Abstract
Context: Among the essential amino acids, phenylalanine, tryptophan, and tyrosine are aromatic amino acids which are synthesized by the shikimate pathway in plants and bacteria. Herbicide glyphosate can inhibit the biosynthesis of these amino acids. So, identification of the gene tolerant to glyphosate is very important. It has been shown that the common reed or Phragmites australis Cav. (Poaceae) is relatively tolerant to glyphosate.
Objective: The aim of the current research is identification, cloning, sequencing, and registering of partial aro A gene of the common reed P. australis.
Materials and methods: The partial aro A gene of common reed (P. australis) was cloned in Escherichia coli and the amino acid sequence was identified/determined for the first time.
Results: This is the first report for isolation, cloning, and sequencing of a part of aro A gene from the common reed. A 670 bp fragment including two introns (86 bp and 289 bp) was obtained. The open reading frame (ORF) region in part of gene was encoded for 98 amino acids. Alignment showed high similarity among this region with Zea mays (L.) (Poaceae) (94.6%), Eleusine indica L. Gaertn (Poaceae) (94.2%), and Zoysia japonica Steud. (Poaceae) (94.2%). The alignment of amino acid sequence of the investigated part of the gene showed a homology with aro A from several other plants. This conserved region forms the enzyme active site.
Conclusion: The alignment results of nucleotide and amino acid residues with related sequences showed that there are some differences among them. The relative glyphosate tolerance in the common reed may be related to these differences.
Introduction
Aromatic amino acids are essential for all organisms. 5-Enolpyruvylshikimate-3-phosphate synthase (EPSPS) catalyses the sixth step of the shikimate pathway. This enzyme catalyses transfer of the phosphoenolpyruvate moiety to shikimate-3-phosphate and produces 5-enolpyruvylshikimate-3-phosphate (EPSPS) (Schönbrunn et al., Citation2001). This enzyme is essential for the biosynthesis of the aromatic amino acids (phenylalanine, tyrosine and tyrosine and subsequently lignins, tannins, and other phenylpropanoids) in plants and bacteria (Green, Citation2009; Kahrizi & Salmanian, Citation2008; Yi et al., Citation2007). EPSPS is widely studied because it is the only enzyme that is inhibited by the non-selective broad-spectrum herbicide glyphosate. In contrast, this pathway is absent in mammals, EPSPS serves as an important target for novel antibiotics (Hollander-Czytko & Amrhein, Citation1987; Lewendon & Coggins, Citation1983). Thus, glyphosate can block the production of aromatic amino acid and then lead to organism death. Two classes of EPSPS have been identified. The first class contains enzymes that are naturally glyphosate sensitive. This group was found in plants and bacteria such as Arabidopsis thaliana L. (Brassicaceae), Brassica napus L. (Brassicaceae), and Escherichia coli. The second class involves enzymes that are naturally glyphosate tolerant such as Agrobacterium tumefaciens CP4 and Pseudomonas sp. Strain PG 2982 (Yi et al., Citation2007). EPSPS has been studied in some systems including plants. The aro A gene that encodes EPSPS has been isolated from plants, fungi, and bacteria (Kahrizi et al., Citation2007).
Some reports on behalf of farmers indicate that complete deracination of the common reed or Phragmites australis, (Cav.) Trin. ex Steud. (Poaceae) by means of glyphosate often is not possible (Back & Holomuzki, Citation2008; Marks et al., Citation1994). The common reed is a large perennial grass that is a very aggressive, dense, and robust member of the grass family. It is an American and Eurasia native (Baran et al., Citation2002). Normally the glyphosate-tolerant plants have been reported previously. In 1987, Gasser and coworkers determined the complete nucleotide sequences of aro A cDNAs of Petunia hybrid Vilm. (Solanaceae) and Lycopersicon esculentum Mill. (Solanaceae). They compared these sequences with other sequences from fungi and bacteria sources (Gasser et al., Citation1987). The EPSPS gene of A. thaliana was cloned and sequenced and then fused to CaMV35S to produce glyphosate-tolerant plants (Klee et al., Citation1987). Many mutated sites that can be a cause of the resistance to glyphosate have been reported such as Salmonella typhimurium P101S in the aro A gene (Stalker et al., Citation1985), G96 of E. coli, simultaneous substitution of G96 and A183 of Brassica napus (Kahrizi et al., Citation2007). Although EPSPS has been found in many species, it has never been cloned from a common reed. In this experiment, partial aro A gene of the common reed (P. australis) encoding the enzyme EPSPS was cloned in E. coli and the sequence was identified for the first time.
Materials and methods
Chemicals, enzymes, and kits
All the chemicals employed for the preparation of reagents and kits (T/A cloning kit and plasmid extraction) were procured from Fermentas (Amherst, NY). DNA-free sensitive (DFS) Taq DNA polymerase enzyme was obtained from Bioron (Newtown Square, PA). RNase A and T4 DNA ligase were purchased from Fermentas (Amherst, NY).
