Abstract
Context: Homocysteine-induced endothelial cellular senescence may contribute to some cardiovascular disorders. Icariin (ICA), a flavonoid derived from Epimedium sagittatum Maxim. (Berberidaceae), has been reported to increase production of nitric oxide (NO) and reduce reactive oxygen species (ROS) levels in human umbilical vein endothelial cells (HUVECs).
Objective: To observe the effects of ICA on homocysteine-induced senescence and the underlying mechanisms in HUVECs.
Materials and methods: ICA at concentrations of 0.1, 1, and 5 μM was added into homocysteine pretreated HUVECs. Cellular senescence was assayed by senescence-associated β-galactosidase (SA-β-gal) staining and cumulative population doublings (CPDs). ICA (5 μM) was given orally to homocysteine-treated rats, luminal surface of aortic artery of rats was subjected to SA-β-gal staining. Protein expression was measured by western blot.
Results: Homocysteine significantly increased cellular senescence both in vitro and in vivo. After treatment by ICA, the percentage of SA-β-gal-positive cells, and the ROS level significantly decreased. The CPDs were partially restored. ICA also significantly reduced the mean density of SA-β-gal staining in vivo. We found that NO production and phosphorylation of AKT, ERK, and endothelial NO synthase (eNOS) were elevated by ICA in HUVECs. Furthermore, the increased level of NO production was fully abolished by the phosphatidylinositol-3-kinase (PI3K) inhibitor wortmannin. The mitogen-activated protein kinase (MEK) inhibitor PD98059, which can inhibit phosphorylation of ERK, did not show this ability.
Discussion and conclusion: Our results indicate that ICA delays homocyteine-induced endothelial senescence in vitro and in vivo. Activation of PI3K/Akt-eNOS-dependent signaling pathway may be responsible for this efficacy of ICA.
Keywords::
Introduction
A great deal of evidence supports the idea that endothelial cellular senescence plays an important role in pathogenesis of cardiovascular diseases including atherosclerosis (CitationFenton et al., 2001; CitationMinamino et al., 2002; CitationErusalimsky, 2009). Senescent endothelial cells show impaired nitric oxide (NO) function system and acquire a distinct secretary phenotype including a set of pro-inflammatory cytokines and adhesion molecules (CitationChen & Goligorsky, 2006). In mammals, homocysteine is derived from methionine as a result of cellular methylation reactions. Hyperhomocysteinemia was identified to be an independent risk factor for atherosclerosis and other cardiovascular diseases (CitationClarke et al., 1991; CitationLawrence de Koning et al., 2003; CitationAustin et al., 2004; CitationZhou et al., 2005). A recent systematic meta-analysis showed that each increase of 5 µM in homocysteine level increases the risk of chronic heart diseases by ~20%, independent of other chronic heart disease risk factors (CitationClarke et al., 1991). Induction of cellular senescence by homocysteine may contribute to its adverse effects. It accelerated endothelial cellular senescence, enhanced telomere length loss, increased protein expression of intracellular adhesion molecule-1 (CitationXu et al., 2000; CitationZhu et al., 2006) and chronic oxidative stress (CitationPerez-de-Arce et al., 2005). Homocysteine also induced senescence of endothelial progenitor cells, possibly through decrease of telomerase activity and AKT phosphorylation (CitationZhu et al., 2006).
Icariin (ICA, C33H40O15; molecular weight: 676.67; ) is a flavonoid isolated from the traditional Chinese herbal medicine Epimedium sagittatum Maxim. (Berberidaceae) has been proven to possess efficacy on osteoporosis, cardiovascular diseases, and to improve sexual function and immunoregulation (CitationYap et al., 2007; CitationZhang et al., 2007; CitationSong et al., 2008). ICA is the major pharmacological active component of this herb. It has been shown to have beneficial effects on cardiovascular functions (CitationXu & Huang, 2007), have antioxidant effect and exert protective effects on human umbilical vein endothelial cells (HUVECs) injury induced by H2O2 in vitro (CitationWang & Huang, 2005). ICA also increased production of NO and endothelial NO synthase (eNOS) expression in HUVECs (CitationChung et al., 2008). These reports suggest that ICA may delay homocysteine-induced endothelial cellular senescence, thus contributing to its beneficial effects on the cardiovascular system. However, these effects of ICA have not been investigated yet.
