide by human liver and kidney beta-glucuronidase: determination of paracetamol by capillary electrophoresis. J Chromatogr B Biomed Sci Appl, 721, 295–299.
- Chung HY, Baek BS, Song SH, Kim MS, Huh JI, Shim KH, Kim KW, Lee KH. (1997). Xanthine dehydrogenase/xanthine oxidase and oxidative stress. Age, 20, 127–139.
- Collins RA, Ng TB, Fong WP, Wan CC, Yeung HW. (1997). Inhibition of glycohydrolase enzymes by aqueous extracts of Chinese medicinal herbs in a microplate format. Biochem Mol Biol Int, 42, 1163–1169.
- Connor HD, Thurman RG, Galizi MD, Mason RP. (1986). The formation of a novel free radical metabolite from CCl4 in the perfused rat liver and in vivo. J Biol Chem, 261, 4542–4548.
- Ferreira MA, Nunes Odel R, Leal LK, Pessoa OD, Lemos TL, Viana GS. (2003). Antioxidant effects in the quinone fraction from Auxemma oncocalyx TAUB. Biol Pharm Bull, 26, 595–599.
- Gerwick WH, Fenical W, Sultanbawa MUS. (1981). Spatane diterpenoids from the tropical marine alga Stoechospermum marginatum (Dictyotaceae). J Org Chem, 46, 2233–2241.
- Hayat S, Atta-ur-Rahman, Choudhary MI, Khan KM, Abbaskhan A. (2002). Two new cinnamic acid esters from marine brown alga Spatoglossum variabile. Chem Pharm Bull, 50, 1297–1299.
- Huang B, Ban X, He J, Tong J, Tian J, Wang Y. (2010). Hepatoprotective and antioxidant activity of ethanolic extracts of edible lotus (Nelumbo nucifera Gaertn.) leaves. Food Chem, 120, 873–878.
- Iwaki K. (2008). Antidiabetic and antioxidant effects of polyphenols in brown alga Ecklonia stolonifera in genetically diabetic KK-Ay mice. Plant Food Hum Nutr, 63, 163–169.
- Jendrassik L, Grof P. (1938). Photometric determination of bilirubin in urine. Biochem Z, 296, 71–79.
- Kapil A, Koul IB. Suri OP. (1995). Antihepatotoxic effects of chlorogenic acid from Anthocephalus cadamba. Phytother Res, 9, 189–193.
- Khan MMT. (1994). Drug from the ocean. In: Dandiya PC, Vohra SB (eds.). Research and Development of Indigenous Drugs. New Delhi, India: IHMMR Hamdard Nagar, 104–112.
- Kim YC, An RB, Yoon NY, Nam TJ, Choi JS. (2005). Hepatoprotective constituents of the edible brown alga Ecklonia stolonifera on tacrine-induced cytotoxicity in Hep G2 cells. Arch Pharm Res, 28, 1376–1378.
- Konig GM, Wrigt AD. (1993). Human medicinal agents from plants. Washington, DC: American Chemical Society.
- Lee SK, Mbwambo ZH, Chung H, Luyengi L, Gamez EJ, Mehta RG, Kinghorn AD, Pezzuto JM. (1998). Evaluation of the antioxidant potential of natural products. Comb Chem High Throughput Screen, 1, 35–46.
- Mehendale HM, Roth RA, Gandolfi AJ, Klaunig JE, Lemasters JJ, Curtis LR. (1994). Novel mechanisms in chemically induced hepatotoxicity. FASEB J, 8, 1285–1295.
- Saint-Cricq de Gualejac N, Glories Y, Vivas N. (1999). Free radical scavenging effect of anthocyanins in red wines. Food Res Int, 32, 327–333.
- Schomberg D, Salzmann M. (1991). Enzyme Handbook. Berlin/New York: Springer-Verlang.
- Sherlock S. (1951). Liver disease. London, England: Churchill.
- Shim SB, Kim NJ, Kim DH. (2000). beta-Glucuronidase inhibitory activity and hepatoprotective effect of 18 beta-glycyrrhetinic acid from the rhizomes of Glycyrrhiza uralensis. Planta Med, 66, 40–43.
- Shyur LF, Huang CC, Lo CP, Chiu CY, Chen YP, Wang SY, Chang ST. (2008). Hepatoprotective phytocompounds from Cryptomeria japonica are potent modulators of inflammatory mediators. Phytochemistry, 69, 1348–1358.
- Siddiqui RA, English D, Harvey K, Cui Y, Martin MI, Wentland J, Akard L, Jansen J, Thompson J, Garcia JG. (1995). Phorbol ester-induced priming of superoxide generation by phosphatidic acid-stimulated neutrophils and granule-free neutrophil cytoplasts. J Leukoc Biol, 58, 189–195.
- Smit AJ. (2004). Medicinal and pharmaceutical uses of seaweed natural products: A review. J Appl Phycol, 16, 245–262.
- Srivastava A, Shivanandappa T. (2010). Hepatoprotective effect of the root extract of Decalepis hamiltonii against carbon tetrachloride-induced oxidative stress in rats. Food Chem, 118, 411–417.
- Stohs SJ. (1995). The role of free radicals in toxicity and disease. J Basic Clin Physiol Pharmacol, 6, 205–228.
- Takahashi T, Sugimoto N, Takahata K, Okamoto T, Kishi T. (1996). Cellular antioxidant defense by a ubiquinol-regenerating system coupled with cytosolic NADPH-dependent ubiquinone reductase: protective effect against carbon tetrachloride-induced hepatotoxicity in the rat. Biol Pharm Bull, 19, 1005–1012.
- Tan AS, Berridge MV. (2000). Superoxide produced by activated neutrophils efficiently reduces the tetrazolium salt, WST-1 to produce a soluble formazan: A simple colorimetric assay for measuring respiratory burst activation and for screening anti-inflammatory agents. J Immunol Methods, 238, 59–68.
- Vaya J, Aviram M. (2001). Nutritional antioxidants: Mechanism of action, analyses of activities and medical applications. Curr Med Chem-Imm Endoc & Metab Agents, 1, 99–117.
- Wolf PL. (1999). Biochemical diagnosis of liver diseases. Indian J Clin Biochem, 14, 59–90.
- Wong CK, Ooi VE, Ang PO. (2000). Protective effects of seaweeds against liver injury caused by carbon tetrachloride in rats. Chemosphere, 41, 173–176.
- Zhu W, Fung PC. (2000). The roles played by crucial free radicals like lipid free radicals, nitric oxide, and enzymes NOS and NADPH in CCl(4)-induced acute liver injury of mice. Free Radic Biol Med, 29, 870–880.