Abstract
Context: Acute kidney injury (AKI) is often encountered in patients receiving cisplatin (CisPt), a chemotherapeutic drug that induces numerous toxic side effects. Techniques used to limit nephrotoxicity during CisPt treatment are not fully effective; about a third of patients experience AKI. New nephroprotective strategies, including pharmacological approaches, must be developed.
Objective: The present study investigated the nephroprotective potential of Angelica sinensis (Oliv.) Diels (Apiaceae) root towards CisPt tubulotoxicity.
Materials and methods: HK-2 cells were incubated with CisPt (10 µM) and/or with a methanolic extract of A. sinensis (AS). Nephroprotective capacity was evaluated by means of cellular viability (resazurin assay) and apoptosis (annexin-V/PI staining), oxidative stress generation (H2DCF-DA oxidation), Ki-67 index (immunofluorescence), cell cycle analysis (DNA staining), cell migration rate (scratch assay), extracellular matrix deposition (collagen determination), and β-catenin relocalization.
Results: CisPt decreased cell viability [76% versus Ctrl], which was associated with an increased apoptosis. Simultaneous treatment with 50 µg/ml AS enhanced cell survival [84% versus Ctrl] and decreased the apoptosis rate. AS could not alleviate CisPt-induced oxidative stress; but doses of 5 and 50 µg/ml raised the Ki-67 index [135 and 244% versus Ctrl] and cell migration rates [1.2 and 1.3-fold versus Ctrl]. Finally, both doses of AS limited the amount of collagen deposition [121.6 and 119.6% for 5 and 50 µg/ml, respectively, versus 131.0% for CisPt-treated cells] and prevented the relocalization of β-catenin from the membrane to the nucleus.
Conclusion: These results confirm the nephroprotective potential of A. sinensis and require further investigations aiming at identifying its active compounds.
Introduction
Because of their unique roles in the organism, the kidneys are particularly vulnerable to the toxic effects of xenobiotics such as drugs or environmental contaminants. In fact, the kidneys receive about 25% of cardiac output. They are especially dedicated to the elimination and reabsorption of metabolites, and exert high metabolic activity. These factors contribute to making the kidneys the preferential targets of circulating toxic substances.
Cisplatin (cis-diamminedichloroplatinum or CisPt) is a metal complex used as a chemotherapeutic drug for the treatment of a variety of solid-organ tumors, including non-small cell lung carcinoma, testicular, ovarian, breast, head, and neck cancers (Pabla & Dong, Citation2008). Although CisPt appears to be a powerful drug for treating various forms of cancers, its use is associated with major adverse side effects such as nephrotoxicity, ototoxicity, neurotoxicity, bone marrow suppression, nausea and vomiting. Nephrotoxicity, leading to acute kidney injury (AKI), is probably the most concerning adverse effect of CisPt treatment. Thus, as the drug is highly detrimental to the structure and function of the kidneys, the dose must be limited, which may compromise the anticancer therapy (Yao et al., Citation2007).
When nephrotoxicity occurs, renal proximal tubular epithelial cells (RPTECs) undergo oxidative stress, which modifies the structure of biomolecules such as DNA, proteins and lipids. This cytotoxic activity may lead to cell death via apoptosis or necrosis (Lieberthal et al., Citation1996). The lack of tubular regeneration capacity, along with the resulting cell death, may lead to a transient loss of the kidneys function and structure. Fibrosis, a healing process characterized by the deposition of fibrotic tissue in the renal interstitium, is frequently observed. Fibrosis is a key element in the onset and progression of chronic kidney disease (Wynn, Citation2010).
AKI is partially reversible, but it can become more severe and can last longer with repeated injections of CisPt. Until now, the only successful strategy implemented clinically to avoid the onset of AKI consists in ensuring adequate hydration and diuresis of treated patients. The outcomes of this procedure are still not fully satisfactory, as about a third of patients treated with CisPt still experience AKI (Yao et al., Citation2007).
Recent research has aimed at identifying protective molecules and/or mechanisms that could be used to prevent or alleviate CisPt insults and the onset of AKI (Barabas et al., Citation2008; dos Santos et al., Citation2012). These pharmacological approaches have mainly focused on discovering compounds of natural origin (Nagwani & Tripathi, Citation2010; Nitha & Janardhanan, Citation2008; Sohn et al., Citation2009), notably issuing from Traditional Chinese Medicine (TCM) remedies.
The root of Angelica sinensis (Oliv.) Diels (Apiaceae), a TCM drug also known as Danggui (or Dong Quai in English), has been used traditionally as a blood tonic to treat female sexual dysfunction such as menstrual disorders and menopausal symptoms (Chao & Lin, Citation2011; Low Dog, Citation2005). Modern research has highlighted several other pharmacological effects such as anti-inflammatory activity (Chao & Lin, Citation2011), immunomodulation (Choy et al., Citation1994), anti-tumoral activity (Chao & Lin, Citation2011), neuroprotection (Lin, Citation2011) and hepatoprotection (WHO, Citation2004).
In TCM treatments, Angelica sinensis is often prescribed in association with Astragalus membranaceus (Fisch.) Bge (Fabaceae). Studies using this combination realized in vitro (Wojcikowski et al., Citation2009) and in vivo (Meng et al., Citation2011; Wang et al., Citation2004) reported nephroprotective activities towards various toxicity models. A renoprotective activity has also been reported during clinical trials (Li & Wang, Citation2005).
The present in vitro study aimed at characterizing how CisPt-mediated tubulotoxicity may be alleviated by A. sinensis extract. Five key biological processes occurring during AKI were investigated: (i) cellular death mediated by apoptosis (Havasi & Borkan, Citation2011; Pabla & Dong, Citation2008; Wynn, Citation2010); (ii) increased oxidative stress; (iii) tubular regeneration capacities of healthy cells (Lee & Kalluri, Citation2010; Megyesi et al., Citation2002; Wynn, Citation2010); (iv) extracellular matrix (ECM) deposition, involving notably collagen (Yang et al., Citation2010; Wynn, Citation2010); and (v) dedifferentiation processes of epithelial cells via activation of the β-catenin pathway (Hao et al., Citation2011; Liu, Citation2010).
