Abstract
Context: Nerium indicum Mill. (Apocynaceae) was reported for its efficient in vitro antioxidant and iron-chelating properties.
Objective: This study demonstrates the effect of 70% methanol extract of N. indicum leaf (NIME) towards in vitro DNA protection and ameliorating iron-overload-induced liver damage in mice.
Materials and methods: Phytochemical and HPLC analyses were carried out to standardize the extract and the effect of Fe2+-mediated pUC18 DNA cessation was studied. Thirty-six Swiss Albino mice were divided into six groups of blank, negative control (iron overload only), and iron-overloaded mice receiving 50, 100, and 200 mg/kg b.w. doses of NIME and desirox (20 mg/kg b.w.). The biochemical markers of hepatic damage, various liver and serum parameters, and reductive release of ferritin iron were studied.
Results and discussion: The presence of different phytocomponents was revealed from phytochemical and HPLC analyses. A substantial supercoiled DNA protection, with [P]50 of 70.33 ± 0.32 µg, was observed. NIME (200 mg/kg b.w.) significantly normalized the levels of alanine aminotransferase, aspartate aminotransferase, alkaline phosphatase, and bilirubin by 126.27, 125.25, 188.48, and 45.47%, respectively. NIME (200 mg/kg b.w.) was shown to alleviate the reduced levels of superoxide dismutase, catalase, glutathione-S-transferase, and non-enzymatic-reduced glutathione, by 48.95, 35.9, 35.42, and 13.22%, respectively. NIME also lowered raised levels of lipid peroxidation, protein carbonyl, hydroxyproline, and liver iron by 32.28, 64.58, 136.81, and 83.55%, respectively.
Conclusion: These findings suggest that the active substances present in NIME may be capable of lessening iron overload-induced toxicity, and possibly be a useful drug for iron-overloaded diseases.
Introduction
Liver is one of the most vital organs of the human body and is a center of nutrient metabolism and waste metabolite excretion (Anusha et al., Citation2011). Hepatic damage is associated with distortion of these metabolic functions (Wolf, Citation1999) and sometimes results in serious health problems. Hepatotoxicity is the most common finding in patients with iron overload since the liver is involved in taking up and storing excessive amounts of iron (Papanastasiou et al., Citation2000). Iron, the most abundant transition metal, is essential for growth and survival of all mammalian cells. Although an optimum level of iron is always maintained by cells to balance between essentiality and toxicity, in some situations the balance is disrupted which results in iron-overloaded toxicity. This is probably via the formation of reactive oxygen species, including highly reactive hydroxyl radicals through Haber–Weiss reactions that can mediate injury to various biomolecules leading to oxidative stress (Halliwell & Gutteridge, Citation1984). This leads to oxidative damage of proteins, lipids, and nucleic acids, thereby resulting in several human diseases such as hepatocellular necrosis (Olynyk et al., Citation1995), inflammation (Deugnier et al., Citation1992), fibrosis (Gardi et al., Citation2002), and even cancer (Niederau et al., Citation1985). However, several iron-chelating agents such as deferoxamine, 1,2-dimethyl-3-hydroxypyrid-4-one (deferiprone, L1), and deferasirox are currently available for clinical use and act as iron chelators to takedown excessive iron from the patient’s body. These drugs also come with various side effects and disadvantages (Al-Refaie et al., Citation1992; Kontoghiorghes, Citation2003), which ultimately raises the need for the development of a better, safer, and more effective drug from natural resources.
Phytoconstituents including phenolics and flavonoids are the most important representatives that offer alleviation of hepatic ailments. It has been found that most of them are effective antioxidants (López et al., Citation2003; Rice-Evans et al., Citation1996) where iron chelation is a crucial part of their antioxidant activity (Cook & Samman, Citation1996). Thus, the search for crude drugs of plant origin with antioxidant activity has become a primary focus of study on hepatoprotection. Nerium indicum Mill. (Apocynaceae) (syn. N. oleander L. and N. odorum Aiton) is widely distributed in the Mediterranean region, subtropical Asia, and the southwestern United States of America. Parts of N. indicum have been used medicinally in the treatment of swelling, leprosy, skin diseases as a heart tonic, diuretic (Chopra et al., Citation1956; Manjunath, Citation1966) and are reported to possess a wide range of biological activities including cardiotonic, antibacterial, cytotoxic, antiplatelet aggregation, and central nervous system-depressant activities (Siddiqui et al., Citation1995, Citation1997; Zia et al., Citation1995). It was reported that the extracts of N. indicum and oleandrin show anticancer activity in a species-specific manner (Pathak et al., Citation2000).
Our previous study reported that the 70% methanol extract of N. indicum leaf has shown its antioxidant and iron-chelating properties, in vitro, and was found to be a rich source of phenolic and flavonoid compounds (Dey et al., Citation2012). Based on these observations, the present study was undertaken to assess whether the 70% methanol extract of N. indicum leaf (NIME) can normalize the damage caused to a liver by iron overload in Swiss albino mice.