In the present study, we examined the effects of ICA on senescence of HUVECs in the presence of homocysteine in vitro and in vivo and explored its possible mechanisms. As a result, we found that ICA can delay homocysteine-induced endothelial cellular senescence. Activation of PI3K/AKT-eNOS signaling pathway is at least partially responsible for the effects of ICA.
Materials and methods
Materials
Homocysteine, wortmannin, and PD98059 were obtained from Sigma (St Louis, MO, USA). ICA with purity >98% was purchased from Shanghai Yousi Biotechnologies, Inc. (Shanghai, China). Endothelial cell base medium (EBM-2) and HUVECs were from Clonetics (Walkersville, MD, USA). Antibodies against p-AKT, AKT, p-ERK, ERK, p-eNOS, eNOS were procured from Cell Signaling Technology (Beverly, MA, USA). Fetal bovine serum was from GIBCO (Carlsbad, CA, USA). All other reagents were purchased from Beyotime Institute of Biotechnology (Shanghai, China) unless indicated otherwise.
Cell culture
HUVECs were maintained in EBM-2 supplemented with 10% fetal bovine serum. The cells were plated at a density of 1 × 104 cells/cm2 per 75 cm2 flasks or 6-well plates. The medium was replaced every 2 days. After reaching ~80–90% confluence, endothelial cells were trypsinized and counted. Cellular viability was determined by Trypan blue staining (0.5%) in physiological saline [phosphate-buffered saline (PBS)], usually with a viability >95% after trypsinization. Cells were cultured for 24 h in EBM-2 supplemented with 1% fetal bovine serum before being exposed to various experimental conditions. Population doubling (PD) was calculated at the end of each passage by the following formula: PD = (log10Y – log10X)/log102 (Y indicates the number of cells counted at the end of the passage; X the number of cells seeded). Cumulative population doublings (CPDs) were calculated as the sum of all the changes in PD.
SA-β-gal activity assay
Senescence-associated β-galactosidase (SA-β-gal) activity in cells and tissues was examined as described by CitationMinamino et al. (2002). In brief, HUVECs were fixed for 10 min in 2% formaldehyde, 0.2% glutaraldehyde in PBS, and incubated for 12 h at 37°C without CO2 with fresh β-gal staining solution: 1 mg/ml 5-bromo-4-chloro-3-indolyl-β-d-galactopyranoside, 5 mM potassium ferrocyanide, 5 mM potassium ferricyanide, and 2 mM MgCl2, pH 6.0. Cells were counterstained with 4′6-diamidinophenylindole (DAPI, 0.2 mg/ml in 10 mM NaCl) for 10 min to count the total cell number. The percentage of SA-β-gal-positive cells was determined by counting the number of blue cells within a total of 1,000 cells. All the SA-β-gal activity assays were performed in replicate and the experiment was repeated three times. For measurement of SA-β-gal positive cells in vivo, all work with rats was approved by the Animal Ethics Committee of Fudan University, Shanghai, China and was performed in accordance with the “Guide for the Care and Use of Laboratory Animals” published by the National Institutes of Health. Healthy 4-month-old male Wistar rats weighing 180–200 g, purchased from Experimental Animal Center, Fudan University, Shanghai, China, were randomly assigned to normal control group (Norm) (n = 6), homocysteine-given group (HCY) (n = 6), and ICA-treated homocysteine-given group (HCY+ICA) (n = 6). Animals were housed in an air-conditioned room at 23 ± 1°C with alternating 12 h cycles of light and dark. Animals were fed a standard diet and given water ad libitum. The control group was freely accessible to standard chow. The other two groups were given homocysteine by intragastric administration at dose of 100 mg·kg–1·day–1 with or without ICA at dose of 20 mg·kg–1·day–1 for 42 days. Rats were killed at end of the experiment, and the aortic artery was taken for fixation and SA-β-gal staining as above. Quantification of the SA-β-gal staining was accomplished by Image-Pro Plus Software. The image was opened in Image-Pro Plus in gray scale and staining density for representative areas was calculated. The mean staining density for each group was taken for further calculations and statistical analysis.