Material and methods
Herbal extract
Bulk Angelicae sinensis radix was kindly provided by Complemedis AG (Trimbach, Switzerland). A certificate of analysis issued by Phytax GmbH (Schlieren, Switzerland) reports compliance to the European Pharmacopoeia 6.0. A voucher specimen was stored in our laboratory. The roots were ground into powder and extracted with methanol (1:10) during 2 h under agitation. The extraction was repeated three times; the solutions were filtered, combined and evaporated to dryness using an AES SpeedVac device (Savant, Farmingdale, NY). The yield was 32.4%. The extract (AS) was stored at −20 °C until use.
HPLC-UV for phytochemical analysis of the extract
The ferulic acid content and the Z-ligustilide content were determined in the AS methanolic extract.
Ferulic acid determination
The method was adapted from the European Pharmacopoeia 6.0 (Council of Europe, Citation2013). The chromatographic conditions were as follows: pre-column, Adsorbosphere C18 All-Guard 5 µm (25 × 4.6 mm i.d.; Alltech, Deerfield, MA); column, Waters Symmetry C18 5 µm (150 × 3.9 mm i.d.; Milford, MA). The mobile phase consisted in a gradient of acetonitrile/water (8:92) + 0.5% acetic acid (solvent A) and acetonitrile (solvent B): 0 min: 100% A; 19 min: 100% A; 20 min: 0% A; 28 min: 0% A; 29 min: 100% A; 34 min: 100% A. Oven temperature was maintained at 35 °C. The injection volume was 20 µl and the flow rate was 0.8 ml/min. The component was quantified at the wavelength of 316 nm.
Z-Ligustilide determination
The chromatographic conditions were adapted from the literature (Yan et al., Citation2008) as follows: pre-column, adsorbosphere C18 All-Guard 5 µm (25 × 4.6 mm i.d.; Alltech, Deerfield, MA); column, Waters Atlantis C18 5 µm (250 × 4.6 mm i.d.; Milford, MA). The mobile phase consisted in a gradient of water + 0.1% acetic acid (solvent A) and methanol (solvent B): 0 min: 60% A; 3 min: 60% A; 18 min: 0% A; 21 min: 0% A; 22 min: 60% A; 23 min: 60% A. Oven temperature was maintained at 35 °C. The injection volume was 10 µl and the flow rate was 1.0 ml/min. The component was quantified at the wavelength of 384 nm.
Cell culture and treatment
HK-2 cells, originating from RPTECs, were obtained from American Type Culture Collection (CRL-2190, ATCC, Manassas, VA), and grown in low-glucose DMEM containing 10% fetal bovine serum (FBS PAA Clone, PAA laboratories, Pasching, Austria), 2 mM l-glutamine and 1% penicillin-streptomycin. Cells were sub-cultured or harvested for experiments when they reached about 90% confluence. For experimental purposes, cells were used between passages 6 and 25, harvested by trypsinization and seeded on 60 mm Petri dishes (4 × 105 cells), 4-well-chambered slides (Lab-Tek II, Nunc, Rochester, NY) (2.4 × 104 cells), 12-well plates (8 × 104 cells) or 96-well plates (1 × 104 cells). Next, the cells were incubated for 24 h in FBS-containing medium, rinsed twice with DMEM and treated with the studied substances in FBS-depleted medium. Cells assigned to be protected against the nephrotoxic effect were pre-treated with AS extract 1 h prior to the addition of CisPt solution.
CisPt solutions were prepared from the marketed drug Cisplatine Hospira® (Hospira Benelux, Antwerpen, Belgium). Working doses were selected according to the results obtained from preliminary experiments based on MTT and crystal violet assays (data not shown). The working concentration of CisPt corresponded to its LD25; and the working concentration of AS extract corresponded to the highest no-effect concentration (i.e., not exceeding LD1).
Cell viability assay
Cells were treated with CisPt, with AS, or with a combination of CisPt and AS in 96-well plates, washed twice with PBS, and assessed for their viability by incubation for 1.5 h with 0.44 mM resazurin solution (Sigma-Aldrich, St Louis, MO). Absorbances were measured at 540 and 620 nm using an iEMS Reader MF spectrophotometer (Thermo Labsystems, Breda, The Netherlands). The percentage of reduced dye was calculated with the following formula:
where ɛOX is the molar extinction coefficient of resazurin (47.6 at 540 nm and 34.8 at 620 m); ɛRED is the molar extinction coefficient of resorufin (104.4 at 540 nm and 5.5 at 620 nm); A is the absorbance of test wells; A′ is the mean absorbance of blank wells; λ1 = 540 nm; λ2 = 620 nm. Viability (i.e., metabolic activity) was normalized against the control condition.
Apoptosis detection with annexin V/propidium iodide staining
Cells were treated with CisPt or with a combination of CisPt and AS in 12-well plates, harvested and centrifuged at 1400 g. The supernatant was discarded and cells were resuspended and incubated with an Annexin V-FITC detection kit (BD Pharmingen, San Diego, CA) in the dark for 15 min. Suspensions were then analyzed using a BD FACSCanto II flow cytometer (BD Pharmingen, San Diego, CA) and 104 cells were recorded. The data were analyzed with FlowJo software (Tree Star, Ashland, OH); debris and cell clumps were removed by gating, and the proportions of living, apoptotic, and necrotic cells were calculated.
Oxidative stress measurement
Cells were treated with CisPt, with AS, or with a combination of CisPt and AS in 12-well plates, rinsed and treated with 10 µM 2′,7′-dichlorodihydrofluorescein diacetate (H2DCF-DA) solution (Sigma-Aldrich, St Louis, MO). Cells were harvested, centrifuged at 1400 g and analyzed using a BD FACSCanto II flow cytometer (BD Pharmingen, San Diego, CA); 104 cells were recorded. The data were analyzed with FlowJo software (Tree Star, Ashland, OH); debris and cell clumps were removed by gating, and mean fluorescence intensities (MFI; geometric mean) were estimated.