Materials and methods
Chemicals
Iron–dextran and guanidine hydrochloride were purchased from Sigma-Aldrich, St. Louis, MO. Trichloroacetic acid (TCA), nitro blue tetrazolium (NBT), reduced nicotinamide adenine dinucleotide (NADH), phenazine methosulfate (PMS), ferrozine, glutathione reduced, bathophenanthroline sulfonate disodium salt, thiobarbituric acid (TBA), and 5,5′-dithiobis-2-nitrobenzoic acid (DTNB) were obtained from Sisco Research Laboratories Pvt. Ltd, Mumbai, India. Hydrogen peroxide, ammonium iron (II) sulfate hexahydrate [(NH4)2Fe(SO4)26H2O], 1-chloro-2,4-dinitrobenzene (CDNB), chloramine-T, hydroxylamine hydrochloride, dimethyl-4-aminobenzaldehyde, and 2,4-dinitro phenylhydrazin (DNPH) were obtained from Merck, Mumbai, India. Ferritin was purchased from MP Biomedicals, Santa Ana, CA. Streptomycin sulfate was obtained from HiMedia Laboratories Pvt. Ltd, Mumbai, India. The standard oral iron-chelating drug, desirox, with the parent group Deferasirox, was obtained from Cipla Ltd., Kolkata, India.
Plant material
White flowered variety of N. indicum was collected from the campus area of University of North Bengal, India, during the month of August 2011. The plant was identified by taxonomist Prof. A. P. Das of Department of Botany, University of North Bengal and the herbarium sheet of the sample plant was stored at the Botany Department Herbarium, University of North Bengal with the accession number of 9618.
Animals
Male Swiss albino mice (20 ± 2 g) were purchased from Chittaranjan National Cancer Institute (CNCI), Kolkata, India, and were maintained under a constant 12 h dark/light cycle at an environmental temperature of 22 ± 2 °C and provided with a normal laboratory pellet diet and water ad libitum. All experiments were performed after obtaining approval from the Institutional Animal Ethics Committee, with certified regulations of the Committee for the Purpose of Control and Supervision of Experiments on Animals (CPCSEA), Ministry of Environment and Forest, Govt. of India (Bose Institute Registration no. 95/1999/CPCSEA).
Plant extract preparation
Powder (100 g) of the shade-dried leaves of N. indicum was mixed with 70% methanol in water (1000 ml) and stirred using a magnetic stirrer for 15 h; the mixture was then centrifuged at 2850g and the supernatant was decanted. The process was repeated by adding the same solvent with the precipitated pellet. The supernatants from two phases were mixed, concentrated in a rotary evaporator, and lyophilized. The obtained dried extract was stored at −20 °C until use.
Phytochemical analysis and standardization of the leaf extract
Phytochemical analysis of NIME was carried out for carbohydrates, alkaloids, tannins, terpenoids, triterpenoids, anthraquinones, saponins, and glycosides using standard qualitative methods as described previously (Harborne & Baxter, Citation1995; Kokate et al., 1997).
NIME was then subjected for quantification of carbohydrates (Sadasivam & Manickam, Citation1997), alkaloids (Singh et al., Citation2004), ascorbic acid (Roe, Citation1961), and tannins (Robert, Citation1971) following standard procedures.
HPLC standardization of the leaf extract
For HPLC analysis, stock solutions (10 µg/ml) were prepared in the mobile phase for the sample and different standard phytocompounds. Samples were then filtered through a 0.45 µm polytetrafluoroethylene (PTFE) filter (Millipore, Billerica, MA) to remove any particulate matter. Analysis was performed using a HPLC-Prominence System RF10AXL (Shimadzu Corp.) equipped with degasser (DGU-20A5), quaternary pump (LC-20AT), auto-sampler (SIL-20A) and detectors of reflective index (RID-10A), fluorescence (RF-10AXL), and diode array (SPD-M20A). The injection volume used was 20 µl, and the sample and standards were analyzed in triplicates. Gradient elution consecutive mobile phases of acetonitrile and 0.5 mM ammonium acetate in water, at a flow rate of 1 ml/min for 80 min through the column (Z1C-HILIC) were maintained at 25 °C. The detection was carried out at 254 nm.
In vitro study
Protection of Fe2+ mediated DNA breakdown
The DNA protection was studied using supercoiled pUC18 plasmid DNA according to an earlier reported method (Hermes-Lima et al., Citation1998), with minor modifications. In Hepes buffer (pH 7.2, 13 mM), FeSO4 solution (15 μM), NIME of varying doses (0–150 μg), DNA (1 μg), and water were added to make an initial reaction mixture and then H2O2 solution (0.0125 mM) was added to start the reaction. After 10 min, the reaction was stopped by adding desferal (0.2 mM) as a stopping reagent followed by loading buffer. Each reaction mixture (20 µl) was loaded in 1% agarose gel. After migration, the gel was stained with ethidium bromide and visualized in a UV transilluminator. The DNA bands were quantified through densitometry and the following formula were used to calculate the percentage of protection:
where SC is the supercoiled; OC is the open circular; 1.4 is the correction factor
The ability of the plant extract to protect the DNA supercoil can be expressed by the concentration of sample required for 50% protection, designated as the [P]50 value.
