Abstract
Context: Antidiabetic activity of Coptis chinensis Franch (Ranunculaceae) polysaccharide (CCPW) has been reported. However, its molecular mechanism remains unclear.
Objective: An attempt was made to further verify the antidiabetic activity of CCPW on type 2 diabetes mellitus (T2DM) and elucidate the mechanism of antidiabetic activity.
Materials and methods: Male Wistar rats were fed with high-fat diet (HFD) and injected with streptozotocin (STZ) to generate a T2DM model. Effects of CCPW on fasting blood glucose (FBG), triglyceride (TG), total cholesterol (TC), glutathione (GSH), glutathione peroxidases (GSH-Px), superoxide dismutases (SOD), catalase (CAT), malondialdehyde (MDA), c-jun n-terminal kinase (JNK), phosphorylated insulin receptor substrate 1 (phospho-IRS1), phosphorylated phosphatidylinositol 3 kinase (phospho-PI3Kp85) and glucose transporter 4 (Glut4) were investigated.
Results: FBG level of diabetic rats could be significantly inhibited by 51.2, 42.7, and 23.3% through administration of CCPW at doses of 200, 100, and 50 mg/kg b.w., respectively (p < 0.01). CCPW also could significantly reduce TG by 19.2, 12.1, and 7.4%, and TC by 24.2, 20.9, and 18.7%, respectively (p < 0.05 or p < 0.01). CCPW showed an obvious antioxidant effect through increasing GSH-Px, SOD, and CAT activities, and decreasing GSH and MDA contents (p < 0.05 or p < 0.01). Furthermore, CCPW could inhibit JNK and phospho-IRS1 expression and promote the expression of phospho-PI3Kp85 and Glut4 compared with those in the DM group (p < 0.05 or p < 0.01).
Discussion and conclusion: CCPW can produce antidiabetic activity in rats with T2DM through its antioxidative effect, which is closely related to the JNK/IRS1/PI3K pathway.
Introduction
There has been a sharp increase in diabetes across the world. It is estimated that the number of diabetics will rise to 380 million worldwide by 2025, and 95% of those people will have T2DM (Zimmet et al., Citation2001). Accumulating evidence demonstrates that T2DM is one of the most important diseases threatening human health after cancer and cardiovascular diseases (Peter, Citation2000). The morbidity of T2DM is especially poor due to the combination of elevated living standards, decreased physical work, unhealthy lifestyles, and enlarged number of the elderly nowadays. T2DM is a multi-factorial disease characterized by hyperglycemia resulting from defects of insulin secretion and insulin action, which leads to impaired functions in carbohydrate, lipid, and protein metabolism (Kahn, Citation2003). In spite of the application of plentiful hypoglycemic agents in clinic, there is no effective drug for diabetes due to severe toxicity and side effects, inconvenience, and unstable efficacy (Lee et al., Citation2003; Moon et al., Citation2004).
Coptis chinensis Franch (Ranunculaceae), also known as huanglian in Chinese, one of the most widely used traditional Chinese medicines, has attracted much attention because of its multiple pharmacological effects, such as antidiabetic, anti-inflammation, and anticancer (Prieto et al., Citation2003; Wang et al., Citation2011; Yuan et al., Citation2006). Our previous study confirmed that CCPW could have beneficial physiological effects on DM and its complications (Jiang et al., Citation2013). However, the molecular mechanism of CCPW in the treatment of diabetes mellitus remains unclear. In this study, a rat T2DM model was established using HFD and STZ, and an attempt was made to further verify the antidiabetic activity of CCPW on T2DM and elucidate the mechanism of antidiabetic activity in which the antioxidative stress of CCPW should be focused to regulate JNK/IRS1/PI3K pathway.
Materials and methods
Materials and chemicals
Dried Coptis chinensis was purchased from a local medicine market and identified by Prof. Guangxin Yuan (College of Pharmacy, Beihua University) according to the identification standards of Pharmacopeia of the People's Republic of China. CCPW was isolated and purified by a series of water extraction and alcohol precipitation procedures (Jiang et al., Citation2013). CCPW obtained with the above methods was a non-reducibility polysaccharide, in which the content of the total sugar was 88.73%, the content of uronic acid was 29.92%, and the content of protein was 2.85%. STZ was purchased from Sigma Chemical Co. (St. Louis, MO). TG, TC, NEFA/FFA, GSH, GSH-Px, SOD, CAT, and MDA test kits were obtained from Huili Biotech Co. (Changchun, Jilin, China). RT-PCR test kit was gained from Takara Biotech Co. (Otsu, Japan) and antibodies of JNK, IRS1, phospho-IRS1 (Ser307), PI3K, phospho-PI3Kp85, and Glut4 were purchased from Cell Signaling Technology (Beverly, MA). All other reagents were of analytical grade from Sinopharm Chemical Reagent Co. (Beijing, China).