Ki-67 immunostaining
Cells were treated with AS or CisPt on chambered slides, rinsed twice in PBS, fixed in 4% paraformaldehyde solution for 20 min, then rinsed and permeabilized with 0.01% Triton X for 5 min. Cells were blocked with goat serum (PAA Laboratories, Pasching, Austria) for 1 h, then incubated with a primary rabbit anti-Ki-67 antibody (Abcam, Cambridge, UK) for 1 h. The slides were rinsed twice and incubated with a secondary Alexa Fluor 488 conjugated goat anti-rabbit antibody (Invitrogen, Eugene, OR) for 30 min. The slides were rinsed twice, mounted with DAPI-containing mountant (Invitrogen, Eugene, OR) and examined with an Axioskop fluorescence microscope (Zeiss, Germany) at magnification 400×. Twenty pictures per chamber were acquired. The Ki-67 index was calculated by dividing the number of Ki-67 positive cells by the total number of cells (Yang et al., Citation2010).
Cell cycle analysis
Following treatment in 12-well plates, cells were harvested and fixed with ice-cold 66% ethanol, then incubated at 4 °C for 1 h. Pellets were centrifuged at 1400 g, washed with PBS, resuspended in PI/RNase Staining Buffer (BD Pharmingen, San Diego, CA) following the manufacturer’s instructions, then incubated at room temperature for 30 min. Cells were washed, resuspended and analyzed using a BD FACSCanto II flow cytometer (BD Pharmingen, San Diego, CA). About 104 HK-2 cells were recorded using the BP 585/42 filter at a maximum rate of 200 events/s.
Cell cycle analysis was carried out with FlowJo software (Tree Star, Ashland, OH): debris and cell clumps were removed by gating, and proportions of G0/G1, S, and G2/M cells were determined according to the Dean-Jett-Fox model (Anonymous, Citation2011).
Wound healing assay
Cells were seeded on 60-mm Petri dishes, incubated for 48 h, yielding approximately 90–100% confluence, and were then growth arrested by serum starvation for 24 h. Linear scratches were made through cell monolayers with a sterile 200 µl pipette tip. Debris were removed and 10 pictures were taken (time t0) using a Motic AE21 microscope equipped with a Moticam 2300 camera (Motic, Wetzlar, Germany) at magnification 100×. Cells were then treated with AS or FBS for 18 h, and 10 new pictures of the scratch were taken (time t18). The area of the scratch was measured using TScratch (Geback et al., Citation2009) and the migration rate (µm/h) was calculated.
Assessment of collagen synthesis
Cells were treated with CisPt, with AS, or with a combination of CisPt and AS in 96-well plates, rinsed with PBS, and assessed for their metabolic activity with the resazurin assay as described above. Next, cells were washed with PBS, fixed with ice-cold methanol for 1 h at 4 °C, rinsed twice with 1% acetic acid and stained for 2 h with 0.1% picrosirius red (PSR) staining solution (Hu et al., Citation2009). Wells were then rinsed three times with 1% acetic acid. The dye was solubilized in 0.1 M NaOH and the absorbances of the wells were measured at 540 nm. Absorbances attributed to PSR staining were normalized according to the metabolic activity of each well. The correlation between the number of cells, metabolic activity, and the amount of protein determined by the bicinchoninic acid method was verified in preliminary experiments.
β-catenin determinations
Membranous β-catenin determination
Cells were treated with CisPt, with AS, or with a combination of CisPt and AS on 4-well chambered slides. The slides were rinsed, fixed in 4% paraformaldehyde solution for 20 min, rinsed and permeabilized with 0.01% Triton X for 5 min. The slides were rinsed again and cells were blocked with donkey serum (sc-2044, Santa Cruz Biotechnology, Santa Cruz, CA) for 1 h. The slides were then incubated with mouse anti-human β-catenin primary antibody (sc-7963, Santa Cruz Biotechnology, Santa Cruz, CA) for 1 h, washed twice, incubated with cyanine-3 conjugated donkey anti-mouse secondary antibody (715-166-151, Immuno Research Lab Jackson, West Grove, PA) for 30 min, rinsed twice, mounted with DAPI-containing Prolong Gold antifade reagent (Invitrogen, Eugene, OR) and examined at magnification 400× with an Axioskop fluorescence microscope (Zeiss, Germany) equipped with a DP200 camera (Deltapix, Maalov, Denmark).
Prior to the evaluation of fluorescence intensity, the domain of linearity and the reproducibility of the method were controlled using Inspeck™ Orange (λEx = 540 nm/λEm = 560 nm) fluorescently labeled microbeads (6.0 µm diameter) (Molecular Probes, Eugene, OR).
For each condition, 10 pictures were taken, and analyzed using Fiji (Fiji Is Just ImageJ) software (International Association for Universal Design, Ohtsubo, Japan). The fluorescence intensity was evaluated by the measurement of luminance. The nuclei were counterstained with DAPI. The blue-fluorescent areas were discarded from the pictures, allowing removal of the signals corresponding to nuclear β-catenin.
Cytoplasmic/nuclear β-catenin determination
Cells were treated with CisPt or with a combination of CisPt and AS in 12-well plates, harvested, centrifuged at 500 g and fixed in Cytofix/Cytoperm solution (BD Pharmingen, San Diego, CA) for 20 min at 4 °C. The suspension was washed twice, and incubated with anti-human β-catenin-phycoerythrin monoclonal antibody (R&D Systems, Mineapolis, MN) in the dark for 30 min. Cells were washed twice and analyzed using a BD FACSCanto II flow cytometer (BD Pharmingen, San Diego, CA). A minimum of 104 cells were recorded. The data were analyzed with FlowJo software (Tree Star, Ashland, OR): debris and cell clumps were removed, and mean fluorescence intensities (MFI; geometric mean) were calculated.
Statistical analysis
Unless otherwise stated, experiments were performed four times using biologically independent samples. Results to be normalized against controls were treated as described previously (Valcu & Valcu, Citation2011). Data were compared by means of a one-way ANOVA with post-hoc Student’s t-test (Bonferroni correction) using GraphPad Prism 5 software (GraphPad, San Diego, CA); p values <0.05 were considered significant.