Bacteria strain, plasmid, and plant material
Escherichia coli DH5α strain used in the present study was grown in the LB medium at 37 °C with shaking (200 rpm). Plasmid pTZ (from T/A cloning kit, Fermentas, Amherst, NY) was utilized for T/A cloning and sequencing. The common reed collected from the Kermanshah River was used as the experimental plant material.
EPSPS gene amplification
Genomic DNA was isolated from the common reed using the CTAB method (Murray & Thampson, Citation1980). As aro A gene was unknown, a part of aro A gene that is localized between two conserved sequences was amplified by PCR using specific primers TKZF forward 5′CTTCTTGGG GAA TGCTGGAAC 3′ (Tm = 61 °C) and TKZR: 5′AAGAGCCAAAGGAGCAGCCAT 3′ (Tm = 61 °C). These primers were designed by means of the some software Oligo 5 and DNAstar package (National Biosciences, Inc., Chester, NY) based on plants conserved EPSPS sequences especially Poaceae family such as Sorghum bicolor (L.) Moench., Zea mays L., Triticum aestivum L., and Oryza sativa L.
The aro A gene cloning and sequencing
The considerable PCR product (670 bp) was cloned into pTZ plasmid by means of T/A cloning. Plasmid extraction was done from these clones by means of a standard method (Sambrook & Russell, Citation2001). For the molecular analysis to ensure the presence of distinct insert, primary screening on the LBA/X-Gal/IPTG/ampicillin was carried out. Then the white clones and one of the blue clones were selected for further analysis (Sambrook & Russell, Citation2001). The second step was PCR amplification from plasmids with insert and negative control, with specific primers (TKZF and TKZR). Finally, plasmid with authentic insert was sequenced from both directions with M13 universal primers (M13F (-20) and M13RPUC(-40)), using the capillary method with the ABI system (model: 3730XL) at Macrogen Company, Seoul, Korea.
Results and discussion
Cloning of aro A gene of common reed
Genomic DNA that was purified from leaves of common reed showed high quality and quantity (data not shown). As there is no report about aroA gene in P. australis, in this experiment, the genomic DNA was used. The use of cDNA in unknown genes will cause the loss of information about introns numbers and sequence. The partial aro A gene of common reed was amplified using specific primers TKZF and TKZR from genomic DNA () and a 670 bp fragment was cloned into pTZ plasmid by means of the T/A cloning method.
Analysis of the cloned gene
Some analyses were carried out to find the plasmid with a distinct insert. The first step was white/blue screening in the LBA/X-Gal/IPTG/amp medium. In the second step, plasmids extracted from putative clones were confirmed by PCR-specific primers (TKZF and TKZR). Electrophoresis of the above PCR product showed a band of about 670 bp in length (). Last, it was confirmed by sequencing. The partial sequence of the common reed aro A gene (670 bp) was determined (). For further analysis, the sequenced fragment was submitted to the Blast server for alignment. The alignment of partial sequence belonged to EPSP synthase and gave almost 30 similar sequences showing similarity between P. australis sequence and Eleusine indica (epsps-R). According to this result, we selected nine sequences with high similarity for the next analysis (). As the fragment was isolated from genomic DNA, therefore, this sequence may contain exon and intron fragments. The multiple alignments of sequenced fragment with selected sequences () were performed by Megalign software (DNASTAR Inc., Madison, WI) in the DNAstar package. On the basis of these results, the partial common reed sequence has two sequences (86 bp and 289 bp) that are absent in other cDNA sequences (). As we found donor and acceptor sites in our sequence that are necessary for splicing, one of them was the exon–intron junction (5′-end) which is called the donor site and another was intron–exon junction (3′-end) that is called the acceptor site (Gasser et al., Citation1987; Iida & Sasaki, Citation1983). Four common patterns were often used as signals for the first one [AG/GTA, /GTAAGT, RG/GTGAG, and AG/GTXXGT (R = A or G and X = A, T, G, or C)] (Iida & Sasaki, Citation1983). Thus, we found junction points in our sequence after cutting two regions in the common reed (intron regions), and finally, a sequence with 295 bp length resulted (), on which other analyses were carried out. Amino acid sequence of partial common reed sequence was obtained by the means of Mapdraw and Editseq software (DNASTAR Inc., Madison, WI). This sequence was then submitted to EBI and NCBI servers (www.ebi.ac.uk and www.ncbi.nlm.nih) to blast with similar amino acid sequences. The nucleotide sequence of our partial aro A (predicted) was aligned with the aro A from various plants using Megalign software (DNASTAR Inc., Madison, WI). A diagram of phylogenetic analysis (genetic distance) as determined by the Clustal W method using Megalign software (DNASTAR Inc., Madison, WI) is shown in . The comparison of nucleotide sequence of the P. australis aro A with other plants showed that P. australis aro A has highly sequence identity to aro A of Zea mays (94.6%), Eleusine indica (epsps-R), Zoysia japonica (94.2%), and Sorghum bicolor (94.2%) (). The amino acid sequence of P. australis aro A (predicted) was multiple aligned with other selected aro A from database. This alignment showed that the amino acid sequence has high similarity to aro A amino acid sequences from Oryza sativa (100%), Z. mays, Z. japonica (98%), and E. indica (epsps-R) (97.9%) (). A phylogenetic relationship, based on the genetic distance of the amino acid sequences as determined by the Clustal method using the DNAstar software, is shown in .