Experimental animals
Male Wistar rats (200–250 g) were purchased from the Experimental Animal Breeding Centre, Jilin University (Changchun, Jilin, China). All rats were housed in an air-conditioned room at 22 ± 1 °C, with a humidity of 50 ± 10%, and a constant 12 h light and 12 h dark cycle. They were fed with diet and given tap water ad libitum. Normal-pellet diet (NPD), consisting of 5% fat, 53% carbohydrate, 23% protein, with total calorific value 25.0 kJ/kg, and HFD, consisting of 22% fat, 48% carbohydrate, 20% protein with total calorific value 44.3 kJ/kg, were ordered from the Experimental Animal Breeding Centre, Jilin University. All experimental animals were overseen and approved by the Animal Care and Use Committee of Jilin University before and during experiments.
Preparation for HFD/STZ-induced diabetic rats
The rats were randomly divided into two groups. One group (n = 8, normal control, NC) was fed with NPD and the other group (n = 60) was fed with HFD. After 4 weeks, the rats fed with HFD were injected intraperitoneally with STZ dissolved in citrate buffer (pH 4.5) at the dose of 30 mg/kg b.w. and FBG levels were measured on week 4 after the injection. The rats fed with NPD were injected intraperitoneally with the citrate buffer vehicle. The HFD-fed rats with FBG value above 7.8 mmol/L were randomly divided into four groups (n = 8 each) and were fed with HFD continuously. One group was used as the DM group, and the other three groups were orally gavaged with CCPW dissolved in normal saline at the doses of 200 (HCCPW), 100 (MCCPW), and 50 (LCCPW) mg/kg b.w. per day, respectively. Rats in the normal control and diabetic control groups were gavaged with normal saline. The bodyweight was recorded weekly.
On the 28th day of the experiment, the animals were deprived of food overnight before being sacrificed. Blood samples obtained from tails were separated by centrifugation for 5 min and kept at −80 °C. The hepatic tissue was removed promptly, and stored in liquid nitrogen for future use.
FBG and oral glucose tolerance test
Oral glucose tolerance test (OGTT) was conducted in control and treated rats, 24 h before they were sacrificed. After overnight fast, blood samples were collected from the veins of rat tails to obtain baseline blood glucose levels. Subsequently, all the rats in groups were gavaged with 2 g/kg b.w. of glucose solution (40%, wt/vol). FBG levels were measured at 0, 30, 60, 90, and 120 min by the glucose oxidase method according to instructions of the kit.
Estimation of lipid and NEFA/FFA profiles in blood samples
Approximately 24 h after completion of FBG and OGTT, the rats were sacrificed and their blood samples were collected from their tails. The lipid and NEFA/FFA profiles for all groups were measured by an enzyme-linked immunosorbent assay (ELISA) kit (Huili Biotech Co., Changchun, Jilin, China).
SH-Px, SOD, CAT activities, and GSH, MDA contents in hepatic tissue
GSH-Px, SOD, CAT activities, and GSH, MDA contents in hepatic tissue were also measured by commercially available kits as described earlier (Huili Biotech Co., Changchun, Jilin, China) (Jurczuk & Brzóska, Citation2004).
Semi-quantitative RT-PCR analysis
Total RNA was prepared with the TRIzol reagent according to the manufacturer’s instructions. RNA was treated with DNase, purified by ethanol precipitation, resuspended in DEPC-treated water, and stored at −80 °C. RNA sample concentration was measured with a spectrophotometer. The total RNA quality was estimated by formaldehyde gel electrophoresis. Semi-quantitative RT-PCR for JNK, Glut4, and GAPDH was performed using a standard procedure and primer sequences were as follows: for JNK: forward, 5′-ACAGTGAGCAGAGCAGGCATAGTG-3′ and reverse, 5′-TCCTCCCCAAACAAAATAGAACCA-3′, for Glut4: forward, 5′-AACCTGTTCCAACAACGGAGGA-3′ and reverse, 5′-AGACCATTGAACAAGGCACCAA-3′, for GAPDH: forward, 5′-GTATGACTCTACCCACGGCAAGT-3′ and reverse, 5′-TGGCCCCACCCTTCAGGTGAGC-3′.