Results
Phytochemical analysis of the AS extract
Two compounds (ferulic acid and Z-ligustilide) were assessed by means of HPLC-UV. The amounts of ferulic acid content and Z-ligustilide content per g of crude methanolic extract were 0.743 ± 0.005 mg and 30.55 ± 0.71 mg, respectively. The chromatogram obtained for ferulic acid determination, employing conditions adapted from the European Pharmacopoeia 6.0, is displayed in Figure 1(S) (Supplementary file). Both values are within the range of concentrations previously reported for these compounds (Chao & Lin, Citation2011; WHO, Citation2004).
AS protects HK-2 cells against CisPt-induced mortality
After treatment with 10 µM CisPt for 48 h, HK-2 cell survival dropped to 76 ± 2% compared with control conditions (). Upon co-treatment with 50 µg/ml AS, the cell survival was enhanced to 84 ± 3% (p < 0.01). No statistically significant effect was observed for co-treatment with CisPt and a concentration of 5 µg/ml AS compared with CisPt treatment alone. Interestingly, cells treated with both 50 and 5 µg/ml AS alone displayed higher viability rates (110 ± 2% and 109 ± 2%, respectively).
AS reduces CisPt-induced HK-2 cells apoptosis
The protective effects of AS towards CisPt-induced cell death were further confirmed by analyzing apoptosis rates. To assess the proportions of cells undergoing apoptosis, flow cytometry was used to detect annexin V/PI stained cells. Necrotic cells – for which no modification was observed upon treatment with CisPt as compared with the control condition – were not taken into account. In fact, necrosis only occurs at high concentrations of CisPt (dos Santos et al., Citation2012).
The treatment of HK-2 cells with 10 µM CisPt raised the proportion of apoptotic cells from 4.2 ± 0.6% in the control condition to 12.9 ± 1.6% (). Upon co-treatment with both 50 and 5 µg/ml AS, the apoptosis rates were significantly reduced to 8.4 ± 1.7 and 8.0 ± 1.0%, respectively.
Figure 2. Proportions of apoptotic cells after treatment for 48 h with 10 μM CisPt and/or 50 or 5 μg/ml AS determined with flow cytometry determination following Annexin V/PI staining. Results are displayed as means ± SD of four independent experiments (**p < 0.01; ***p < 0.001).
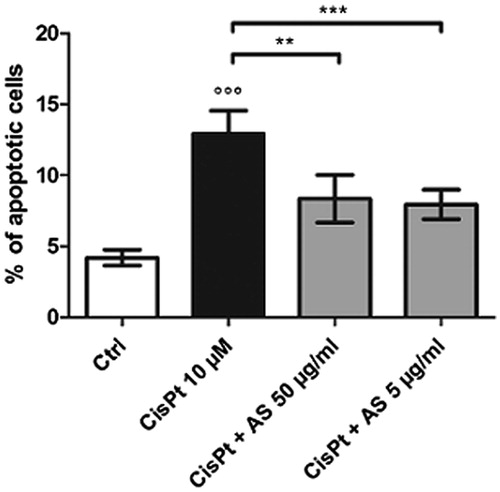
These results confirm the capability of A. sinensis to reduce HK-2 cell mortality when given concomitantly with CisPt. This effect appeared to be mediated by a lower apoptosis rate which was not concentration related in the investigated range.
AS does not reduce CisPt-induced oxidative stress
After 24 and 48 h treatment with 10 µM CisPt, HK-2 cells exhibited increased fluorescence intensities (131 ± 8% and 179 ± 12% above those in the control condition, respectively), as detected by oxidized H2DCF-DA probe. The results displayed in indicate that co-treatment with 50 or 5 µg/ml AS did not alleviate oxidative stress at either of the incubation times tested.
AS promotes HK-2 cell proliferation
The Ki-67 index was measured after immunostaining cells treated for 48 h with the AS extract or CisPt. Upon treatment with 10% FBS, the proportion of proliferative cells rose to 307 ± 3% as compared to the serum-depleted control condition (). This phenomenon is due to EGF (epidermal growth factor), which is present in FBS (Ryan et al., Citation1994). CisPt treatment also increased the Ki-67 index to 216 ± 5%, but this effect should be regarded as the consequence of the toxic activity of CisPt that triggers a G2/M cell cycle arrest, leading to artificially higher Ki-67 indexes (Bunel et al., Citation2014; Jamieson & Lippard, Citation1999).
Figure 4. Proliferation (Ki-67) index (A) and cell cycle distribution (B) assessments for HK-2 cells treated for 48 h with test substances. Results are means ± SD of four independent experiments (**p < 0.01; ***p < 0.001 compared to the control group).
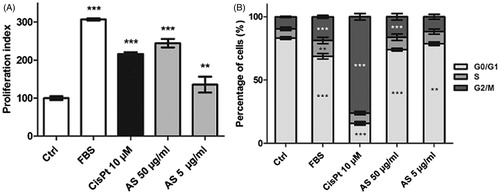
AS treatment at doses of 50 and 5 µg/ml raised the Ki-67 index to 244 ± 11% and 135 ± 21%, respectively. A cell cycle analysis was performed to determine if increase in the Ki-67 index was a consequence of a cell cycle arrest, or an enhancement of the cell proliferation rate (). FBS induced a slight decrease in the proportion of G0/G1 cells (68.8 ± 2.2% versus 83.2 ± 1.0% in the control condition) and a slight increase in the proportion of G2/M cells (18.7 ± 1.1% versus 9.5 ± 0.5% in the control condition). CisPt treatment triggered G2/M checkpoint arrest, resulting in a sharp decrease in G0/G1 cells (15.8 ± 1.4%) associated with a sharp increase in G2/M cells (76.1 ± 2.6%).
Both 50 and 5 µg/ml doses of AS induced slight modulations in the cell cycle phases distribution comparable with those observed with FBS treatment: G0/G1 cells decreased (74.1 ± 1.1% and 78.7 ± 1.4% for 50 and 5 µg/ml AS, respectively) and G2/M cells increased (16.1 ± 2.6% for 50 µg/ml and 11.7 ± 2.0% (not significant) for 5 µg/ml AS). These results suggest that the AS effect on the Ki-67 index was a consequence of an enhanced cell proliferation rate rather than a cell cycle arrest.
