Abstract
Context: The filtrate of Staphylococcus aureus culture, named staphylococcal enterotoxin C injection, has been used for 10 years in China. SEC2 has been claimed to be the only staphylococcal enterotoxins (SEs) without certifiable evidence.
Objectives: To present an efficient procedure for the expression and purification of recombinant proteins SEG and SEI, from S. aureus.
Materials and methods: In present work, we extracted total DNA from S. aureus (FRI 1230) and the recombinant proteins of SEG and SEI were then cloned, expressed and purified using E. coli. Splenic lymphocytes were used as effector cells and K562 and B16 cells were used as target cells to evaluate the inhibitory and stimulatory abilities of purified rSEG and rSEI on in vitro proliferation.
Results: The size of amplified products of SEG and SEI genes were found to be about 400 and 467 bp, respectively. pGEX-SEG and pGEX-SEI were constructed successfully. SEG and SEI were demonstrated to be active stimulators of T-cell proliferation; moreover, they inhibited the proliferation of K562 cells and B16 cells.
Discussion: The current findings suggest that SEC2 might not be the only active component of staphylococcal enterotoxin C injection and may involve other essential proteins like SEG and SEI in its clinical efficacy.
Conclusion: This efficient procedure for the expression and purification of SEG and SEI and may be useful for mass production of therapeutically important proteins. In the future, proteins acting as active stimulators of T-cell proliferation may help in developing effective cancer therapy.
Introduction
Staphylococcal enterotoxins (SEs), 26 to 30 kDa proteins, are produced by Staphylococcus aureus (S. aureus) and have been considered responsible for most common cases of human food poisoning. Five major serological types of enterotoxins, SEA, SEB, SEC, SED and SEE, have been characterized. Moreover, SEG through SEQ have been recently identified. They are known to share a number of genetic and biochemical characteristics, with similar functions (Dings et al., Citation2000; Stiles et al., Citation1993). Their ability to stimulate lymphocyte proliferation and lymphokine production even at low concentrations (10−13–10−16 M) account for making them potent T-cell activators. Moreover, their ability of inducing apoptotic death of tumor cells is of particular interest (Hansson et al., Citation1997; Jie et al., Citation2007; Ulrich, Citation2006). Gaojushen was prepared and processed from the filtrate of S. aureus culture and, after a phase III clinical trial in China, it was licensed for marketing in 1996. (Chen, Citation2005).
Ampuls of staphylococcal enterotoxin C injection, a filtrate of Staphylococcus aureus culture, have been used for ten years in China as cancer therapy with proved efficacy (Chen, Citation2001). However, the active components responsible for the therapeutic effects have not been elucidated. Our previous investigation demonstrated that SEC2, SEM and SEI genes existed in the chromosome of S. aureus (FRI 1230) which is the productive bacterial strain of staphylococcal enterotoxin C injection (Ding et al., Citation2009; Morandi et al., Citation2007; Pan et al., Citation2007; Xue et al., Citation2006). Non-classical SEs include SEG, SEI, SEK, SEM, SEN, SEO and SEQ. In our previous work (Pan et al., Citation2007; Xue et al., Citation2006) we have demonstrated the bioactivity of SEM, SEN, SEK and SEQ. In the present study we have attempted to clone and express other genes of SEs, namely SEG and SEI. Further, we have detected their biological activity.
Materials and methods
Cell lines and culture
K562 (human chronic myelogenous leukemia cell line, ATCC CCL 243) and B16 (murine melanoma cell line, ATCC CRL-6322), Standard procedures were used for cell culture.
Animals
ICR mice (20 ± 2 g) were provided by the Animal Research Center, Academy of Medical Science, Zhejiang province, China. The animals were housed in a 24 h light-dark cycle controlled room with a temperature and humidity of 25 ± 2 and 50%, respectively. All animal experiments were conducted in accordance with the accepted standards for animal care approved by laboratory of animal centre, Zhejiang University Hangzhou, China. For the mouse cell assay, splenocytes were obtained from the spleen of ICR mice by the method described previously (Hosoya et al., Citation2002). Briefly, mouse spleen was ground with two glass slides and the fragments were sieved by passing through 70 mm-nylon mesh. Finally the cells were centrifuged at 15 000 × g at RT for half an hour. The cells were then re-suspended (90% RPMI1640 with 10% FBS) for further analysis.