Western blot analysis
Protein levels of JNK, IRS1, phospho-IRS1, PI3K, phospho-PI3Kp85, and Glut4 in hepatic tissue were assayed by western blot analysis. Hepatic tissue from control and experimental rats were homogenized and lysed in ice-cold RIPA lysis buffer containing a protease inhibitor. The supernatant protein concentration was measured by the Bradford assay. The protein was separated by SDS-PAGE using a 12% gel and transferred to a PVDF membrane. The membrane was blocked with 5% non-fat milk, and then incubated with anti-JNK, anti-IRS1, anti-phospho-IRS1 (Ser307), anti-PI3K, anti-phospho-PI3Kp85, and anti-Glut4 primary antibodies. After washing with TBST, the membrane was incubated with the secondary antibody. An enhanced chemiluminescence kit was used to detect the bands. GAPDH was chosen as an internal control. Proteins were quantitatively determined by densitometry using Image J from NIH website.
Statistical analysis
All results were expressed as means ± standard deviations (SD) for each group. Statistical calculation was done by SPSS version 13.0 software (SPSS Inc., Chicago, IL). The significance of the difference between the means of test and control studies was established by Student’s t-test. p Values of less than 0.05 or 0.01 were considered significant.
Results
CCPW-induced increase in bodyweight of diabetic rats
Male Wistar rats were fed with HFD for 4 weeks and then injected with STZ followed by continual HFD-fed to generate a non-genetic rodent model mimicking human T2DM (Benny et al., Citation2005; Srinivasan et al., Citation2005). There was a significant difference in bodyweight between the group of HFD-fed and NPD-fed (p < 0.01). FBG level in the HFD group increased, but there was no significant difference compared with that in the NPD group (p > 0.05). The analysis of TG and TC showed a significant increase corresponding with the high-fat diet (p < 0.05) ().
Table 1. Glycolipid metabolic indicators in NPD and HFD-fed rats for 4 weeks.
The rats treated with HFD/STZ in the DM group had a greater bodyweight loss than those in the NC group, which was consistent with the clinical symptoms of patients with diabetes mellitus. After treatment with CCPW, bodyweight of all rats administrated at doses of 200 (HCCPW), 100 (MCCPW), and 50 (LCCPW) mg/kg b.w. significantly increased compared with those in the DM group (p < 0.05 or p < 0.01). Therefore, CCPW could dose dependently increase the bodyweight in diabetic rats ().
CCPW showed antidiabetic activity in diabetic rats
The antidiabetic activity of CCPW in diabetic rats was evaluated through the FBG and OGTT. As seen from , the rats of the NC group had a normal FBG level which was different from that of the DM group (p < 0.01). FBG level of diabetic rats could be significantly inhibited by 51.2%, 42.7%, and 23.3% through administration of CCPW at doses of 200, 100, and 50 mg/kg b.w., respectively, compared with the diabetic control group (p < 0.01) (). In OGTT, the maximum blood glucose levels in NC at 60 min and CCPW-treated groups appeared at 30 min after the oral glucose challenge. The blood glucose level of rats in HCCPW, MCCPW, and LCCPW groups were significantly suppressed although they were still higher than the initial level after the challenge. This suppression effect on the blood glucose level would persist until the blood glucose level reached the initial level. Among CCPW-treated groups, the higher dose-treated rats usually had more potent glucose tolerance than the lower dose-treated rats, which was reduced progressively in the following order: HCCPW > MCCPW > LCCPW, indicating a dose-dependent effect of CCPW on the glucose tolerance in the rats ().
Decreased lipid and NEFA/FFA profiles of diabetic rats with CCPW treatment
To further evaluate the antidiabetic activity of CCPW, we estimated the metabolic indicators associated with T2DM in different groups. The results showed that CCPW at doses of 200, 100, and 50 mg/kg b.w. could significantly reduce TG by 19.2, 12.1, and 7.4%, and TC by 24.2, 20.9, and 18.7%, respectively, compared with the diabetic control group (p < 0.05 or p < 0.01) (). As illustrates, the treatment with CCPW also decreased the level of NEFA/FFA dose dependently in HFD/STZ-induced diabetic rats. NEFA/FFA level in blood samples of diabetic rats were remarkably higher than those in the NC group and when being treated with CCPW, the diabetic rats showed obviously lower NEFA/FFA level compared with those in the DM group (p < 0.01).