Figure 2. Analysis of the pTZ carrying the aro A gene. The PCR-amplified insert of the plasmid is shown in lanes 3–5 and plasmid without insert (negative control) is shown in lane 2. A 1 kb MW marker is shown in lane 1. Plasmid of lane 3 was selected to sequence.
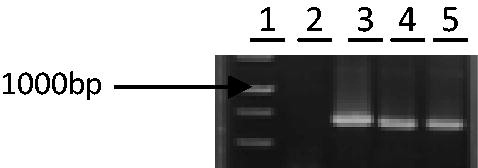
Figure 3. The partial sequence of Phragmites australis with intron regions. The underlined regions are primers (forward (TKZF) and reverse (TKZR)). The regions in gray box are introns (86 bp and 289 bp).
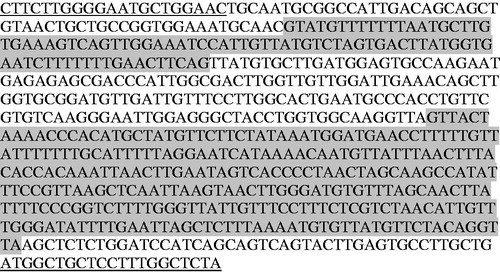
Figure 4. The partial sequence of aro A of Phragmites australis without intron regions. The underline regions are primers (forward (TKZF) and reverse (TKZR)).
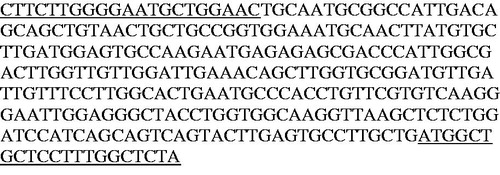
Figure 5. The phylogenic relationship of Phragmites australis with other species. A phylogenic tree (unrooted) based on the genetic distance of amino acid sequences was constructed by the Clustal method with using the DNAstar software.
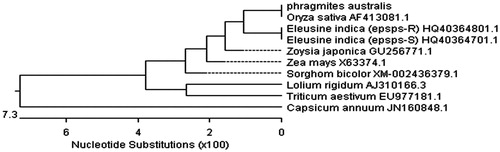
Table 1. Selected sequences from NCBI for multiple sequence alignment analysis.
Conserved region in common reed partial aro A gene
One conserved region was reported in different references that is the junction point of glyphosate. This conserved region is XLGNAG TAXRX that was reported in some species such as E. coli (in 90–102 position), S. typhimurium (90–102 position), P. hybrid (95–107 position), L. esculentum (95–107 position), A. thaliana (95–107 position), B. napus (95–107 position), and Z. mays (95–107 position). This shows that the region is the same among the mentioned species but in case of Aspergillus nidulans and Saccaromyces servisiae, this region is different. A comparison between partial amino acid sequence of common reed and above species showed that this region in the common reed is similar to the first mentioned species and different from A. nidulans and S. servisiae () (Padgette et al., Citation1991). This result shows that in the part of aro A of the common reed amino acid sequence is similar to the consensus sequence and some sequence of the species. So, this conserved sequence is conserved too.
Table 2. Sequence comparison of the conserved region of several aro A in organisms and Phragmites australis. Besides P. australis Smith, others have previously reported by Gasser et al. (Citation1987), Klee et al. (Citation1987), and Padgette et al. (Citation1991).
Consideration of polypeptide structure of common reed partial aro A gene
Amino acid sequence was submitted to PredictProtein and ExPASy servers (www.predictprotein.org and www.web.expasy.org/protparam) and following results were obtained: Sequence length: 98, secondary structure: loop = 46.9%, strand = 19.4%, helix = 33.7%, molecular weight: 9880.6, PI predict: 9.19, positive charge (glutamate, aspartate): 6, negative charge (arginine, lysine): 9, amino acid percent (), chemical formula: C438H727N121O127S5.
Table 3. Amino acid composition of partial EPSPS gene of Phragmites australis.