In vivo study
Experimental design
Mice were randomly divided into six groups comprising six mice in each group. One group received normal saline only and was labeled as blank (B). The other five groups were injected intraperitoneally with five doses of iron–dextran of 100 mg/kg b.w. (one dose every 2 d). One iron–dextran group (C) was treated with normal saline and other four groups were orally treated with 50 mg/kg b.w. (S50), 100 mg/kg b.w. (S100), 200 mg/kg b.w. (S200) NIME, and 20 mg/kg b.w. desirox (D), for 21 d starting the day following the first iron–dextran injection.
Sample collection and tissue preparation
After treatment, mice were fasted overnight on the 21st day, anesthetized with ethyl ether and blood was collected by cardiac puncture. Serum from the blood samples was separated using cooling centrifuge and stored at −80 °C until analysis. The liver was removed, washed with ice-cold saline, and divided into three parts. One major portion of the liver was cut, weighed, and homogenized in 10 volume of 0.1 M phosphate buffer (pH 7.4) containing 5 mM EDTA and 0.15 M NaCl and centrifuged at 8000g for 30 min at 4 °C. Protein concentration in the supernatant was estimated according to the Lowry method (Lowry et al., Citation1951) using BSA as a standard and remaining supernatant was then used for further analysis. Another part of the liver sample was weighed and digested using equivolume mixture of sulfuric acid and nitric acid and the iron content was analyzed. The remaining part of the liver sample was used for histopathological studies.
Serum markers and ferritin levels
Alanine amino transferase (ALAT), aspartate amino transferase (ASAT), and billirubin in serum samples were measured using the commercial kits of Merck, Mumbai, India. Serum alkaline phosphatase (ALP) was estimated using the kit supplied by Sentinel Diagnostics, Milano, Italy. Serum ferritin level was measured using enzyme-linked immunosorbent assay kit (Monobind Inc., Lake Forest, CA) according to the manufacturer’s instructions.
Antioxidant enzymes
Superoxide dismutase (SOD) was assayed by measuring inhibition of the formation of blue colored formazan at 560 nm according to the previously reported technique (Kakkar et al., Citation1984). Catalase (CAT) activity was measured by following the decomposition of H2O2 over time at 240 nm according to a method described previously (Bonaventura et al., Citation1972). The level of glutathione-S-transferase (GST) was determined by a previously reported method (Habig et al., Citation1974) based on the formation of GSH–CDNB conjugate and increase in the absorbance at 340 nm. Reduced glutathione (GSH) level was measured spectrophotometrically at 412 nm by a standard method (Ellman, Citation1959).
Lipid peroxidation products
The lipid peroxide levels in liver homogenates were measured in terms of thiobarbituric acid reactive substances (TBARS), as an index of malondialdehyde accumulation according to a formerly reported method (Buege & Aust, Citation1978).
Protein carbonyl content
Protein carbonyl content of the samples was determined according to the previously described method (Reznick & Packer, Citation1994). In brief, 10% w/v streptomycin sulfate was mixed with the sample and then centrifuged at 2800g for 15 min. Then an equal amount of the supernatant and 10 mM DNPH in 2 M HCl was incubated at room temperature for 20 min. After the reaction was completed, 10% cold TCA was added to precipitate the proteins and the precipitates were washed with ethyl acetate–ethanol mixture (1:1) for three times to remove unreacted DNPH. The final protein pellet was dissolved in 1 ml of 6 M guanidine hydrochloride solution and the absorbance was measured at 370 nm, using the molar extinction coefficient of DNPH, ɛ = 2.2 × 10−4/M cm.
Hydroxyproline content
Liver samples were hydrolized with 6 M HCl and hydroxyproline was measured by Ehrlich’s solution according to the method described previously (Bergman & Loxley, Citation1963). Results were calculated after taking absorbance at 558 nm and a standard curve (R2 = 0.9907) of 4-hydroxy-l-proline was prepared. The collagen content was determined by multiplying the amount of total hydroxyproline content in each sample by a factor of 7.69 (Kivirikko et al., Citation1967). Results are expressed as milligrams of collagen per liver (wet weight).
Liver iron
Liver iron was measured according to a formerly reported colorimetric method (Barry & Sherlock, Citation1971). Samples were incubated with bathophenanthroline sulfonate for 30 min at 37 °C and the absorbance was measured at 535 nm.
Histopathological study
The liver samples were excised, washed with normal saline, and processed separately for histological study. Initially, the material was fixed at 10% buffered neutral formalin for 48 h. A paraffin-embedding technique was carried out and sections were taken at 5 μm thicknesses, stained with hematoxylin and eosin, and examined microscopically for histopathological changes.
In vitro ferritin iron release
This assay was performed according to a previously described method (Hynes & Coinceanainn, Citation2002). The release of ferritin iron was measured using the ferrous chelator ferrozine as a chromophore. The reaction mixture contained 200 μg ferritin, 500 μM ferrozine, in 50 mM pH 7.0 phosphate buffer. The reaction was started by the addition of NIME of different amounts (100–500 μg) and the change in absorbance was measured continuously at 560 nm for 20 min. A cuvette containing ferritin, ferrozine, and phosphate buffer but lacking plant extract was used as the reference solution.
Statistical analysis
All data are reported as the mean ± SD of six measurements. Statistical analysis was performed using KyPlot version 2.0 beta 15 (32 bit) and Origin professional 6.0 (SPSS Inc., Chicago, IL). Comparisons among groups were made according to pair t-test. In all analyses, a p value of <0.05 was considered significant.