Bacterial strains, plasmids, enzymes, reagents and medium
Staphylococcus aureus (FRI 1230) was used for the extraction of the total DNA. Escherichia coli (E. coli) strain DH5α was used for the transformation of the vectors. E. coli strain BL21 (DE3) was applied as host strain for expression. The plasmids pGEM-T and pGEX-4T-1 (Promega BioSciences, Madison, WI) were used to sequence and express SEG and SEI. The restriction endonucleases, rTaq polymerase, T4 DNA ligase, MTT, ConA and DNA gel extraction kit were purchased from Shanghai Sangon Biotechnology (Shanghai, China). DNA sequencings were completed by Shanghai Sangon Biotechnology. IPTG were purchased from BBI (USA). Glutathione Sepharose 4B was from Amersham Pharmacia Biotech (Uppsala, Sweden). BCA kit was from Beyotime (Jiangsu, China).
DNA extraction and PCR amplification
Total DNA was extracted from S. aureus with protease K and SDS-Tris solution and purified with saturated phenol by centrifugation as described previously (Pan et al., Citation2007). Initial denaturation condition for PCR was set at 94 °C for 5 min, followed by 25 cycles of denaturation at 94 °C for 30 s, annealing at 52 °C for 60 s, extension at 72 °C for 60 s, and a final extension at 72 °C for 10 min. The mature peptide was amplified with the following primer pairs: SEG (GTAGGATCCCAACCCGATCCTAAATTAGAC, GGACTCGAG, TCAGTGAGTATTAAGAAATAC); SEI: (GTAGGATCCCAAGGT GATATTGGTGTAGGT, GGACTCGAGTTAGTTACTATCTACATATGA). All primers were synthesized in Shanghai Sangon Biotechnology.
Construction and sequencing analysis of pGEM-SEG and pGEM-SEI
After recovery of the amplified PCR products, the products were cloned into pGEM-T vectors and the plasmids were transformed to DH5α competent cells. The transformants were cultured in a LB plate with IPTG/X-gal and ampicillin, and white clones were selected. The recombinant plasmids with SEG or SEI genes were identified with restriction endonucleases cleavage and confirmed by sequencing analysis. The sequences of the inserts were compared to DNA sequences of standard sequence of SEG and SEI in PubMed and confirmed to be right.
Construction and verification of rSEG and rSEI expression vectors
The recombinant plasmids pGEM-SEG and pGEX-4T-1 were cleavaged with BamH I and Xho I. The cleavaged products of mature peptide of SEG with sites of restriction endonucleases and the products of the fragment of plasmid pGEX-4T-1 were recovered and connected to construct the expression vector of GST-SEG, named pGEX-SEG. The resultant expression vector plasmids were transformed to competent cells and the amplification products were identified with restriction endonucleases. In addition, the expression vector of rSEI was constructed and verified in the same way.
Expression and purification of rSEG and rSEI in E. coli.
Transformed pGEX-SEG into E. coli BL21, the transformants bearing plasmid pGEX-SEG were inoculated in 5 ml LB culture medium containing ampicillin and shaking cultured for 6 h. The expression strains were inoculated in 2 × YT culture medium with dilution of 1:100 and shaking cultured at 37 °C. When grown to OD600 = 0.8, the expression strains were induced with 1 nM IPTG at 30 °Cfor 5 h, centrifuged at 8000 rpm for 10 min at 4 °C and pellets were collected. Each milliliter culture was suspended with 50 μl ice-cold PBS containing 0.5% Triton X-100 and processed with a FRENCH press (Thermo Spectronic Instruments, Inc., Rochester, NY). The supernatant was collected after centrifugation (12 000 rpm, 30 min) and preserved at 4 °C for further purification. The supernatant and sediment were detected directly by SDS-PAGE to observe the water solubility of rSEG.
The GST-4B gel column was equilibrated with PBS. Elution was performed using the reduced glutathione buffer (0.05 M Tris-HCl, 0.05 M glutathione, pH 8.0). Gene Quant pro was used to detect the elution of rSEG to OD280 < 0.1. The purity and concentration of collected compositions of proteins were detected by SDS-PAGE. In addition, rSEI and GST were expressed, purified and detected the same way.