Measurement of nitrite
Total NO level including NO2– and NO3– were measured using a commercial assay kit from Beyotime Institute of Biotechnology which first reduces NO3– to NO2– by a nicotinamide adenine dinucleotide (NADH) dependent nitrate reductase, followed by the classical Griess reagent. The detailed protocol was consistent with instructions provided by the manufacture. Analysis was carried out in 96-well microtiter plates, and absorbance was read at a wavelength of 540 nm using a Bio-Tek Power Wave X340 microtiter plate spectrophotometer (Bio-Tek Instruments, Inc., Highland Park, VT, USA). Standard curves were prepared for each microtiter plate according to the kit instructions. The experiment was repeated three times.
Fluorescent analysis of reactive oxygen species (ROS) generation
Dihydroethidium (DHE) was used to stain intracellular ROS (CitationPeshavariya et al., 2007). DHE freely diffuses across the cell membrane, then is dehydrated, and incorporates into DNA and/or RNA of the cell. HUVECs (5000) in each well of a 96-well plate were incubated with homocystine for 3 days. Then 5 μM ICA was added and incubated with cells for 15 to 30 min, respectively, at 37°C. Subsequently, DHE was added into each well to a final concentration of 10 μM. After incubation at 37°C for 30 min, the dye was aspirated and the cells were washed once by PBS for 2 min to remove extracellular DHE. The fluorescence was measured at an excitation wavelength of 520 ± 10 nm and an emission wavelength of 610 ± 5 nm, using a multi-well fluorescent plate reader (Bio-Tek Power Wave X340; Bio-Tek Instruments, Inc.). This experiment was repeated three times.
Western blot
Cells in each of six-well plate were rinsed twice with cold PBS, followed by addition of cell lysis buffer containing 150 mM NaCl, 50 mM Tris-HCl, 5 mM EDTA, 1% Nonidet P-40, 0.5% deoxycholate, 1% SDS with proteinase inhibitor cocktail (Sigma) on ice for 15 min, and then centrifuged for 20 min at 13,000g. The supernatant was collected. The protein concentration was measured with the Bradford method. Total protein (50 mg) was dissolved in sample buffer and boiled for 5 min before loading onto polyacrylamide gels. Then proteins were transferred to polyvinylidine difluoride filter membranes, and blocked with 5% nonfat dry milk in Tris-buffered saline/0.05% Tween-20. The membrane was incubated with monoclonal antibody against AKT, p-AKT, ERK, p-ERK, eNOS, p-eNOS, followed by incubation with horseradish peroxidase-conjugated secondary antibodies and visualized with enhanced chemiluminescence (ECL) kit.
Statistics
All data are given as means ± SD. Analysis of variance (ANOVA) by using a least-significant difference (LSD) post hoc test or ANOVA for multiple comparisons was used (SPSS Software 11.0). Differences were considered to be significant at a value of p < 0.05.
Results
ICA inhibited homocysteine-induced endothelial cellular senescence in vitro and in vivo
To test the hypothesis that ICA prevents senescence induced by homocysteine, we established a model for human replicative cellular senescence using passaged HUVECs. Coculture of HUVECs with homocysteine resulted in an increase in percentage of SA-β-gal-positive cells with a maximum effects achieved at 400 μM (). In the prolonged culture, the number of SA-β-gal-positive cells at 400 μM progressively rose from ~5% at day 1 to 24% at day 7 (). Over the 7-day period in culture, homocysteine produced as much as about five-fold increase in the percentage of senescent endothelial cells. When HUVECs under 400 μM homocysteine stimulation were treated by ICA (0.1–5 μM), the percentage of SA-β-gal-positive cells were reduced significantly in a dose-dependent manner. ICA showed maximum effect at 5 μM (). Homocysteine inhibited CPDs of HUVECs (). On the other hand, 5 μM of ICA treatment relatively increased CPDs of HUVECs in each passage. The maximum effect of ICA on CPDs was achieved at passage 14 to 16 ().
Figure 2. Icariin (ICA) delayed the endothelial cellular senescence in vitro. (A) Human umbilical vein endothelial cells (HUVECs) were stimulated with 0, 50, 200, 400 μM homocysteine for 7 days, the percentage of senescence-associated β-galactosidase (SA-β-gal) positive cells were measured. (B) HUVECs cultured with 400 μM homocysteine were treated with 0.1 to 1, 5 μM ICA, the percentage of SA-β-gal positive cells were measured. (C) HUVECs exposed to homocysteine in the presence or absence of 5 μM ICA, the population doublings in each passage were observed. HCY, homocysteine; ICA, icariin. *p < 0.05 vs. control; **p < 0.01 vs. control; #p < 0.05 vs. HCY; ##p < 0.01 vs. HCY.