AS enhances cell motility
The scratch assay aims at evaluating the motility of cells and is commonly used to assess the potential of substances to enhance cellular regeneration capacity (Ruszymah et al., Citation2012). In an AKI setting, cellular migration may contribute to tubules regeneration and thus curb the onset of fibrosis (Liu, Citation2006). After the scratch was created, a 10% FBS treatment induced a 1.9-fold increase in cell migration rates over the control condition (), whereas 50 and 5 µg/ml AS enhanced cell migration to approximately 1.3- and 1.2-fold over the control condition, respectively.
Figure 5. Scratch assay for HK-2 cells after 24 h serum-deprivation. Monolayers were scratched with a pipette tip and incubated with test substances for 18 h. Phase contrast microscopy images (100 × magnification) for control, FBS, 50, and 5 μg/ml AS treatments at start (left pictures) and after 18 h (right pictures). Migration rates are displayed as means ± SD of four independent experiments (*p < 0.05; **p < 0.01; ***p < 0.001 compared to the control conditions).
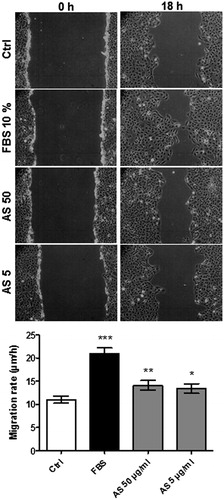
CisPt-induced collagen deposition is limited by AS co-treatment
Picrosirius red staining allows the collagen produced by cell cultures to be quantified (Hu et al., Citation2009; Xu et al., Citation2007). Exposure of HK-2 cells to 50 and 5 µg/ml AS for 48 h did not modify the amount of collagen produced as compared to the control condition (). However, when CisPt produced a 131.0 ± 6.1% increase in collagen synthesis, both doses of AS limited the deposition to 119.6 ± 4.1% (50 µg/ml) and to 121.6 ± 4.4% (5 µg/ml).
Figure 6. Collagen quantification upon treatment of HK-2 cells with test substances for 48 h and PSR staining. Absorbances were normalized according to their metabolic activity (resazurin assay) and compared to the control condition. Results are displayed as means ± SD of four independent experiments (*p < 0.05; **p < 0.01; ***p < 0.001).
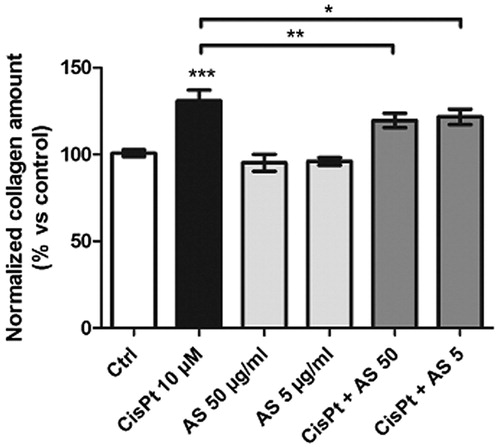
Assessments of β-catenin
Membranous β-catenin
The amounts of membranous β-catenin were assessed by means of quantitative fluorescence image analysis (QFIA). After 48 h treatment with 10 µM CisPt, membranous β-catenin expression was reduced 3.2-fold compared to the control condition (). Upon co-treatment with 50 and 5 µg/ml AS, β-catenin losses in both cases fell 2.1-fold below those in the control condition. This indicates that AS was capable of preventing the loss of the epithelial phenotype induced by CisPt.
Figure 7. Immunostained membranous β-catenin after treatment for 48 h for control (A) and CisPt conditions (B). Ten images were acquired per condition and fluorescence intensities were measured; DAPI counterstaining was subtracted to remove eventual β-catenin translocated to the nucleus. Luminance values were then recorded (C). Results are displayed as means ± SD of four independent experiments (*p < 0.05; ***p < 0.001 compared to the control group). Representative fluorescence images confirming the nuclear/cytoplasmic localization of β-catenin for control (D) and CisPt treatment (E). Cells were analyzed with a flow cytometry technique and the geometrical mean fluorescence intensity was evaluated (F). Means ± SD of four independent experiments (**p < 0.01; ***p < 0.001).
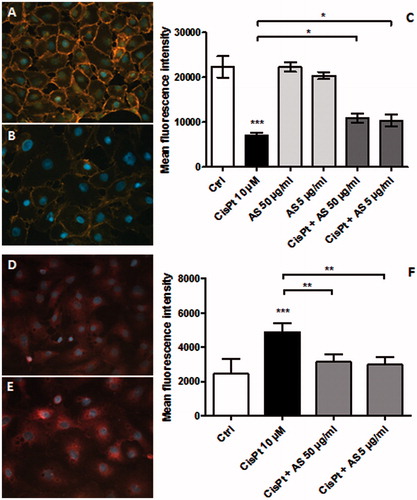
Intracytoplasmic/nuclear β-catenin
HK-2 cells grown in the same conditions were stained for intracytoplasmic/nuclear β-catenin and analyzed by flow cytometry. As shown in , compared with the control condition, CisPt induced a 2.0-fold increase in the fluorescence intensities attributed to the nuclear form of β-catenin. Upon co-treatment with 50 and 5 µg/ml AS, the increase dropped to 1.3- and 1.2-fold, respectively. This indicates that AS prevented the relocalization of β-catenin from the membrane to the nucleus.
Discussion
CisPt exerts its action not only on tumor cells but also on RPTECs that are exposed to the glomerular ultrafiltrate. Through oxidative stress and DNA binding, CisPt triggers apoptosis (Cummings & Schnellmann, Citation2002), cell cycle arrest (dos Santos et al., Citation2012) and cellular dedifferentiation processes (Zeisberg & Duffield, Citation2010) that are the starting points for AKI and fibrotic repair (Wynn, Citation2010). Effectively preventing AKI may help to curb the onset of interstitial fibrosis and the progression to end-stage renal disease.
In the present study, a crude polar (methanolic) extract of A. sinensis showed several protective effects towards the key biological phenomena involved in AKI.