Bioactivity analysis of rSEG and rSEI in vitro
The abilities of purified rSEG and rSEI to stimulate T-cells were detected as follows: our method involves isolating lymphocytes from ICR mouse spleen, after getting rid of the red blood cells, placing 5 × 104 cells/well in a 96-well plate with or without various stimuli with four parallel wells. Allow the cells to proliferate for 2 d at 37 °C. The concentrations of various stimuli, such as ConA, GST, rSEG and rSEI, were detected with a BCA kit. The amount of proliferation was detected on the 44 h by adding 15μl PBS containing MTT (0.5 mg/ml) to each well and incubating for another 4 h. The formazan crystals were dissolved in 120 μl DMSO and the absorbance was measured at A570nm with an ELISA reader. Absorbance values for wells containing medium alone were subtracted from the results of the test wells. These data were presented as means of SD from four independent experiments and statistically analyzed by Student’s t-test.
Then, the abilities of purified rSEG and rSEI of inhibiting the proliferation of tumor cells were observed. Splenic lymphocytes were used as effector cells and K562 and B16 cells were used as target cells. The cell population of splenic lymphocyte was 5 × 104 cells/well and K562 and B16 cells were 2.5 × 105/well. Inhibition of proliferation of K562/B16 cells as a consequence of treatment with the target recombinant proteins was evaluated using the MTT assay described previously. Inhibition of K562/B16 (%) was calculated with the equation: 100- [(growth inhibition of K562/B16-lympholeukocyte background discharge)/tumor cell control] × 100, and analyzed with graphs. These data were presented as means ± SD from four independent experiments and statistically analyzed by Student’s t-test.
Results
Cloning and sequencing of SEG and SEI genes
After clean-up and ligation reaction, the SEG and SEI genes were amplified by PCR from the DNA of the production strain and then inserted into pGEM-T vector. Sequence analysis of single clone showed that the main band obtained followed complete encoding sequence of SEG or SEI genes PCR amplification of SEG and SEI gene and verification of positive clone with pGEM-T-SEG and pGEM-T-SEI by double RE digestion is shown in . The results shown in depict the size of amplified products of SEG and SEI genes to be about 400 bp and 467 bp, respectively. Two additional restriction endonuclease sites BamH I and Xho I had been introduced at the 5′ and 3′ ends of the DNA encoding of SEG and SEI, respectively.
Figure 1. PCR amplification of SEG and SEI gene and verification of positive clone with pGEM-T-SEG and pGEM-T-SEI by double RE digestion. M: DNA marker; 1: PCR product of SEI; 2: result of double RE digestion of positive clone with pGEM-T-SEI; 3: result of double RE digestion of positive clone with pGEM-T-SEG. 4: result of double RE digestion of negative clone with pGEM-T-SEG; 5: PCR products of SEG.
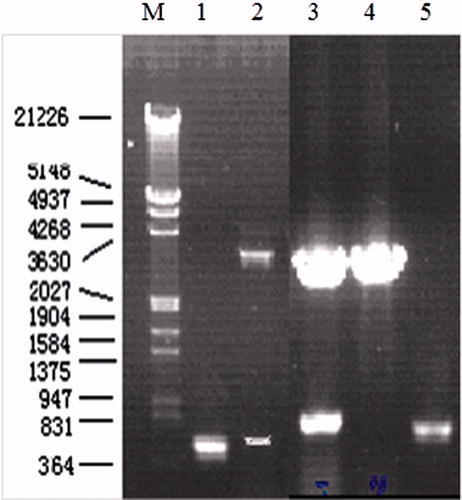
Construction and verification of fusion expression vectors
pGEX-SEG and pGEX-SEI were constructed successfully and the verification of positive clone with pGEX-SEG and pGEX-SEI by double RE digestion is shown in . Two additional restriction endonuclease sites BamH I and Xho I had been introduced at the 5′ and 3′ ends of the DNA encoding of SEG and SEI, respectively, for the verification of the constructs.