Results and discussion
Plants are abundant source of phytoconstituents having various pharmacological activities including antioxidant, free radical scavenging, and iron-chelating proficiencies. These iron-chelating agents consist of a range of bidentate, tridentate, and hexadentate ligands in which two, three, and six atoms, respectively, are able to coordinate with iron, forming octahedral complexes (Liu & Hider, Citation2002; Tam et al., Citation2003). With the intention to investigate the underlying principle of traditional usage of the plant as medicine, biologically active phytocomponents present in the plant are needed to be extracted with different solvents. The leaf of this plant possessed a significant amount of phenolics and flavonoids. In this study, we have done qualitative and quantitative determination of several phytoconstituents of NIME, which showed the presence of carbohydrates, tannins, alkaloids, terpenoids, triterpenoids, saponins, glycosides, and ascorbic acid among which alkaloids are present in significant amounts, carbohydrates in moderate, and tannins in insignificant amounts (). HPLC analysis was then performed to identify the presence of bioactive compounds by comparing the retention time of reference compounds under the same condition. Four main peaks having retention times 10.82, 14.14, 18.42, and 19.93 min appeared on the HPLC chromatogram that was found to correspond to ellagic acid, methyl gallate, catechin, and reserpine, respectively (). As evident from the phytochemical screening and HPLC standardization of the samples, the likely presence of bioactive compounds also justifies the procedure for extraction as well as the activity of the plant extract.
Figure 1. HPLC chromatogram of NIME. Inset shows the expanded region of the chromatogram with retention time of 10–13.5 min. Peaks marked signify the retention peak of ellagic acid (10.82 min), methyl gallate (14.14 min), catechin (18.42 min), and reserpine (19.93 min).
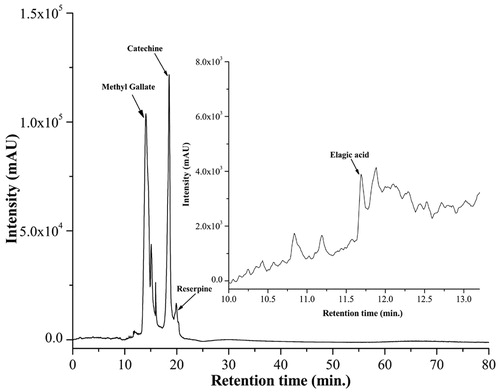
Table 1. Qualitative and quantitative phytochemical analysis of N. indicum leaf extract.
In iron-overloaded liver, iron takes part in the initiation and propagation of various ROS which then leads to the oxidative damage to many vital biomolecules, resulting in the peroxidation of membrane lipids, mitochondrial damage, DNA fragmentation, and ultimately cell death (Sayre et al., Citation2005). The extract when checked for its in vitro DNA protective potential, it showed the ability to protect the scission of pUC-18 plasmid DNA () in a dose-dependent manner. pUC-18 supercoiled DNA was used as a control (lane 1). Lane 2 comprised only of the open circular form of DNA generated by Fenton’s reaction. Addition of gradually increasing concentrations of NIME resulted in the restoration of DNA in the supercoiled form (lanes 3–8). The results in showed the dose-dependent protection by NIME with a [P]50 value of 70.33 ± 0.32 µg. This observation infers the antioxidative, especially hydroxyl radical scavenging as well as iron-chelating ability of the extract. Previous observations suggested that the extracts with the ability to chelate free iron as well as to protect Fenton reaction mediated supercoiled DNA damage in vitro were also exhibited promising in vivo iron chelation and thereby hepato-protective activity (Hazra et al., Citation2012; Sarkar et al., Citation2012a,Citationb). Our current observation of in vitro study depicts the challenging hydroxyl radical scavenging as well as iron-chelating ability of NIME and thereby its possible in vivo hepatoprotective activity against iron-induced toxicity.
Figure 2. DNA protection activity of NIME. (A) In vitro protection against oxidative damage to pUC-18 by NIME. Picture of agarose gel of pUC-18 DNA showing bands of supercoiled (SC) and open circular (OC) forms. Lanes on the gel represent: (lane 1) control DNA (no H2O2 or Fe2+); (lane 2) reaction mixture without extract; (lanes 3–8) reaction mixture with extract of increasing concentrations and (B) the graph represents its % of protection, for NIME. The results are mean ± S.D (n = 6). ***p < 0.001 versus 0 µg/ml.
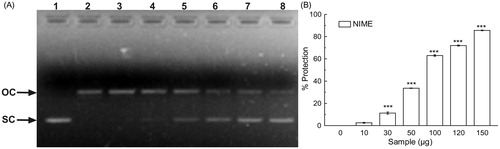
In the present study, iron-overloaded condition in mice was developed by the intraperitoneal administration of iron–dextran. Intraperitoneal iron–dextran injection resembled the hemochromatosis while avoiding direct interruption of plant extract on intestinal iron absorption leading to hepatic and serum iron overload. Iron-overloaded hepatic damage leads to the leakage of the cellular enzymes into the bloodstream resulting in increased levels of serum ALAT, ASAT, ALP, and bilirubin (Reddy & Lokesh, Citation1996). Iron–dextran administration was shown to cause severe acute liver damage in mice, as demonstrated by a significant elevation of ALAT (196%), ASAT (148%), ALP (300%), and bilirubin (116%) levels in serum, as shown in . After treatment with NIME, the levels of these enzymes and bilirubin were significantly restored, and the values obtained were well competent with those treated with standard iron chelator drug desirox, thereby revealing its hepatoprotective activity.