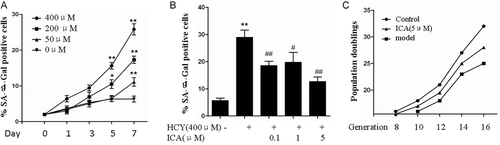
We further examined SA-β-gal activity in aortic vascular wall in homocysteine-administered rats. Marked β-gal staining (blue color) were observed in the luminal surface of rat aortic artery in HCY group rats. But in normally fed rats, little SA-β-gal staining was seen. In HCY+ICA rats, SA-β-gal staining was decreased (). Mean staining densities (arbitrary units) were 0.008 ± 0.004 for control group rats, 0.021 ± 0.008 for HCY group, and 0.014 ± 0.005 for HCY+ICA group. ICA significantly decreased the mean density of SA-β-gal staining compared to HCY group (p < 0.05). These results indicate that homocysteine induces the endothelial cellular senescence in vivo, which can be inhibited by ICA.
Figure 3. Icariin (ICA) decreases homocysteine-induced endothelial cellular senescence in luminal surface of rat aortic artery. Rats were given control diet, control diet plus homocysteine or control diet plus homocysteine and ICA for 42 days. Rat aortic arteries were fixed in 10% formaldehyde for 24 h and stained with senescence-associated β-galactosidase (SA-β-gal) staining solution. The values are presented as means ± SD, n = 6 samples each from an animal. Box indicates the areas calculated for mean density. HCY, homocysteine; ICA, icariin. *p < 0.05 vs. control; **p < 0.01 vs. control; #p < 0.05 vs. HCY.
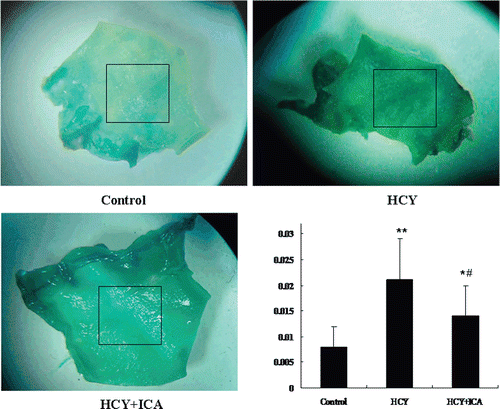
ICA rapidly reduced the ROS level in HUVECs
ROS are thought to be critical players in the development of cellular senescence. We therefore detected ROS in HUVEC exposed to homocysteine with or without ICA. We used DHE as a fluorescent probe to measure the ROS level of homocysteine-treated HUVECs for 3 days, and found homocysteine treatment increased the ROS level reflected by intracellular fluorescent intensity. To observe the rapid response of intracellular ROS level in HUVECs to ICA, 5 μM ICA were added into homocysteine-treated HUVECs culture for 30 to 60 min, respectively. As a result, ICA rapidly reduced the ROS level. Maximum effects were achieved at ~30 min ().
Figure 4. Icariin (ICA) rapidly reduced the intracellular reactive oxygen species (ROS) level in human umbilical vein endothelial cells (HUVECs). HUVECs exposed to 400 μM homocysteine were loaded with dihydroethidium (DHE). Then cells were treated with 5 μM ICA for 15 to 30 min. The fluorescence intensity was measured using a fluorescent plate reader at an excitation wavelength of 520 ± 5 nm and an emission wavelength of 620 ± 5 nm for DHE. The values are presented as means ± SD of three experiments. HCY, homocysteine; ICA, icariin. **p < 0.01 vs. control; ##p < 0.01 vs. HCY.