Expression and solubility of recombinant protein rSEG and rSEI
The recombinant expression vectors (pGEX-SEG and pGEX-SEI) were transformed into E. coli BL21 for the expression of fusion proteins. The expression conditions were adjusted on the basis of the yield of target and typical fusion proteins. E. coli BL21 strain harboring pGEX-SEG and pGEX-SEI expressed high-level of target fusion proteins. Molecular weights of the dominant bands (55 kDa) were in accordance with the expected typical fusion protein, while the dominant bands were observed to be absent in uninduced control E. coli BL21 strain. The recombinant proteins were purified by affinity chromatography. Satisfactory water solubility without observed inclusion bodies and purified fusion proteins was observed via GS-4B gel column for both recombinant rSEG and rSEI. The expression pattern and purified result of protein is shown in .
Figure 3. Expression and purified protein of recombinant SEG and SEI pattern and recombinant IL-24 protein purified from affinity chromatography. (A) 1: supernatant of the induced BL21 with plasmid pGEX-SEG after disrupting by sonication; 2: supernatant of induced BL21 with plasmid pGEX-SEG; 3,4: purified protein of rSEG; 5: Marker. (B) 1: supernatant of the induced BL21 with plasmid pGEX-SEI after disrupting by sonication; 2: supernatant of non-induced BL21 with plasmid pGEX-SEI; 3,4,6:purified protein of rSEI; 5: Marker.
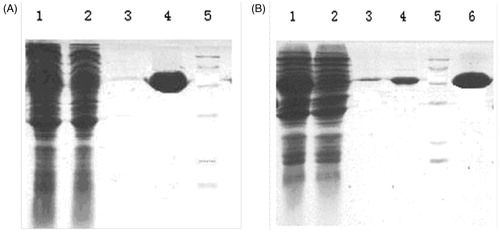
Bioactivity analysis of rSEG and rSEI in vitro
The stimulatory and inhibitory effects rSEG and rSEI on proliferation of splenic lymphocytes were evaluated by the MTT assay. ConA and GST served as positive and negative control, respectively. According to the results of MTT, negative control (GST, 10 μg/ml) when was compared with the splenic lymphocytes of ICR mice, the results indicated that after 48 h the growth of cells was significantly inhibited by incubation with the splenic lymphocytes that were stimulated with rSEG or rSEI at serial concentrations (0.01, 0.1 and 1 μg/ml) as compared with the negative control (). The inhibitory effects of rSEG or rSEI splenic lymphocytes on proliferation of K562 and B16 cells were also examined by the MTT assay. as shown in and .
Figure 4. Effects of rSEG and rSEI on multiplication exponential of mouse lymphocytes by the MTT assay. Compared with the negative control group: ***p < 0.001, **p < 0.01, *p < 0.05.
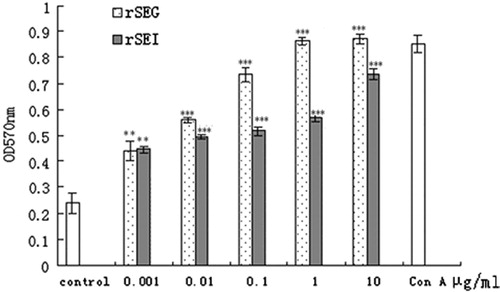
Discussion
Over the past several years, investigational studies have greatly contributed in determining new therapeutic targets and drug delivery strategies for the treatment of fatal diseases like cancer (Lane et al., Citation2011; Luo et al., Citation2009; Patyar et al., Citation2010; Shaheen et al., Citation2011) and diabetes (Akash et al., Citation2013a, Citation2013b) which have complicated pathogenesis including generation of oxidative stress and various inflammatory responses (Akash et al., Citation2012, Citation2013c; Brown, Citation2000). Despite this progress, there is a great need regarding the drugs used commonly for the treatment of various diseases to be evaluated for their possible toxic effects (Rehman et al., Citation2012; Saleem et al., Citation2012) and biodisposition kinetics (Parveen et al., Citation2012).
Owing to their ability to stimulate T-cells, SEs have attained considerable attention for being an ideal approach for cancer treatment. For a decade, the filtrate of S. aureus culture has been used as therapy for cancer in China. However, SEC2 was declared to be the only active component. Lou et al. (Citation2007) isolated SEC2 from staphylococcal enterotoxin C injection (GaojunshengR) and detected the concentration of SEC2 by ELISA. However, as concluded by our previous work (Xue et al., Citation2006), participation of SEC2 in SEC injection had been verified in cancer therapy, and the presence and contribution of other active component present should also be taken into account. Therefore, recently we isolated the genes SEM and SEN, in the same bacterial strain, and confirmed the biological activities of recombinant proteins (Ding et al., Citation2011; Pan et al., Citation2007).