Table 2. The effect of NIME on serum markers (ALAT, ASAT, ALP, and bilirubin) and liver parameters (SOD, catalase, GST, and GSH) in iron-overloaded mice.
Cells possess intrinsic defense mechanism against oxidative stresses which involve the range of antioxidant enzymes such as SOD, CAT, GST, and non-enzymatic antioxidant, GSH (Beckman & Ames, Citation1998). The activity of antioxidant enzymes and the non-enzymatic antioxidant was significantly reduced in iron-overloaded mice. After orally treating the iron-overloaded mice with NIME, the levels of these antioxidant parameters were significantly increased as shown in . It is found that, except for SOD and GSH, in other studies the highest dose of the extract approached the value near that of the Desirox.
TBARS, the end product of the lipid peroxidation, was increased (74%) as the result of the oxidative stress in iron-overloaded mice compared with the normal mice. Elevated level of TBARS is assumed as the main marker of hepatocellular injury (Bonkovsky et al., Citation1981). As shown in , after treatment with NIME, TBARS is substantially decreased to a normal level. Iron-induced pathogenicity in liver also leads to oxidation of protein that results in the modification of catalytic and structural functions of various essential proteins (Dalle-Donne et al., Citation2003). Another consequence of liver damage is the excessive accumulation of extracellular proteins including collagen and significantly increased the amount of hydroxyproline which leads to liver fibrosis (Ramm & Ruddell, Citation2005). As reflected in , an elevation of protein carbonyl (155%) and hydroxyproline (138%) contents was evident in iron-overloaded mice compared with normal. When treated with NIME, these two liver damage markers were also found significantly arrested with increasing doses.
Figure 3. Inhibitory effect of NIME on the biochemical parameters of liver damage against iron-overloaded induced hepatic injury in mice. (A) Lipid peroxidation level, (B) protein carbonyl content and (C) collagen content (hydroxyproline content). Mice were randomly divided into six groups (blank, B; control, C; 50 mg/kg b.w. NIME, S50; 100 mg/kg b.w. NIME, S100; 200 mg/kg b.w. NIME, S200; desirox group, D) and treated as described in ‘Experimental design’ section. Values are expressed as mean ± SD of six mice. **p ≤ 0.01, ***p ≤ 0.001 compared with blank and #p < 0.05, ##p ≤ 0.01, ###p ≤ 0.001 compared with control.
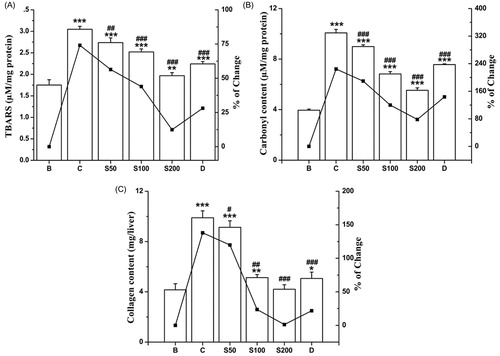
On the basis of the fact that 5/6th part of the surfeited iron in our body is settled in the liver, most procedures seek the measurement of liver iron levels for diagnosis. A raised up liver iron content (132.97%) was found in the iron-overloaded mice compared with normal mice. An increased of NIME reduced the iron levels to 118.15, 82.21, and 49.42% with respect to doses S50, S100, and S200, respectively () in the respective groups. An intracellular protein, ferritin, is responsible for storage of iron in a non-toxic form; modulating the body’s iron level and also helps in preventing iron from facilitating oxidative damage to cell constituents. Serum ferritin is one of the key markers developed as a consequence of iron-overloaded induced hepatic toxicity as the amount of ferritin in a blood indirectly reflects the amount of iron present in the liver. Upon NIME administration, a notable tendency of dose-dependent reduction in serum ferritin concentrations was observed as good as the standard (); thus supporting its iron-chelating potency.
Figure 4. In vivo hepatic and serum iron chelation capacity of NIME on iron-overloaded mice. (A) Liver iron content and (B) serum ferritin level. Mice were randomly divided into six groups (blank, B; control, C; 50 mg/kg b.w. NIME, S50; 100 mg/kg b.w. NIME, S100; 200 mg/kg b.w. NIME, S200; desirox group, D) and treated as described in the ‘Experimental design’ section. Values are expressed as mean ± SD of six mice. **p ≤ 0.01, ***p ≤ 0.001 compared with blank and ##p < 0.01, ###p ≤ 0.001 compared with control.