Prevention of cell death via apoptosis
It has been reported that CisPt-mediated cell death in vivo was governed by apoptotic and necrotic pathways depending on the concentration of the drug. The relative contribution of each process in nephrotoxicity and AKI has not yet been determined (dos Santos et al., Citation2012). Nevertheless, lowering the apoptosis rate may enhance overall cell survival, and thus avoid the loss of functional tubules that is responsible for the AKI setting.
In our experimental setting, both doses of AS (50 and 5 µg/ml) were observed to prevent CisPt-induced cellular death, as measured by the resazurin assay. However, only the highest dose protected cells at a statistically significant level. A significant reduction in the apoptosis rate was observed at both tested doses. While CisPt induced a 3.1-fold increase in the proportion of apoptotic cells compared to the control condition, AS co-treatment decreased this rate to 1.6-fold. As each dose of AS showed a similar efficacy in lowering apoptosis, a lower apoptosis rate may not be the only factor enhancing cell survival, which improved slightly with the higher AS concentration. One would expect additional necrotic processes to be a possible explanation for this discrepancy. In our experimental conditions, CisPt did not produce higher necrosis rates. Other mechanisms, such as an enhancement in cellular proliferation or prevention of cell detachment, might be involved (cf. “Factors possibly enhancing tubular regeneration” and “Membranous β-catenin” sections).
Effects on oxidative stress
After incubation with CisPt for 24 and 48 h, ROS/RNS generation increased in HK-2 cells. Indeed, oxidative stress is an important feature of CisPt toxicity in vivo (Yao et al., Citation2007). AS extract was tested for its potential to alleviate oxidative stress, but none of the tested concentrations had a beneficial effect. Thus, the protective effects of A. sinensis towards CisPt-mediated nephrotoxicity in our model cannot be explained by a neutralization of oxidative/nitrosative stress.
Previous studies have reported a moderate antioxidant activity for an ethanolic extract of A. sinensis (Yang et al., Citation2009), as well as for its polysaccharides. The polysaccharides can be extracted with water and have been shown to have a hepatoprotective activity mediated by oxidative stress reduction (Yu et al., Citation2013).
Factors possibly enhancing tubular regeneration
Enhancing the renewal of RPTECs, by increasing both their cellular proliferation and migration capacity, is important for successful tubule repair following CisPt insults (Wynn, Citation2010).
The Ki-67 index was used to evaluate the proliferative potential of healthy HK-2 cells. This protein is located on the nuclei of proliferating cells (i.e., in G1, S, G2, and M phases of the cell cycle) and remains absent in quiescent cells (G0) (Urruticoechea et al., Citation2005). Ki-67 is commonly used to assess the proliferative capacities of tumors. It has also been used in vitro and in vivo for the assessment of RPTEC regeneration (Docherty et al., Citation2006; Pozdzik et al., Citation2008).
CisPt induced a 2.3-fold increase in the Ki-67 index over that in the control condition. This effect was a consequence of its toxicity rather than an enhancement of cell proliferation. Indeed, it is well known that CisPt cross-links DNA strands and triggers cell cycle arrest at G2/M checkpoint, giving rise to higher proportions of Ki-67 positive cells (Jamieson & Lippard, Citation1999). A flow cytometry analysis of the cell cycle phases distribution confirmed this observation: the CisPt treatment yielded a 8.0-fold increase in G2/M cells compared to the proportion in the control condition ().
The use of FBS (10%) as a proliferation inducer increased the Ki-67 index 3.1-fold over that in the control condition. It also moderately increased G2/M cells by 2.0-fold. Both doses of AS yielded similar patterns: for 50 and 5 µg/ml AS, the Ki-67 index increased by 2.4- and 1.4-fold, respectively, whereas the proportion of G2/M cells rose by 1.7- and 1.2-fold, respectively.
The G0/G1 cell profiles measured in the presence of CisPt or AS are consistent with these findings: when CisPt triggered a 5.3-fold decrease in the proportion of G0/G1, FBS produced a decrease of only 1.2-fold, and both 50 and 5 µg/ml triggered an approximately 1.1-fold decrease compared to the control condition. These results suggested that A. sinensis promoted the proliferation of healthy HK-2 cells, probably by stimulating G1 phase entrance, thus lowering the proportion of quiescent cells.
The results obtained for cell proliferation assays are consistent with previous work focusing on cellular proliferation of gastric epithelial cells induced by A. sinensis (Ye et al., Citation2001). Furthermore, the higher cell survival rates (i.e. higher metabolic activities) observed for both 50 and 5 µg/ml AS () can probably be explained by an enhanced cell proliferation capacity, as measured with the Ki-67 index.
Cellular migration, assessed by a scratch assay, highlighted an enhancement in motility rates for both tested doses of AS extract compared to the control conditions. Mechanisms that might explain the effects of A. sinensis on wound healing have already been suggested in a previous paper focusing on fibroblasts (Hsiao et al., Citation2012). These include the downregulation of MARE1 (microtubule-associated protein RP/EB) and NDKB (nucleoside disphosphate kinase B) and upregulation of ARPC5 (actin-related protein 2/3 complex subunit 5) and STMN1 (stathmin).
Considered altogether, these effects support the hypothesis that A. sinensis can help to accelerate cellular regeneration following AKI, a disorder during which the loss of RPTECs is associated with a transient – but not fully irreversible – loss of tubular structure and function (Bucaloiu et al., Citation2012).
Reduction of collagen synthesis
Collagen is a component of fibrotic tissue that is secreted by cells during the scarring process. It also acts as a fibrosis-promoting factor (Wynn, Citation2007). Thus, preventing its deposition may help to preserve the integrity of the interstitial compartment and favor tubular regeneration.
Our results have shown that the tested AS extract reduced the amount of collagen that was deposited following CisPt treatment of HK-2 cells. Angelica sinensis could thus be useful in lowering ECM production, and could probably attenuate fibrotic repairs associated with AKI.