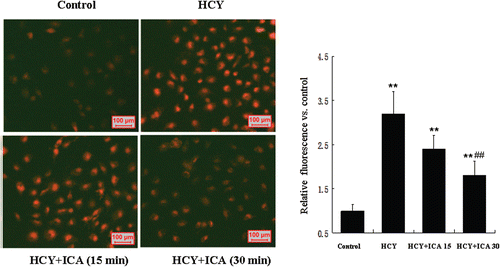
ICA stimulated phosphorylation of AKT, ERK, and eNOS
It has been shown that ROS formation lead to a reduction of AKT and ERK1/2 phosphorylation in aging endothelial cells, which in turn either regulates telomerase activity or NO production (CitationBreitschopf et al., 2001; CitationYoon et al., 2002; CitationHaendeler et al., 2003; CitationKurz et al., 2004). Here, we examined whether or not ICA can activate the downstream regulators of ROS including AKT and ERK1/2. As shown in , ICA induced rapid AKT phosporylation after 30-min incubation in HUVECs, maximum effects were achieved at ~60 min. ERK1/2 phosphorylation was stimulated 10 min later, the maximum effects were achieved at 30–60 min. We further observed the effects of ICA on phosphorylation of AKT, ERK in homocysteine-stimulated HUVECs. We found that homocysteine addition also slightly increased the phosphorylation of ERK. This may be a stress response to higher concentration of homocysteine. After treatment by ICA, the phosphorylation of AKT, ERK were both significantly enhanced (). ICA did not affect the total protein expression of AKT, ERK.
Figure 5. Icariin (ICA) induced phosphorylation of ERK, AKT, eNOS in human umbilical vein endothelial cells (HUVECs). (A) HUVECs were incubated with 5 μM ICA for 10, 30, 60, 120 min, respectively, phosphorylated level of ERK, AKT, and eNOS was determined by western blot analysis. (B) HUVECs were incubated with 400 μM homocysteine in the presence of 5 μM ICA or not. The phosphorylated levels of ERK, AKT, and eNOS were determined by western blot.
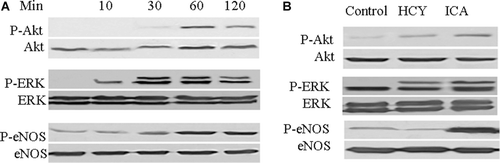
In normal conditions, HUVECs expressed very little phosphorylated eNOS. Homocysteine did not significantly change the expression of the phosphorylated eNOS (). Both in HUVECs and in homocysteine-stimulated HUVECs, ICA increased the protein expression of phosphorylated eNOS ().
ICA partially restored the NO production in homocysteine-treated cells through the PI3K/AKT pathway
In homocysteine-treated HUVECs, the culture medium was harvested and total nitrite was measured. Homocysteine treatment significantly decreased the nitrite content in culture medium. Thirty minutes later, after ICA was added, the nitrite level was increased in a dose-dependent manner with a maximum effects achieved at 5 μM. However, the maximum effects of ICA did not reach the level in control. The presence of PI3K inhibitor wortmannin abolished most effects of ICA on NO production, while the MEK inhibitor PD98059 did not show this ability (). These results suggested that effects of ICA on NO production mainly involved PI3K/AKT-eNOS signaling pathways.
Figure 6. Icariin (ICA) increased nitric oxide (NO) production in human umbilical vein endothelial cells (HUVECs) mainly through PI3K/AKT signaling. HUVECs were incubated with 400 μM homocysteine in the presence or absence of ICA, and NO content in the supernatant was measured. HUVECs were incubated with 400 μM homocysteine and pretreated with PI3K inhibitor (100 nM wortmanin), MEK inhibitor (10 μM, PD98059) for 30 min before exposure to ICA (5 μM), the NO content in supernatant was measured. *p < 0.05 vs. control; **p < 0.01 vs. control; ##p < 0.01 vs. HCY.
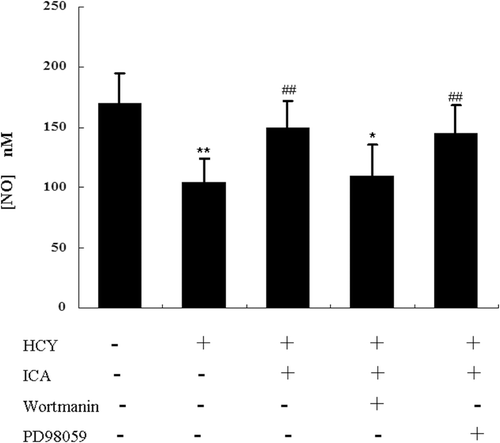
Discussion
SA-β-gal was the most commonly used marker for cellular senescence (CitationItahana et al., 2007). In this study, we performed this assay and showed that a high level of homocysteine resulted in a significant increase in the percentage of SA-β-gal staining cells. After ICA treatment, the percentage declined. Another indicator commonly used for evaluating cellular senescence is CPDs, which examine the effects of ICA on long-term proliferation of HUVECs. Results indicated that homocysteine decreased CPDs, which was partially improved by the ICA treatment. Taken together, our findings above showed that ICA has the ability to delay cellular senescence of HUVECs in vitro. Next, we established an in vivo model to detect if ICA delays or counteracts the homocysteine-induced vascular endothelial cellular senescence. After a 42-day oral administration of homocysteine, rat aortic artery was taken to examine with SA-β-gal staining assay. We found that homocysteine induced significant SA-β-gal staining in the luminal surface of rat aortic artery. After treatment by ICA, much less mean density of SA-β-gal staining was observed. Our results in vitro and in vivo indicated that ICA delayed endothelial cellular senescence, which suggested a possible beneficial role of ICA in the prevention of homocysteine-induced vascular disorders.