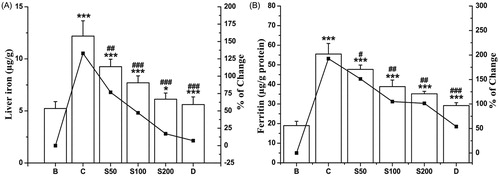
Various iron chelators are available in the market and administered to attenuate the situation created by iron overload. As iron in ferritin is not properly accessed to the applied drug, most of these chelators have limited binding activity for ferric iron. So, iron chelation therapy is dependent on the reductive release of ferritin iron, which is achieved by supplemented addition of a reducing agent such as ascorbate to increase the availability of storage iron to chelators (O’Brien, Citation1974). Previously, the extract of N. indicum leaf had shown reductive ability (Dey et al., Citation2012) and here, the ability of NIME to reductively release the iron from ferritin was quantified by measuring the formation of the ferrous complex of ferrozine [Fe(ferrozine)3]2+. Control experiments without NIME produced negligible amounts of [Fe(ferrozine)3]2+, whereas, after dose-dependant addition of NIME the [Fe(ferrozine)3]2+ complex formation was increased significantly with time ().
Figure 5. Effect of NIME on reductive release of ferritin iron and its correlation with Fe2+-reducing power. (A) Reductive release of ferritin iron. (B) Correlation between released ferritin iron with a reducing power. The iron released in response to the increasing amounts (100–500 μg) of NIME was plotted against reducing power displayed by the same doses.
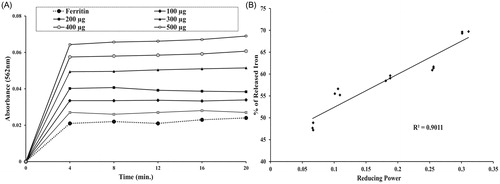
In the present study, a significant positive correlation (R2 = 0.9011) between reducing power and (%) iron released from ferritin has been well established (). Therefore, NIME can also be used as a source of drug to treat iron-overloaded disease state as the present results showed its reductive release activity of ferritin iron dose dependently as well as time dependently.
Histopathological study
Histological observations are executed along with the levels of various biochemical parameters in circulation to mark the extent of hepatic damage. The liver sections of normal mice showed normal cell morphology with well-preserved cytoplasm, prominent nucleus, and well-brought-out central vein (). Iron-dextran control mice showed different degrees of pathological changes including hepatocellular necrosis, ballooning degeneration, inflammation, and loss of cellular boundaries (). In contrast, the liver sections taken from NIME-treated mice groups displayed evidence of lessening of the pathogenesis and revealed a marked reduction in hepatic injuries (). exhibited the improved histology of the liver section taken from the desirox-treated group that is almost similar to the S200 group. However, these observations indicate the in situ hepatoprotective evidence of the extract. The liver sections of the extract-treated mice from histological data showed decrement in diffused ballooning degeneration, restoration from severe hepatic lesions, and neutrophilic cellular inflammation thus gradually impending towards healthy cytoarchitecture with increasing NIME concentration per kg body weight which supports the hepatoprotective activity of the extract.
Figure 6. Photomicrograph of mice liver sections (staining with hematoxylin and eosin) ×40. (A) Control mice liver shows normal cellular integrity. (B) Iron-intoxicated (iron dextran, 100 mg/kg b.w.) liver section showing necrosis (N), fatty-ballooning degeneration, inflammation (I), and loss of cellular boundaries. (C) Liver section treated with iron–dextran + 50 mg/kg b.w. NIME shows improved histology with portal inflammation (PI). (D) Liver section treated with iron–dextran + 100 mg/kg b.w. NIME. (E) Liver section treated with iron–dextran + 200 mg/kg b.w. NIME. (F) Liver section treated with iron–dextran + 20 mg/kg b.w. desirox shows reduced necrotic area and the increased number of hepatocytes. S100 and S200 show reduced hepatocellular necrosis, ballooning degeneration, and inflammation indicating a trend of restoration of normal cellular integrity.
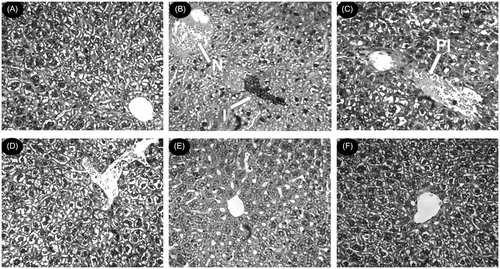
The methanol extract of N. indicum flowers also showed a remarkable hepatoprotective activity against CCl4-induced liver injury in rats at a dose of 400 mg/kg b.w. (Singhal & Gupta, Citation2012). However, the same result was observed at a dose of 200 mg/kg b.w. in case of NIME. When ‘Triphala’ components (Terminalia chebula, Terminalia belerica, and Emblica officinalis) were individually tested in iron-overloaded mice, all the three extracts showed outstanding hepatoprotective activity against iron-induced oxidative stress and liver injury. It was found that the extracts were more efficient in normalizing the levels of serum enzymes and antioxidants as compared with the N. indicum leaf. Liver damage parameters, which are the indicators of the liver health, were also checked and found that the extracts of ‘Triphala’ components were effective in their hepatoprotective activity as compared with the extracts of N. indicum leaf (Hazra et al., Citation2012; Sarkar et al., Citation2012b, Citation2013a). Nevertheless, this activity of NIME was far better as compared with the other Southeast Asian plant, Caesalpinia crista (Sarkar et al., Citation2012a).