Antioxidant effect of CCPW in diabetic rats
In this study, we evaluated the antioxidant effect of CCPW through contents of GSH and MDA and activities of GSH-Px, SOD, and CAT. As shown in , the treatment with CCPW increased the content of GSH and the activity of GSH-Px dose dependently in diabetic rats. GSH content and GSH-Px activity in livers of diabetic rats were higher than those in the NC group and when being treated with CCPW, the diabetic rats showed higher GSH content and GSH-Px activity compared with those in the DM group (p < 0.05 or p < 0.01). As shown in , when treated with CCPW at three doses (200, 100, and 50 mg/kg b.w.), SOD and CAT activities in livers of diabetic rats were remarkably higher than those in the DM group (p < 0.05 or p < 0.01), but lower than those in the normal control group. MDA content in livers of CCPW-treated diabetic rats was lower than that in the DM group (p < 0.01). The results indicated an obvious antioxidant effect of CCPW on diabetic rats, which might be a possible reason for antidiabetic activity.
Table 2. Effects of CCPW on GSH level and GSH-Px activity of diabetic rats.
Table 3. Effects of CCPW on SOD, CAT activity and MDA content of diabetic rats.
Regulation of JNK/IRS1/PI3K pathway by CCPW through its antioxidative effect
In order to further explore the molecular mechanism of CCPW on improving oxidative stress, effects of CCPW on JNK and Glut4 expression at the mRNA level were measured with semi-quantitative RT-PCR. The results showed that the mRNA level of JNK decreased and the mRNA level of Glut4 increased in CCPW treated groups compared with those in the model group (). We also evaluated the effects of CCPW on the expression of JNK, phospho-IRS1 (Ser307), IRS1, phospho-PI3Kp85, PI3K, and Glut4 at protein level with western blot analysis. JNK expression of all rats administrated at the doses of 200, 100, and 50 mg/kg b.w. decreased as compared with those in the DM group (). After CCPW treatment, the hepatic tissue of rats in CCPW-treated groups showed a sharp decrease in phospho-IRS1 (Ser307) and an increase in phospho-PI3Kp85 expression compared with those in the DM group, but the change in the quantity of IRS1 and PI3K could not be found in almost all groups (). CCPW treatment also enhanced Glut4 protein level in diabetic rats (). The results of RT-PCR and western blot analysis indicated that the improvement of oxidative stress by CCPW was closely related to JNK/IRS1/PI3K pathway.
Figure 4. Effects of CCPW on mRNA expression of JNK and Glut4 by semi-quantitative RT-PCR analysis in rats’ hepatic tissue. (A) Effect of CCPW on mRNA expression of JNK; (B) Effect of CCPW on mRNA expression of Glut4. **p < 0.01 versus the DM group.
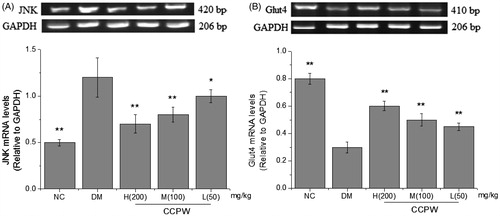
Figure 5. Effects of CCPW on protein expression of JNK, phospho-IRS1 (Ser307), IRS1, phospho-PI3Kp85, PI3K, and Glut4 by western blot analysis in rat hepatic tissue. (A) Effect of CCPW on protein expression of JNK; (B) effects of CCPW on protein expression of phospho-IRS1 (Ser307) and IRS1; (C) effects of CCPW on protein expression of phospho-PI3Kp85 and PI3K; (D) effects of CCPW on protein expression of Glut4. **p < 0.01 versus the DM group.