Further, considered that genes of the enterotoxins (SEG, SEI, SEM, SEN and SEO) are physically clustered in the S. aureus genome (Van Belkum et al., Citation2006), in the current investigation we have tried to express the proteins of SEG and SEI and detected the biological activities of these two SEs. The results of amplified fragments of SEG (400 bp) and SEI (467 bp) obtained as indicated in were observed to be in accordance with the results anticipated. Further, the verification of a positive clone was done with pGEX-SEG and pGEX-SEI by double RE digestion () and the verifications were also found to be in accordance with the results anticipated. Likewise, the appearance of dominant bands only in the E. coli BL21 strain harboring pGEX-SEG and pGEX-SEI, as compared to the uninduced control E. coli BL21 strain, were also in accordance with the expected fusion proteins. Moreover, bioactivity analysis of rSEG and rSEI in vitro was done by evaluating the effects of rSEG and rSEI on multiplication exponential of mouse lymphocytes by the MTT assay. This analysis elucidated that after 48 h, splenic lymphocytes of ICR mice significantly increased with rSEG or rSEI (1 μg/ml) (p < 0.01) as shown in . Similarly, the results shown in and clearly indicated the significant growth inhibition of K562 and B16 cells by various concentrations of rSEG or rSEI. However, the expression of different SEs may be affected by culture conditions affecting the ratio of SEs in S. aureus culture. In this research, we successfully cloned, expressed and purified recombinant proteins rSEG and rSEI, and evaluated their bioactivities. However, we did not confirm the expression of SEG and SEI in S. aureus culture. It should be noted that this study has only examined the bioactivities of rSEG and rSEI and, therefore, more investigational studies are required to reveal the existent active components of the filtrate of S. aureus culture responsible for therapeutic efficacy.
Conclusion and future perspective
To conclude, the present results propose that SEC2 might not be the only active constituent of staphylococcal enterotoxin C injection and may involve other essential proteins such as SEG and SEI in its proven effectiveness. This study presents an efficient approach for the expression and purification of recombinant proteins, and may be constructive for mass production of therapeutically vital proteins. SEC cooperated with other components, such as SEM, SEN, SEG, SEI and other SEs produced by S. aureus, may also be estimated as an effective treatment for tumors. Such approaches might be helpful to improve clinical effects with controllable side effects and improved efficacy. Thereby, efficient procedures for the expression and purification of proteins may be useful for the mass productions of these proteins and development of novel therapeutically important protein drugs. Furthermore, the proteins that act as active stimulators of T-cell proliferation may well assist in developing effectual therapeutics against carcinogenesis by provoking production of various cytokines resulting in potent cell-mediated immunological responses and tumor cell apoptosis. In the future, single or multiple pure SEs depicting minimal side effects and greater clinical efficacy might be used as an ideal treatment strategy for cancer therapy.
Declaration of interest
The authors declare they do not have any conflict of interest for this article. This project was financially supported by a grant (No. 2010C13006) from the Science and Technology Department of Zhejiang Province, China.