Conclusions
The current study concludes the protective effect of NIME against iron-overload-induced liver toxicity as evidenced by biochemical and histopathological studies. NIME may exert its hepatoprotective activity by upregulating antioxidant enzymes and chelating iron to excrete from the body. This activity of the plant extract can be attributed to the significant level of bioactive phytoconstituents. The current findings suggest that NIME can be used as potential iron-chelating drug in the treatment of iron-overloaded diseases.
Acknowledgements
The authors would like to acknowledge Mr. Rhitajit Sarkar and Dr. Bibhabasu Hazra for critical reviewing of the manuscript. The authors would also like to thank Mr. Ranjit Das and Mr. Pradip Mallik for technical assistance in sample preparation, handling of labwares, and animals in experimental procedures.
Declaration of interest
Mr. Dipankar Chaudhuri is grateful to Council of Scientific and Industrial Research (CSIR), Government of India for the support of fellowship. The authors declare that there is no conflict of interest.
References
- Al-Refaie FN, Wonke B, Hoffbrand AV, et al. (1992). Efficacy and possible adverse effects of the oral iron chelator 1,2-dimethyl-3-hydroxypyrid-4-one (L1) in thalassemia major. Blood 80:593–9
- Anusha M, Venkateshwrlu M, Prabhakaran V, et al. (2011). Hepatoprotective activity of aqueous extract of Portulaca oleracea in combination with lycopene in rats. Indian J Pharmacol 43:563–7
- Barry M, Sherlock S. (1971). Measurement of liver iron concentration in needle-biopsy specimens. Lancet 297:100–3
- Beckman KB, Ames BN. (1998). The free radical theory of aging matures. Physiol Rev 78:547–81
- Bergman I, Loxley R. (1963). Two improved and simplified methods for the spectrophotometric determination of hydroxyproline. Anal Chem 35:1961–5
- Bonaventura J, Schroeder WA, Fang S. (1972). Human erythrocyte catalase: An improved method of isolation and a re-evaluation of reported properties. Arch Biochem Biophys 150:606–17
- Bonkovsky HL, Healey JF, Sinclair PR, et al. (1981). Iron and the liver acute and long-term effects of iron-loading on hepatic haem metabolism. Biochem J 196:57–64
- Buege JA, Aust SD. (1978). Microsomal lipid per oxidation. Methods Enzymol 52:302–10
- Chopra RN, Nayar SL, Chopra IC. (1956). Glossary of Indian Medicinal Plants. New Delhi, India: Council of Scientific and Industrial Research
- Cook NC, Samman S. (1996). Flavonoids-chemistry, metabolism, cardioprotective effects, and dietary sources. J Nutr Biochem 7:66–76
- Dalle-Donne I, Rossi R, Giustarini D, et al. (2003). Protein carbonyl groups as biomarkers of oxidative stress. Clin Chim Acta 329:23–38
- Deugnier YM, Loreal O, Turlin B, et al. (1992). Liver pathology in genetic hemochromatosis: A review of 135 homozygous cases and their bioclinical correlations. Gastroenterology 102:2050–9
- Dey P, Chaudhuri D, Chaudhuri TK, Mandal N. (2012). Comparative assessment of the antioxidant activity and free radical scavenging potential of different parts of Nerium indicum. Int J Phytomed 4:54–69
- Ellman GL. (1959). Tissue sulfhydryl group. Arch Biochem Biophys 82:70–7
- Gardi C, Arezzini B, Fortino V, Comporti M. (2002). Effect of free iron on collagen synthesis, cell proliferation and MMP-2 expression in rat hepatic stellate cells. Biochem Pharmacol 64:1139–45
- Habig WH, Pabst MJ, Jakoby WB. (1974). Glutathione S transferases. The first enzymatic step in mercapturic acid formation. J Biol Chem 249:7130–9
- Halliwell B, Gutteridge JMC. (1984). Oxygen toxicity, oxygen radicals, transition metals and disease. Biochem J 219:1–14
- Harborne JB, Baxter H. (1995). Phytochemical Dictionary: A Handbook of Bioactive Compounds from Plants. London: Taylor and Francis Ltd
- Hazra B, Sarkar R, Mandal N. (2012). Protection of Terminalia belerica roxb. against iron overload induced liver toxicity: An account of its reducing and iron chelating capacity. Am J Pharmacol Toxicol 7:109–22
- Hermes-Lima M, Nagy E, Ponka P, Schulman HM. (1998). The iron chelator pyridoxal isonicotinoyl hydrazone (PIH) protects plasmid pUC-18 DNA against OH mediated strand breaks. Free Radical Bio Med 25:875–80
- Hynes MJ, Coinceanainn M. (2002). Investigation of the release of iron from ferritin by naturally occurring antioxidants. J Inorg Biochem 90:18–21
- Kakkar P, Das B, Viswanathan PN. (1984). A modified spectrophotometric assay of superoxide dismutase. Indian J Biochem Biophys 21:130–2
- Kivirikko KI, Laitinen O, Prockop DJ. (1967). Modifications of a specific assay for hydroxyproline in urine. Anal Biochem 19:249–55