Localization of β-catenin
β-catenin plays a dual role in RPTECs: it is involved in cell adhesion, where it links E-cadherin to the actin cytoskeleton. It may thus be used to evaluate the integrity of the epithelial phenotype in kidneys or cultured cells (Zeisberg & Neilson, Citation2009). When RPTECs are injured, these intercellular links are disrupted and β-catenin relocalizes into the nucleus after structural reconfiguration (Gottardi & Gumbiner, Citation2004). It then associates with the TCF/LEF complex and rules the transcription of several genes, notably those involved in fibrosis (Bozic et al., Citation2011). Research has suggested that inhibiting the β-catenin pathway restrains the progression of renal fibrosis (Hao et al., Citation2011; He et al., Citation2011).
Membranous β-catenin
Quantitative fluorescence image analysis of HK-2 cells revealed that CisPt treatment could trigger a massive loss of membranous β-catenin (3.2-fold decrease) compared with control conditions. The AS extract treatment alone did not modify the amount of membranous β-catenin. Co-treatment with CisPt and AS limited this loss at both tested doses of AS (2.1- and 2.2-fold decrease at 50 and 5 µg/ml, respectively). Angelica sinensis was thus able to prevent HK-2 cells from losing their epithelial phenotype upon CisPt treatment. This may prevent cells from detaching and enhance overall survival by maintaining cell–cell interactions.
Internal β-catenin
Measurement by flow cytometry of the amount of relocalizing β-catenin revealed a 2.0-fold increase in nuclear/cystoplasmic proportions of this isoform upon CisPt treatment. Co-incubation with AS restrained the relocalizing form of β-catenin to a 1.3- and 1.2-fold increase over the control condition for 50 and 5 µg/ml AS, respectively. This result indicates that A. sinensis could be effective in reducing the transcription of genes involved in fibrotic processes.
Conclusion
When considered altogether, the results obtained in this work support in vivo data in the literature from a mechanistic point of view, and thus confirm the promising nephroprotective potential of A. sinensis towards CisPt tubulotoxicity. This herbal remedy originating from TCM was found to prevent cell death by lowering CisPt-induced apoptosis rates, and to promote tubular regeneration by enhancing both cellular proliferation and migration. It also lowered ECM deposition, a key process in fibrotic repair, and attenuated β-catenin relocalization, a process that involves the loss of the epithelial phenotype of RPTECs and the accumulation of its nuclear form. Angelica sinensis can thus be considered as a potential TCM remedy that can alleviate the severity and duration of CisPt-induced AKI, and in the longer term, prevent the onset of kidney fibrosis.
Further studies aiming at identifying the compound(s) and the molecular mechanisms responsible for the activities of A. sinensis highlighted in this work are currently under investigation.
Supplementary material available online
Supplementary Figure 1S.
Figure_1S.jpg
Download JPEG Image (32.7 KB)Acknowledgements
The authors are grateful to Complemedis AG (Trimbach, Switzerland) for kindly providing bulk Radix Angelicae sinensis. Thanks are also expressed to E. De Prez, M. Faes and O. Vaillant for skillful technical assistance and to T. Baudoux and C. Husson for their valuable advice on flow cytometry. Prof. J.-M. Kauffmann and Prof. G. Lucy are gratefully acknowledged for providing their help for the revision of the manuscript.
Declaration of interest
Valérian Bunel is a fellow of the Fonds de la Recherche Scientifique – FNRS (FRIA grant). The authors report no declarations of interest.
References
- Anonymous. (2011). FlowJo, data analysis software for flow cytometry. In: FlowJo Advanced Tutorial. 7.6.2 ed. Ashland: Tree Star Inc
- Barabas K, Milner R, Lurie D, Adin C. (2008). Cisplatin: A review of toxicities and therapeutic applications. Vet Comp Oncol 6:1–18
- Bozic M, De Rooij J, Parisi E, et al. (2011). Glutamatergic signaling maintains the epithelial phenotype of proximal tubular cells. J Am Soc Nephrol 22:1099–111
- Bucaloiu ID, Kirchner HL, Norfolk ER, et al. (2012). Increased risk of death and de novo chronic kidney disease following reversible acute kidney injury. Kidney Int 81:477–85
- Bunel V, Antoine MH, Nortier J, et al. (2014). Protective effects of schizandrin and schizandrin B towards cisplatin nephrotoxicity in vitro. J Appl Toxicol 34:1311–19
- Chao WW, Lin BF. (2011). Bioactivities of major constituents isolated from Angelica sinensis (Danggui). Chin Med 6:1–7
- Choy YM, Leung KN, Cho CS, et al. (1994). Immunopharmacological studies of low molecular weight polysaccharide from Angelica sinensis. Am J Chin Med 22:137–45
- Council of Europe. (2013). European Pharmacopoeia. Strasbourg: Tec & Doc
- Cummings BS, Schnellmann RG. (2002). Cisplatin-induced renal cell apoptosis: Caspase 3-dependent and -independent pathways. J Pharmacol Exp Ther 302:8–17
- Docherty NG, O’Sullivan OE, Healy DA, et al. (2006). TGF-beta1-induced EMT can occur independently of its proapoptotic effects and is aided by EGF receptor activation. Am J Physiol Renal Physiol 290:F1202–12
- Dos Santos NA, Carvalho Rodrigues MA, Martins NM, Dos Santos AC. (2012). Cisplatin-induced nephrotoxicity and targets of nephroprotection: An update. Arch Toxicol 86:1233–50
- Geback T, Schulz MM, Koumoutsakos P, Detmar M. (2009). TScratch: A novel and simple software tool for automated analysis of monolayer wound healing assays. Biotechniques 46:265–74