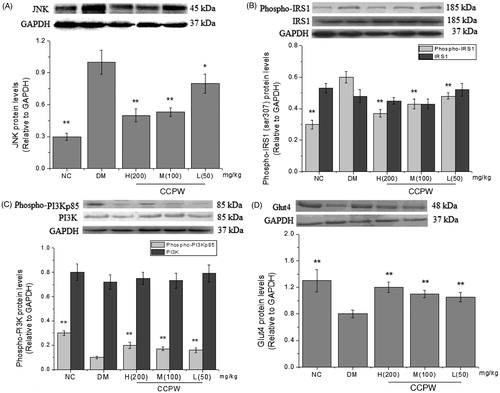
Discussion
Polysaccharide is a kind of natural macromolecule composed of monosaccharides connected by glycosidic bonds. Since the molecules are mainly composed of carbon and oxygen, it is also known as carbohydrates. Polysaccharide is sourced from plants, animals, and microorganisms. As a basic life material, polysaccharides are the most abundant biopolymers in nature. Polysaccharide offers many biological effects such as structural support, protective function, and energy storage. A plethora of experimental results have shown that polysaccharides possess the properties of immunoregulation, anticancer, antioxidation, antifatigue, and lowering blood sugar, cholesterol, and blood lipid (Chen & Qi, Citation2013; Chen et al., Citation2008; Jiang et al., Citation2008; Yuan et al., Citation2008). It is a popular topic in the medical field around the world due to its low toxicity, fewer side effects and high impact on the body (Arumugam et al., Citation2008; Chi et al., Citation2008). CCPW was extracted from the roots of dried Coptis chinensis and the hypoglycemic and blood–lipid lowering activities were confirmed in diabetic mice. However, the mechanism of CCPW in the treatment of diabetes mellitus remains unclear.
It has been proposed recently that oxidative stress may be a primary factor in the etiology of T2DM and its complications (Bandeira et al., Citation2012; Folli et al., Citation2011). It has been a long history since people knew that the progression of several diseases and pathologies was greatly dependent on the modulation of oxidative stress in mammalian systems (Berlett & Stadtman, Citation1997; Finkel & Holbrook, Citation2000). The consumption of adipogenic diets and the accumulation of adipose tissue could significantly increase levels of oxidative stress in mammalian systems. Abundant clinical evidence demonstrated that diabetes correlated closely with oxidative stress, resulting in an increased reactive oxygen species (ROS) production or a reduction in the antioxidant defense system (Susztak et al., Citation2006). Oxidative stress could also have a significant inhibitory effect on insulin signaling in both in vitro and cell culture systems (Dokken et al., Citation2008; Gardner et al., Citation2003; Maechler et al., Citation1999). In this study, we established a non-genetic rodent model mimicking human T2DM through feeding with HFD while giving a STZ injection at the end of the fourth week. Increased FBG and lipid levels and oxidative stress were shown in diabetic rats. After CCPW treatment, CCPW could reduce FBG level and improve glucose tolerance. CCPW could also decrease the content of the lipid and NEFA/FFA profiles in blood samples of diabetic rats. Furthermore, CCPW showed an obvious antioxidant effect through increasing the activities of GSH-Px, SOD, and CAT and decreasing the content of GSH and MDA in livers. These results indicated a clear antioxidant activity of CCPW in diabetic rats, which might be a possible reason for its antidiabetic activity.
It is still not exactly clear how oxidative stress causes T2DM or metabolic malfunction although several mechanisms have been proposed (Savu et al., Citation2012). The most common mechanism is that oxidative stress stimulates stress signaling mitogen-activated protein kinases (MAPKs), such as JNK, and then in turn causes inhibition of insulin signaling. The important role of ROS to activate JNK in the etiology of T2DM has been well accepted (Hotamisligil, Citation2006). The activation of JNK has been shown to directly phosphorylate IRS1 at inhibitory sites that prevent recruitment of this protein to the activated insulin receptor (Aguirre et al., Citation2000, Citation2002). Thus, JNK-mediated IRS1 phosphorylation disrupts downstream events such as PI3K and Glut4 in the insulin-signaling pathway leading to diabetes mellitus (Draznin, Citation2006; Taniguchi et al., Citation2006). As might be predicted by the strong inhibitor effect of JNK on insulin signaling, mice lacking JNK showed an improved glucose tolerance and insulin sensitivity compared with control mice when fed on a high-fat diet (Hirosumi et al., Citation2002). In order to further explore the molecular mechanism of CCPW on antidiabetic activity, effects of CCPW on JNK, phospho-IRS1 (Ser307), IRS1, phospho-PI3Kp85, PI3K, and Glut4 expression were measured. The results showed that compared with the model group, CCPW could inhibit JNK expression and improve Glut4 at the mRNA level, inhibit JNK and phospho-IRS1 (Ser307) expression, and improve the expression of PI3Kp85 and Glut4 at the protein level, but could not affect the expression of IRS1 and PI3K obviously. Many studies have demonstrated that oxidative stress is closely related to diabetes and insulin resistance (Lucchesi & Freitas, Citation2013; Venkatasamy et al., Citation2013). Oxidative stress can promote the production of JNK, thus make IRS1 phosphorylated, and finally result in diabetes mellitus (Kaneto et al., Citation2005, Citation2007). It was verified in our experiments that CCPW could reduce the expression of JNK through its antioxidative stress and then inhibit the phosphorylation of IRS1 (Ser307); the inhibition of CCPW on the phosphorylation of IRS1 could increase PI3Kp85 expression and then increase the expression of Glut4 by affecting the whole pathway of PI3K.