- Kokate CK, Purohit AP, Gokhale SB. (1997). Pharmacognosy, 7th ed. Pune, India: Nirali Prakashan
- Kontoghiorghes GJ. (2003). Do we need more ironchelating drugs? Lancet 362:495–6
- Liu ZD, Hider RC. (2002). Design of clinically useful iron (III)-selective chelators. Med Res Rev 22:26–64
- López M, Martínez F, Del Valle C, et al. (2003). Study of phenolic compounds as natural antioxidants by a fluorescence method. Talanta 60:609–16
- Lowry OH, Roesborough MJ, Farr AL, Randall RJ. (1951). Protein measurement with Folin–Phenol reagent. J Biol Chem 193:265–75
- Manjunath BL. (1966). The Wealth of India. New Delhi: Council of Scientific and Industrial Research, 15
- Niederau C, Fischer R, Sonnenberg A, et al. (1985). Survival and causes of death in cirrhotic and in noncirrhotic patients with primary hemochromatosis. N Engl J Med 313:1256–62
- O’Brien RT. (1974). Ascorbic acid enhancement of desferrioxamine induced urinary iron excretion in thalassemia major. Ann NY Acad Sci 232:221–5
- Olynyk J, Hall P, Reed W, et al. (1995). A long-term study of the interaction between iron and alcohol in an animal model of iron overload. J Hepatol 22:671–6
- Papanastasiou DA, Vayenas DV, Vassilopoulos A, Repanti M. (2000). Concentration of iron and distribution of iron and transferrin after experimental iron overload in rat tissues in vivo: Study of the liver, the spleen, the central nervous system and other organs. Pathol Res Pract 196:47–54
- Pathak S, Multani AS, Narayan S, et al. (2000). Anvirzel, an extract of Nerium oleander, induces cell death in human but not murine cancer cells. Anticancer Drugs 11:455–63
- Ramm GA, Ruddell RG. (2005). Hepatotoxicity of iron overload: Mechanisms of iron-induced hepatic fibrogenesis. Semin Liver Dis 25:433–49
- Reddy AC, Lokesh BR. (1996). Effect of curcumin and eugenol on iron-induced hepatic toxicities in rats. Toxicology 107:39–45
- Reznick AZ, Packer L. (1994). Oxidative damage to proteins: Spectrophotometric method for carbonyl assay. Methods Enzymol 233:357–63
- Rice-Evans CA, Miller NJ, Paganga G. (1996). Structure-antioxidant activity relationships of flavonoids and phenolic acids. Free Radic Biol Med 20:933–56
- Robert EB. (1971). Method for estimation of tannin in grain sorghum. Agro J 63:511–2
- Roe JH. (1961). Comparative analysis for ascorbic acid by the 2,4-dinitro-phenylhydrazine method with the coupling reaction at different temperatures: A procedure for determining specificity. J Biol Chem 236:1611–3
- Sadasivam S, Manickam A. (1997). Biochemical Methods. New Delhi: New Age International (P) Limited, 10–197
- Sarkar R, Hazra B, Mandal N. (2012a). Hepatoprotective potential of Caesalpinia crista against iron-overload-induced liver toxicity in mice. Evid Based Complement Altern Med 2012:Article ID 896341
- Sarkar R, Hazra B, Mandal N. (2012b). Reducing power and iron chelating property of Terminalia chebula (Retz.) alleviates iron induced liver toxicity in mice. BMC Complement Altern Med 12:44
- Sarkar R, Hazra B, Mandal N. (2013a). Amelioration of iron overload-induced liver toxicity by a potent antioxidant and iron chelator, Emblica officinalis Gaertn. Toxcol Ind Health. [Epub ahead of print]. doi:10.1177/0748233713483195
- Sarkar R, Hazra B, Mandal N. (2013b). Anti-oxidative protection against iron-induced liver damage in mice by Cajanus cajan (L.) Millsp. leaf extract. Indian J Exp Biol 51:165–73
- Sayre LM, Moreira PI, Smith MA, Perry G. (2005). Metal ions and oxidative protein modification in neurological disease. Ann Ist Super Sanita 41:143–64
- Siddiqui BS, Sultana R, Begum S, et al. (1997). Cardenolides from the methanolic extract of Nerium oleander leaves possessing central nervous system depressant activity in mice. J Nat Prod 60:540–4
- Siddiqui S, Begum S, Siddiqui BS, Lichter W. (1995). Two novel cytotoxic pentacyclic triterpenoids from Nerium oleander leaves. Phytochemistry 39:171–4
- Singh DK, Srivastava B, Sahu A. (2004). Spectroscopic determination of rauwolfia alkaloids: Estimation of reserpine in pharmaceuticals. Anal Sci 20:571–3
- Singhal KG, Gupta GD. (2012). Hepatoprotective and antioxidant activity of methanolic extract of flowers of Nerium oleander against CCl4-induced liver injury in rats. Asian Pac J Trop Med 9:677–85
- Tam TF, Leung-Toung R, Li W, et al. (2003). Iron chelator research: Past, present and future. Curr Med Chem 10:983–95
- Wolf PL. (1999). Biochemical diagnosis of liver disease. Indian J Clin Biochem 14:59–90
- Zia A, Siddiqui BS, Begum S, Suria A. (1995). Studies on the constituents of leaves of Nerium oleander on behavior pattern in mice. J Ethnopharmacol 49:33–9