Cells eventually enter into a irreversible growth arrest after extended propagation in culture, although these cells are viable and metabolically active. This state is termed replicative senescence which usually requires weeks or months of culture. This cellular senescence is associated with the loss of telomeres (Citationvon Figura et al., 2009). In addition, a diversity of stressors elicits a similar growth arrest within just a few days, referred to as stress-induced premature senescence (CitationAndreassi, 2008; CitationGoligorsky et al., 2009). Under normal conditions, endothelial cells rarely divide with a turnover rate of approximately once every 3 years (CitationForeman & Tang, 2003). Thus, endothelial cellular senescence in vivo might be triggered mainly by various stressors, although the telomere-dependent replicative senescence perhaps also plays a role (CitationFarhat et al., 2008). Among identified stressors, the free-radical theory proposed that the aging cell and aging organism is largely the result of the cumulative effects of ROS, and was widely accepted (CitationLu & Finkel, 2008). In the current study, homocysteine significantly increased ROS levels. ICA reduced the level of homocysteine-induced ROS, which is in accordance with the previous report that ICA had an antioxidant effect (CitationWang & Huang, 2005). These results suggest that the protective effect of ICA on homocyteine-induced endothelial senescence is partially due to its antioxidant action.
ROS have also been recognized as molecules with important functions and as second messengers in cell signaling, being produced in response to external stimuli and subsequently used for signal amplification (CitationDröge, 2002). ROS can directly activate signaling molecules via modification, or indirectly activate signaling cascades by inhibiting protein tyrosine phosphatases. Mitogen-activated protein kinases (MAPKs) are a family of protein-serine/threonine kinases that include at least three distinctly regulated subgroups in mammals: extracellular signal-regulated kinase (ERK), Jun amino-terminal kinase (JNK), and p38 MAPK (CitationChang & Karin, 2001). MAPKs were regulated by MAPK kinases, also called MEKs. Serine-threonine protein kinase AKT (PKB), another critical protein kinase, usually plays as an important survival signal. Studies have established that ROS acted as an upstream regulator of both MAPKs and AKT. ROS can activate MAPKs and inhibit AKT (CitationBreitschopf et al., 2001; CitationYoon et al., 2002; CitationHaendeler et al., 2003; CitationKurz et al., 2004). Subsequently, MAPKs and/or AKT are involved in endothelial cellular senescence through regulation of various downstream targets (CitationShan et al., 2008). In the present study, results showed that homocysteine indicated no significant effects on phosphorylation of ERK and AKT. Pretreatment by ICA induced significantly activation of ERK and AKT. There are reports that indicated ERK was involved in H2O2-induced cell response (CitationHu et al., 2005). In the present study, the concentration of homocysteine was up to 400 μM, thus we deduced that the increase of phosphorylation of ERK was a result of stress response. ICA treatment in the absence of homocysteine elevated the phosphorylation of ERK and AKT. In the presence of homocysteine, ICA also significantly enhanced the phosphorylation of ERK and AKT.
Next, we measured which in ERK and AKT was responsible for the action of ICA. eNOS is an important downstream target of AKT. Activated eNOS enhanced NO production, which has been proven to be closely associated with endothelial senescence (CitationVasa et al., 2000; CitationHayashi et al., 2008). In the present study, we found ICA can increase the phosphorylation of eNOS and production of NO. Furthermore, we detected whether or not ERK or AKT-specific inhibitor can abolish the effects of ICA on NO production. Results showed that ICA activated the eNOS phosphorylation. The PI3K inhibitor wortmannin abolished most effects of ICA on NO production, while the MEK inhibitor PD98059 did not show this ability. Thus, results suggested PI3K/AKT is largely responsible for the effects of ICA on delaying endothelial cellular senescence.