In conclusion, CCPW can produce antidiabetic activity in rats with T2DM through its antioxidative effect, which is closely related to JNK/IRS1/PI3K pathway.
Declaration of interest
This work was funded by the project of Education Department of Jilin Province (2014203), Health Department of Jilin Province (2013Q013), Administration of traditional Chinese Medicine of Jilin Province (2012163), and supported by Development and Reform Commission of Jilin Province.
References
- Aguirre V, Uchida T, Yenush L. (2000). The c-jun NH2-terminal kinase promotes insulin resistance during association with insulin receptor substrate-1 and phosphorylation of Ser307. J Biol Chem 275:9047–54
- Aguirre V, Werner ED, Giraud J. (2002). Phosphorylation of Ser307 in insulin receptor substrate-1 blocks interactions with the insulin receptor and inhibits insulin action. J Biol Chem 277:1531–7
- Arumugam S, Hanumantha RBR, Periasamy S. (2008). Anti-peroxidative and anti-hyperlipidemic nature of Ulva lactuca crude polysaccharide on d-galactosamine induced hepatitis in rats. Food Chem Toxicol 46:3262–7
- Bandeira SM, Guedes GS, Fonseca LJ. (2012). Characterization of blood oxidative stress in type 2 diabetes mellitus patients: Increase in lipid peroxidation and SOD activity. Oxid Med Cell Longev 10:81–93
- Benny K, Huat T, Chee HT. (2005). Antidiabetic activity of the semi-purified fractions of Averrhoa bilimbi in high fat diet fed-streptozotocin-induced diabetic rats. Life Sci 76:2827–39
- Berlett BS, Stadtman ER. (1997). Protein oxidation in aging, disease, and oxidative stress. J Biol Chem 272:20313–16
- Chen H, Qi X. (2013). Study on the effect of polysaccharides from Solanum nigrum Linne on cellular immune function in tumour-bearing mice. Afr J Tradit Complement Altern Med 10:41–6
- Chen H, Zhang M, Qu Z. (2008). Antioxidant activities of different fractions of polysaccharide conjugates from green tea (Camellia sinensis). Food Chem 10:559–63
- Chi AP, Chen JP, Wang ZZ. (2008). Morphological and structural characterization of a polysaccharide from Gynostemma pentaphyllum Makino and its anti-exercise fatigue activity. Carbohydr Polym 74:868–74
- Dokken BB, Saengsirisuwan V, Kim JS. (2008). Oxidative stress-induced insulin resistance in rat skeletal muscle: Role of glycogen synthase kinase-3. Am J Physiol Endocrinol Metab 294:615–21
- Draznin B. (2006). Molecular mechanisms of insulin resistance: Serine phosphorylation of insulin receptor substrate-1 and increased expression of p85a: The two sides of a coin. Diabetes 55:2392–7
- Finkel T, Holbrook NJ. (2000). Oxidants, oxidative stress and the biology of ageing. Nature 408:239–47
- Folli F, Corradi D, Fanti P. (2011). The role of oxidative stress in the pathogenesis of type 2 diabetes mellitus micro- and macrovascular complications: Avenues for a mechanistic-based therapeutic approach. Curr Diabetes Rev 7:313–24
- Gardner CD, Eguchi S, Reynolds CM. (2003). Hydrogen peroxide inhibits insulin signaling in vascular smooth muscle cells. Exp Biol Med 228:836–42
- Hirosumi J, Tuncman G, Chang L. (2002). A central role for JNK in obesity and insulin resistance. Nature 420:333–6
- Hotamisligil GS. (2006). Inflammation and metabolic disorders. Nature 444:860–7
- Jiang S, Du P, An L. (2013). Antidiabetic effect of Coptis chinensis polysaccharide in high-fat diet with STZ-induced diabetic mice. Int J Biol Macromol 55:118–12