- Gottardi CJ, Gumbiner BM. (2004). Distinct molecular forms of beta-catenin are targeted to adhesive or transcriptional complexes. J Cell Biol 167:339–49
- Hao S, He W, Li Y, et al. (2011). Targeted inhibition of β-catenin/CBP signaling ameliorates renal interstitial fibrosis. J Am Soc Nephrol 22:1642–53
- Havasi A, Borkan SC. (2011). Apoptosis and acute kidney injury. Kidney Int 80:29–40
- He W, Kang YS, Dai C, Liu Y. (2011). Blockade of Wnt/beta-catenin signaling by paricalcitol ameliorates proteinuria and kidney injury. J Am Soc Nephrol 22:90–103
- Hsiao CY, Hung CY, Tsai TH, Chak KF. (2012). A study of the wound healing mechanism of a Traditional Chinese Medicine, Angelica sinensis, using a proteomic approach. Evid Based Complement Alternat Med 2012:467531
- Hu Q, Noor M, Wong YF, et al. (2009). In vitro anti-fibrotic activities of herbal compounds and herbs. Nephrol Dial Transpl 24:3033–41
- Jamieson ER, Lippard SJ. (1999). Structure, recognition, and processing of cisplatin-DNA adducts. Chem Rev 99:2467–98
- Lee SB, Kalluri R. (2010). Mechanistic connection between inflammation and fibrosis. Kidney Int 78:S22–6
- Li X, Wang H. (2005). Chinese herbal medicine in the treatment of chronic kidney disease. Adv Chronic Kidney Dis 12:276–81
- Lieberthal W, Triaca V, Levine J. (1996). Mechanisms of death induced by cisplatin in proximal tubular epithelial cells: Apoptosis vs. necrosis. Am J Physiol 270:F700–8
- Lin B. (2011). Polyphenols and neuroprotection against ischemia and neurodegeneration. Mini Rev Med Chem 11:1222–38
- Liu Y. (2006). Renal fibrosis: New insights into the pathogenesis and therapeutics. Kidney Int 69:213–17
- Liu Y. (2010). New insights into epithelial-mesenchymal transition in kidney fibrosis. J Am Soc Nephrol 21:212–22
- Low Dog T. (2005). Menopause: A review of botanical dietary supplements. Am J Med 118:98–108
- Megyesi J, Andrade L, Vieira JM Jr, et al. (2002). Coordination of the cell cycle is an important determinant of the syndrome of acute renal failure. Am J Physiol Renal Physiol 283:F810–16
- Meng LQ, Tang JW, Wang Y, et al. (2011). Astragaloside IV synergizes with ferulic acid to inhibit renal tubulointerstitial fibrosis in rats with obstructive nephropathy. Br J Pharmacol 162:1805–18
- Nagwani S, Tripathi YB. (2010). Amelioration of cisplatin induced nephrotoxicity by PTY: A herbal preparation. Food Chem Toxicol 48:2253–8
- Nitha B, Janardhanan KK. (2008). Aqueous-ethanolic extract of morel mushroom mycelium Morchella esculenta, protects cisplatin and gentamicin induced nephrotoxicity in mice. Food Chem Toxicol 46:3193–9
- Pabla N, Dong Z. (2008). Cisplatin nephrotoxicity: Mechanisms and renoprotective strategies. Kidney Int 73:994–1007
- Pozdzik AA, Salmon IJ, Debelle FD, et al. (2008). Aristolochic acid induces proximal tubule apoptosis and epithelial to mesenchymal transformation. Kidney Int 73:595–607
- Ruszymah BH, Chowdhury SR, Manan NA, et al. (2012). Aqueous extract of Centella asiatica promotes corneal epithelium wound healing in vitro. J Ethnopharmacol 140:333–8
- Ryan MJ, Johnson G, Kirk J, et al. (1994). HK-2: An immortalized proximal tubule epithelial cell line from normal adult human kidney. Kidney Int 45:48–57
- Sohn SH, Lee H, Nam JY, et al. (2009). Screening of herbal medicines for the recovery of cisplatin-induced nephrotoxicity. Environ Toxicol Pharmacol 28:206–12
- Urruticoechea A, Smith IE, Dowsett M. (2005). Proliferation marker Ki-67 in early breast cancer. J Clin Oncol 23:7212–20
- Valcu M, Valcu CM. (2011). Data transformation practices in biomedical sciences. Nat Methods 8:104–5
- Wang H, Li J, Yu L, et al. (2004). Antifibrotic effect of the Chinese herbs, Astragalus mongholicus and Angelica sinensis, in a rat model of chronic puromycin aminonucleoside nephrosis. Life Sci 74:1645–58
- WHO. (2004). WHO Monographs on Selected Medicinal Plants. Geneva: World Health Organization
- Wojcikowski K, Wohlmuth H, Johnson DW, et al. (2009). An in vitro investigation of herbs traditionally used for kidney and urinary system disorders: Potential therapeutic and toxic effects. Nephrology 14:70–9
- Wynn TA. (2007). Common and unique mechanisms regulate fibrosis in various fibroproliferative diseases. J Clin Invest 117:524–9
- Wynn TA. (2010). Fibrosis under arrest. Nat Med 16:523–5
- Xu Q, Norman JT, Shrivastav S, et al. (2007). In vitro models of TGF-beta-induced fibrosis suitable for high-throughput screening of antifibrotic agents. Am J Physiol Renal Physiol 293:F631–40
- Yan R, Ko NL, Li SL, et al. (2008). Pharmacokinetics and metabolism of ligustilide, a major bioactive component in Rhizoma Chuanxiong, in the rat. Drug Metab Dispos 36:400–8
- Yang L, Besschetnova TY, Brooks CR, et al. (2010). Epithelial cell cycle arrest in G2/M mediates kidney fibrosis after injury. Nat Med 16:535–43. 1 p following 143
- Yang WJ, Li DP, Li JK, et al. (2009). Synergistic antioxidant activities of eight traditional Chinese herb pairs. Biol Pharm Bull 32:1021–6
- Yao X, Panichpisal K, Kurtzman N, Nugent K. (2007). Cisplatin nephrotoxicity: A review. Am J Med Sci 334:115–24
- Ye YN, Koo MW, Li Y, et al. (2001). Angelica sinensis modulates migration and proliferation of gastric epithelial cells. Life Sci 68:961–8
- Yu F, Li H, Meng Y, Yang D. (2013). Extraction optimization of Angelica sinensis polysaccharides and its antioxidant activity in vivo. Carbohydr Polym 94:114–19
- Zeisberg M, Duffield JS. (2010). Resolved: EMT produces fibroblasts in the kidney. J Am Soc Nephrol 21:1247–53
- Zeisberg M, Neilson EG. (2009). Biomarkers for epithelial-mesenchymal transitions. J Clin Invest 119:1429–37