In summary, the present data demonstrate that ICA delays homocyteine-induced endothelial cellular senescence in vitro and in vivo possibly through decreasing ROS level, followed by activation of PI3K/AKT-eNOS signaling pathways. ICA can decrease the ROS level, and activate the PI3K/AKT signaling, rather than ERK signaling, to delay endothelial cellular senescence. Our results indicated that ICA should be considered as a potential therapeutic drug for the prevention of homocysteine-induced cardiovascular disorders by delaying homocsyteine-induced endothelial cellular senescence.
Declaration of interest
This work was supported by National Natural Science Foundation of China (No. 30901888). The authors report no conflicts of interest. The authors alone are responsible for the content and writing of the paper.
References
- Andreassi MG. (2008). DNA damage, vascular senescence and atherosclerosis. J Mol Med, 86, 1033–1043.
- Austin RC, Lentz SR, Werstuck GH. (2004). Role of hyperhomoc-ysteinemia in endothelial dysfunction and atherothrombotic disease. Cell Death Differ, 11 Suppl 1, S56–S64.
- Breitschopf K, Zeiher AM, Dimmeler S. (2001). Pro-atherogenic factors induce telomerase inactivation in endothelial cells through an Akt-dependent mechanism. FEBS Lett, 493, 21–25.
- Chang L, Karin M. (2001). Mammalian MAP kinase signalling cascades. Nature, 410, 37–40.
- Chen J, Goligorsky MS. (2006). Premature senescence of endothelial cells: Methusaleh’s dilemma. Am J Physiol Heart Circ Physiol, 290, H1729–H1739.
- Chung BH, Kim JD, Kim CK, Kim JH, Kim JH, Won MH, Lee HS, Dong MS, Ha KS, Kwon YG, Kim YM. (2008). Icariin stimulates angiogenesis by activating the MEK/ERK- and PI3K/Akt/eNOS-dependent signal pathways in human endothelial cells. Biochem Biophys Res Commun, 376, 404–408.
- Clarke R, Daly L, Robinson K, Naughten E, Cahalane S, Fowler B, Graham I. (1991). Hyperhomocysteinemia: An independent risk factor for vascular disease. N Engl J Med, 324, 1149–1155.
- Dröge W. (2002). Free radicals in the physiological control of cell function. Physiol Rev, 82, 47–95.
- Erusalimsky JD. (2009). Vascular endothelial senescence: From mechanisms to pathophysiology. J Appl Physiol, 106, 326–332.
- Farhat N, Thorin-Trescases N, Voghel G, Villeneuve L, Mamarbachi M, Perrault LP, Carrier M, Thorin E. (2008). Stress-induced senescence predominates in endothelial cells isolated from atherosclerotic chronic smokers. Can J Physiol Pharmacol, 86, 761–769.
- Fenton M, Barker S, Kurz DJ, Erusalimsky JD. (2001). Cellular senescence after single and repeated balloon catheter denudations of rabbit carotid arteries. Arterioscler Thromb Vasc Biol, 21, 220–226.
- Foreman KE, Tang J. (2003). Molecular mechanisms of replicative senescence in endothelial cells. Exp Gerontol, 38, 1251–1257.
- Goligorsky MS, Chen J, Patschan S. (2009). Stress-induced premature senescence of endothelial cells: A perilous state between recovery and point of no return. Curr Opin Hematol, 16, 215–219.
- Haendeler J, Hoffmann J, Brandes RP, Zeiher AM, Dimmeler S. (2003). Hydrogen peroxide triggers nuclear export of telomerase reverse transcriptase via Src kinase family-dependent phosphorylation of tyrosine 707. Mol Cell Biol, 23, 4598–4610.
- Hayashi T, Yano K, Matsui-Hirai H, Yokoo H, Hattori Y, Iguchi A. (2008). Nitric oxide and endothelial cellular senescence. Pharmacol Ther, 120, 333–339.
- Hu Y, Wang X, Zeng L, Cai DY, Sabapathy K, Goff SP, Firpo EJ, Li B. (2005). ERK phosphorylates p66shcA on Ser36 and subsequently regulates p27kip1 expression via the Akt-FOXO3a pathway: Implication of p27kip1 in cell response to oxidative stress. Mol Biol Cell, 16, 3705–3718.