- Jiang YH, Jiang XL, Wang P. (2008). The antitumor and antioxidative activities of polysaccharides isolated from Isaria farinosa B05. Microbiol Res 16:424–30
- Jurczuk M, Brzóska MM. (2004). Antioxidant enzymes activity and lipid peroxidation in liver and kidney of rats exposed to cadmium and ethanol. Food Chem Toxicol 42:429–38
- Kahn SE. (2003). The relative contributions of insulin resistance and beta-cell dysfunction to the pathophysiology of type 2 diabetes. Diabetologia 46:3–19
- Kaneto H, Matsuoka TA, Katakami N. (2007). Oxidative stress and the JNK pathway are involved in the development of type 1 and type 2 diabetes. Curr Mol Med 7:674–86
- Kaneto H, Matsuoka TA, Nakatani Y. (2005). Oxidative stress and the JNK pathway in diabetes. Curr Diabetes Rev 1:65–72
- Lee TH, Kim SU, Yu SL. (2003). Peroxiredoxin II is essential for sustaining life span of erythrocytes in mice. Blood 101:5033–8
- Lucchesi AN, Freitas NT. (2013). Diabetes mellitus triggers oxidative stress in the liver of alloxan-treated rats: A mechanism for diabetic chronic liver disease. Acta Cir Bras 28:502–8
- Maechler P, Jornot L, Wollheim CB. (1999). Hydrogen peroxide alters mitochondrial activation and insulin secretion in pancreatic beta cells. J Biol Chem 274:27905–13
- Moon EY, Han YH, Lee DS. (2004). Reactive oxygen species induced by the deletion of peroxiredoxin II (PrxII) increases the number of thymocytes resulting in the enlargement of PrxII-null thymus. Eur J Immunol 34:2119–28
- Peter G. (2000). Obesity as a medical problem. Nature 404:635–43
- Prieto JM, Recio MC, Giner RM. (2003). Influence of traditional Chinese antiinflammatory medicinal plants on leukocyte and platelet functions. J Pharm Pharmacol 55:1257–82
- Savu O, Ionescu-Tirgoviste C, Atanasiu V. (2012). Increase in total antioxidant capacity of plasma despite high levels of oxidative stress in uncomplicated type 2 diabetes mellitus. J Int Med Res 40:709–16
- Srinivasan K, Viswanad B, Ramarao P. (2005). Combination of high-fat diet-fed and low-dose streptozotocin-treated rat: A model for type 2 diabetes and pharmacological screening. Pharmacol Res 52:313–20
- Susztak K, Raff AC, Schiffer M. (2006). Glucose-induced reactive oxygen species cause apoptosis of podocytes and podocyte depletion at the onset of diabetic nephropathy. Diabetes 55:225–33
- Taniguchi CM, Emanuelli B, Kahn CR. (2006). Critical nodes in signaling pathways: Insights into insulin action. Nat Rev Mol Cell Biol 7:85–96
- Venkatasamy VV, Pericherla S, Manthuruthil S. (2013). Effect of physical activity on insulin resistance, inflammation and oxidative stress in diabetes mellitus. J Clin Diagn Res 7:1764–6
- Wang H, Zhang F, Ye F. (2011). The effect of Coptis chinensis on the signaling network in the squamous carcinoma cells. Front Biosci 1:326–40
- Yuan JF, Zhang ZQ, Fan ZC. (2008). Antioxidant effects and cytotoxicity of three purified polysaccharides from Ligusticum chuanxiong Hort. Carbohydr Polym 74:822–7
- Yuan LJ, Tu DW, Ye XL. (2006). Hypoglycemic and hypocholesterolemic effects of Coptis chinensis franch inflorescence. Plant Foods Hum Nutr 61:139–44
- Zimmet P, Alberti K, Shaw GJ. (2001). Global and societal implications of the diabetes epidemic. Nature 414:782–7