Conclusion
The sequence of the partial aro A gene isolated from P. australis was submitted in GenBank (www.ncbi.nlm.nih) with accession number Jn580998 for the first time. On the basis of this result, the cause of relative glyphosate tolerance in P. australis would be easy to determine in future research. This enzyme was registered with accepted name “3-phosphoshikimate 1-carboxyvinyltransferase” and alternative names “3-enol-pyruvoylshikimate-5-phosphate synthase”, “5-enolpyruvylshikimate-3-phosphate synthase”, and “EPSP synthase” with ENZYME entry “EC 2.5.1.19”. This enzyme catalyzes the following reaction:
Acknowledgements
Special thanks to Dr. Ram Chandra Suthar (Department of Biotechnology, P. S. Science and H. D. Patel Arts College, Kadi-382517, S.V. Campus, KSV University, Gujarat, India) and Mr. Utpol Das (Faculty of Life Science and Biotechnology South Asian University, New Delhi.) for critical reading of this manuscript.
Declaration of interest
The authors report no conflicts of interest. The authors alone are responsible for the content and writing of this article. The Razi University, Iran, has financially supported this research project.
References
- Back CL, Holomuzki JR. (2008). Long -term herbicide control of invasive, common reed (phragmites australis) at sheldon marsh, Lake erie. Ohio J Sci 108:108–12
- Baran M, Varadyova Z, Kracmar S, Hedbavny J. (2002). The common reed (Phragmites australis) as a source of roughage in ruminant nutrition. Acta Vet Brno 71:445–9
- Gasser SC, Winter JA, Hironaka CM, Shah DM. (1987). Structure, expression and evolution of the 5-enolpyruvylshikimate-3-phosphate synthase genes of petunia and tomato. J Biol Chem 263:4280–9
- Green JM. (2009). Evolution of glyphosate-resistant crop technology. Weed Sci 57:108–17
- Hollander-Czytko H, Amrhein N. (1987). 5-Enolpyruvylshikimate 3-phosphate synthase, the target enzyme of the herbicide glyphosate, is synthesized as a precursor in a higher plant. Plant Physiol 83:229–31
- Iida Y, Sasaki F. (1983). Recognition patterns for exon-intron junctions in higher organisms as revealed by a computer search. J Biochem 94:1731–8
- Kahrizi D, Salmanian AH, Afshari A, et al. (2007). Simultaneous substitution of Gly96 to Ala183 to Thr in 5-enolpyruvylshikimate 3-phosphate synthase gene of E. coli (k12) and transformation of rapeseed (Brassica napus L.) in order to make tolerance to glyphosate. Plant Cell Rep 26:95–104
- Kahrizi D, Salmanian AH. (2008). Substitution of Ala183Thr in aroA product of E. coli (k12) and transformation of rapeseed (Brassica napus) with altered gene to tolerance of plant to roundup. Transgenic Plant J 2:170–5
- Klee HJ, Muskopf YM, Gasser CS. (1987). Cloning of an Arabidopsis thaliana gene encoding 5-enolpyruvylshikimate-3-phosphate synthase: Sequence analysis and manipulation to obtain glyphosate-tolerant plants. Mol Gen Genet 210:437–42
- Lewendon A, Coggins JR. (1983). Purification of 5-enolpyruvylshikimate-3-phosphate synthase from Escherchia coli. Biochem J 213:187–91
- Marks M, Lapin B, Randall J. (1994). Phragmites australis (P. communis): Threat, management and monitoring. Nat Areas J 14:285–94
- Murray MG, Thampson WF. (1980). Rapid isolation of high molecular weight plant DNA. Nucleic Acid Res 8:4321–5
- Padgette SR, Biest DR, Gasser CS, et al. (1991). Site-directed mutagenesis of a conserved region of the 5-enolpyruvylshikimate-3-phosphate synthase active site. J Biol Chem 266:22364–9
- Sambrook J, Russell DW. (2001). Molecular Cloning, a Laboratory Manual. New York: Cold Spring Harbor Laboratory Press
- Schönbrunn E, Eschenburg S, Shuttleworth WA, et al. (2001). Interaction of the herbicide glyphosate with its target enzyme 5-enolpyruvylshikimate 3-phosphate synthase in atomic detail. Proc Nat Acad Sci 98:1376–80
- Stalker DM, Hiatt WR, Comai L. (1985). A single amino acid substitution in the enzyme 5-enolpyruvylshikimate-3-phosphate synthase confers resistance to the herbicide glyphosate. J Biol Chem 260:4724–8
- Yi Y, Qiao D, Bai L, et al. (2007). Cloning, expression and functional characterization of the Dunaliella salina 5-enolpyruvyl shikimate-3-phosphate synthase gene in Escherichia coli. J Microbiol 45:153–7