- Itahana K, Campisi J, Dimri GP. (2007). Methods to detect biomarkers of cellular senescence: the senescence-associated beta-galactosidase assay. Methods Mol Biol, 371, 21–31.
- Kurz DJ, Decary S, Hong Y, Trivier E, Akhmedov A, Erusalimsky JD. (2004). Chronic oxidative stress compromises telomere integrity and accelerates the onset of senescence in human endothelial cells. J Cell Sci, 117, 2417–2426.
- Lawrence de Koning AB, Werstuck GH, Zhou J, Austin RC. (2003). Hyperhomocysteinemia and its role in the development of atherosclerosis Clin Biochem, 36, 431–441.
- Lu T, Finkel T. (2008). Free radicals and senescence. Exp Cell Res, 314, 1918–1922.
- Minamino T, Miyauchi H, Yoshida T, Ishida Y, Yoshida H, Komuro I. (2002). Endothelial cell senescence in human atherosclerosis: role of telomere in endothelial dysfunction. Circulation, 105, 1541–1544.
- Perez-de-Arce K, Foncea R, Leighton F. (2005). Reactive oxygen species mediates homocysteine-induced mitochondrial biogenesis in human endothelial cells: Modulation by antioxidants. Biochem Biophys Res Commun, 338, 1103–1109.
- Peshavariya HM, Dusting GJ, Selemidis S. (2007). Analysis of dihydroethidium fluorescence for the detection of intracellular and extracellular superoxide produced by NADPH oxidase. Free Radic Res, 41, 699–712.
- Shan H, Bai X, Chen X. (2008). Angiotensin II induces endothelial cell senescence via the activation of mitogen-activated protein kinases. Cell Biochem Funct, 26, 459–466.
- Song YH, Li BS, Chen XM, Cai H. (2008). Ethanol extract from Epimedium brevicornum attenuates left ventricular dysfunction and cardiac remodeling through down-regulating matrix metalloproteinase-2 and -9 activity and myocardial apoptosis in rats with congestive heart failure. Int J Mol Med, 21, 117–124.
- Vasa M, Breitschopf K, Zeiher AM, Dimmeler S. (2000). Nitric oxide activates telomerase and delays endothelial cell senescence. Circ Res, 87, 540–542.
- von Figura G, Hartmann D, Song Z, Rudolph KL. (2009). Role of telomere dysfunction in aging and its detection by biomarkers. J Mol Med, 87, 1165–1171.
- Wang YK, Huang ZQ. (2005). Protective effects of icariin on human umbilical vein endothelial cell injury induced by H2O2 in vitro. Pharmacol Res, 52, 174–182.
- Xu D, Neville R, Finkel T. (2000). Homocysteine accelerates endothelial cell senescence. FEBS Lett, 470, 20–24.
- Xu HB, Huang ZQ. (2007). Vasorelaxant effects of icariin on isolated canine coronary artery. J Cardiovasc Pharmacol, 49, 207–213.
- Yap SP, Shen P, Li J, Lee LS, Yong EL. (2007). Molecular and pharmacodynamic properties of estrogenic extracts from the traditional Chinese medicinal herb, Epimedium. J Ethnopharmacol, 113, 218–224.
- Yoon SO, Yun CH, Chung AS. (2002). Dose effect of oxidative stress on signal transduction in aging. Mech Ageing Dev, 123, 1597–1604.
- Zhang G, Qin L, Sheng H, Yeung KW, Yeung HY, Cheung WH, Griffith J, Chan CW, Lee KM, Leung KS. (2007). Epimedium-derived phytoestrogen exert beneficial effect on preventing steroid-associated osteonecrosis in rabbits with inhibition of both thrombosis and lipid-deposition. Bone, 40, 685–692.
- Zhou H, Wang W, Luo Y. (2005). Contributions of disulfide bonds in a nested pattern to the structure, stability, and biological functions of endostatin. J Biol Chem, 280, 11303–11312.
- Zhu JH, Chen JZ, Wang XX, Xie XD, Sun J, Zhang FR. (2006). Homocysteine accelerates senescence and reduces proliferation of endothelial progenitor cells. J Mol Cell Cardiol, 40, 648–652.