References
- Akash MSH, Rehman K, Chen S. (2013b). Role of inflammatory mechanisms in pathogenesis of type 2 diabetes mellitus. J Cell Biochem 114:525–31
- Akash MSH, Rehman K, Gillani Z, et al. (2013c). Cross-species comparison of IL-1Ra sequence between human and rat. J Proteomics Bioinform 6:038–42
- Akash MSH, Rehman K, Sun H, Chen S. (2013a). Interleukin-1 receptor antagonist improves normoglycemia and insulin sensitivity in diabetic Goto-Kakizaki-rats. Eur J Pharmacol 701:87–95
- Akash MSH, Shen Q, Rehman K, Chen S. (2012). Interleukin-1 receptor antagonist: A new therapy for type 2 diabetes mellitus. J Pharm Sci 101:1647–58
- Brown JM. (2000). Exploiting the hypoxic cancer cell: Mechanisms and therapeutic strategies. Mol Med Today 6:157–62
- Chen TZ. (2001). The exploitation of HAS and its application in tumor therapy. Prog Microbiol Immunol 29:63–9
- Chen, TZ. (2005). Gaojushen: A novel anti-cancer drug prepared from SEC superantigen. Prog Microbiol Immunol 33:49–50
- Ding D, Huang P, Pan Y, Chen S. (2011). Gene detection of staphylococcal enterotoxins in production strain of staphylococcin injection and superantigenic activity of rSEK and rSEQ. World J Microbiol Biotechnol 27:2957–67
- Ding D, Huang P, Sun HY, et al. (2009). Identification of protein components and quantitative immunoassay for SEC2 in staphylococcin injection. J Pharmaceut Biomed Anal 50:79–85
- Dinges MM, Orwin PM, Schlievert PM. (2000). Exotoxins of Staphylococcus aureus. Clin Microbiol 13:16–34
- Hansson J, Ohlsson L, Persson R, et al. (1997). Genetically engineered superantigens as tolerable antitumor agents. Proc Natl Acad Sci USA 94:2489–94
- Hosoya K, Takashima T, Matsuda K, Terasaki T. (2002). Spleen lymphocyte kinetics in mice under normal and inflammatory conditions: An application of the transgenic mouse expressing beta-galactosidase (ROSA 26). Biol Pharm Bull 25:1378–80
- Jie KG, Jiang H, Sun L, et al. (2007). The pilot study of anti-tumor effects versus immunosuppression of Staphylococcal enterotoxin C. Cancer Biol Ther 6:1584–91
- Lane DP, Brown CJ, Verma C, Cheok CF. (2011). New insights into p53 based therapy. Discov Med 12:107–17
- Luo J, Solimini NL, Elledge SJ. (2009). Principles of cancer therapy: Oncogene and non-oncogene addiction. Cell 136:823–37
- Luo P, Lv HX, Zang SM. (2007). Preparation and preliminary application of monoclonal antibody against Staphylococcal enterotoxin C2. Zhongguo Mianyixue Zazhi 23:53–5
- Morandi S, Brasca M, Lodi R, et al. (2007). Detection of classical enterotoxins and identification of entero toxin genes in Staphylococcus aureus from milk and dairy products. Vet Microbiol 124:66–72
- Pan YQ, Ding D, Li DX, Chen SQ. (2007). Expression and bioactivity analysis of Staphylococcal enterotoxin M and N. Protein Expr Purif 56:286–92
- Parveen A, Akash MSH, Rehman K, et al. (2012). Biodisposition kinetics of isoniazid in healthy females. J App Pharm 4:676–81
- Patyar S, Joshi R, Byrav DS, et al. (2010). Bacteria in cancer therapy: A novel experimental strategy. J Biomed Sci 17:21
- Rehman K, Akash MSH, Azhar S, et al. (2012). A biochemical and histopathologic study showing protection and treatment of gentamicin-induced nephrotoxicity in rabbits using vitamin C. Afr J Tradit Complement Altern Med 9:360–5
- Saleem U, Ahmed B, Rehman K, et al. (2012). Nephro-protective effect of vitamin C and Nigella sativa oil on gentamicin associated nephrotoxicity in rabbits. Pak J Pharm Sci 25:727–30
- Shaheen M, Allen C, Nickoloff JA, Hromas R. (2011). Synthetic lethality: Exploiting the addiction of cancer to DNA repair. Blood 117:6074–82
- Stiles BG, Bavari S, Krakauer T, Ulrich RG. (1993). Toxicity of Staphylococcal enterotoxins potentiated by lipopolysaccharide: Major histocompatibility complex class II molecule dependency and cytokine release. Infect Immun 61:5333–8
- Ulrich RG. (2006). Evolving superantigens of Staphylococcus aureus. FEMS Immunol Med Microbiol 27:1–7
- Van Belkum A, Melles DC, Snijders SV, et al. (2006). Clonal distribution and differential occurrence of the enterotoxin gene cluster, egc, in carriage- versus bacteremia-associated isolates of Staphylococcus aureus. J Clin Microbiol 44:1555–7
- Xue Q, Ying YB, Pan YQ, et al. (2006). Expression and bioactivity analysis of Staphylococcal enterotoxin C2. Yao Xue Xue Bao 41:406–11