Abstract
Context: Albizia procera (Roxb.) Benth. (Mimosaceae) has been traditionally used in Thai longevity preparations. Thus, searching for HIV-1 integrase (HIV-1 IN) agents from natural sources is of interest.
Objective: The objective of this study is to examine the inhibitory activity against HIV-1 IN of compounds isolated from the stem bark of Albizia procera.
Materials and methods: The EtOH extract and isolated compounds of Albizia procera bark were examined for anti-HIV-1 IN activity at various concentrations (10–100 µg/mL and 10–100 µM) using the multiplate integration assay and molecular docking.
Results and discussions: The results showed that the ethanol extract had good anti-HIV-1 IN activity with an IC50 value of 19.5 µg/mL, whereas ethyl acetate fraction exhibited the most potent with an IC50 value of 19.1 µg/mL, followed by water fraction (IC50 value = 21.3 µg/mL), hexane and chloroform fractions (IC50 value > 100 µg/mL), respectively. From bioassay-guided isolation, the ethyl acetate fraction was further separated to give two compounds which are (+)-catechin (1) and protocatechuic acid (2), respectively. Of the tested samples, (+)-catechin (1) exhibited appreciable activity against HIV-1 IN with an IC50 value of 46.3 µM, whereas protocatechuic acid (2) showed mild activity with 46.0% inhibition at concentration of 100 µM. (+)-Catechin (1) could interact with Thr66, Gly148, and Glu152 in the core domain of IN enzyme, whereas protocatechuic acid (2) could bind with Thr66, His67, Glu152, Asn155, and Lys159. This is the first report on anti-HIV-1 IN activity of Albizia procera bark. These results may suggest that Albizia procera bark has potential as anti-HIV-1 IN agent.
Introduction
Highly active antiretroviral therapy (HAART) is now required for the treatment of an acquired immunodeficiency syndrome (AIDS). This therapy is based on the combinations of HIV-1 protease (PR) and HIV-1 reverse transcriptase (RT) inhibitors. AIDS has been rapidly spreading in many countries and is worldwide a public health problem. Three enzymes that are essential for the HIV-1 life cycle are HIV-1 PR, RT and integrase (IN) (Katz & Skalka, Citation1994; Lucia, Citation2007). HIV-1 IN has become an appealing target for AIDS treatment since only three HIV-1 IN inhibitors (raltegravir, elvitegravir, and dolutegravir) are now available in the market. Moreover, Albizia procera has not been studied on anti-HIV-1 IN effect. Thus, searching for HIV-1 IN inhibitors from natural sources is becoming an interesting target for AIDS treatment.
HIV-1 IN enzyme is a 32 kDa protein produced from the C-terminal portion of the pol-gene product, which minimally functions as a dimer with 288 amino acids each. The catalytic core domain contains the catalytic triad of acidic residues, the D, D-35 E motif, which are Asp64, Asp116, and Glu152 in the case of HIV-1 IN (Craigie, Citation2001).
Albizia procera (Roxb.) Benth. (Mimosaceae), tall albizia, is a medicinal plant used in traditional medicine prepared for longevity, locally known in Thai as Thing thon. The bark and leaves of Albizia procera are extensively used for the treatment of a variety of wounds and are considered useful in pregnancy and stomachache (Kokila et al., Citation2013). Since the EtOH extract of this plant showed a good effect against HIV-1 IN with an IC50 value of 19.5 µg/mL, its fractions and isolated compounds were therefore tested on anti-HIV-1 IN activity and studied on molecular docking.
Materials and methods
Plant material
The Albizia procera bark was collected from the Suan Ya Thai Thongnoppakhun herbal garden in Angthong province in 2011 and was identified by a Thai traditional doctor, Mr. Sraupsin Thongnoppakhun, and the voucher specimen number is SKP 115011601. The sample was kept at the Herbarium of Department of Pharmacognosy and Pharmaceutical Botany, Faculty of Pharmaceutical Sciences, Prince of Songkla University, Thailand.
Isolation of compounds from Albizia procera bark extract
Three kilograms dried weight of Albizia procera was cleaned, cut into small pieces, and ground to powder. The powder (3 kg) was macerated five times with ethanol (5 × 8 L) at room temperature. The solvent was removed under reduced pressure to give 202.2 g of crude EtOH extract and then partitioned between 90% methanol (500 mL) and hexane (500 mL), then methanol was removed, water (500 mL) was added, and partitioned with chloroform (500 mL). After that the water layer was partitioned with ethyl acetate (500 mL). Each partition was evaporated to dryness in vacuo to give residues of hexane (7.0 g), chloroform (0.6 g), ethyl acetate (14.4 g), and water fractions (98.4 g).
The fractions were dried under reduced pressure and then re-dissolved in 50% DMSO for bioassay. These fractions were prepared in the concentration of 3–100 µg/mL. The ethyl acetate fraction (14.4 g), which showed good separation on thin-layer chromatography (TLC) and exhibited marked anti-HIV-1 IN activity (IC50 value = 19.1 µg/mL), was further separated by silica gel column chromatography using EtOAc:MeOH:H2O (98:1:1–96:2:2) to afford 113 fractions (F1–F113). Fractions F4–F5 (0.74 g) were purified on silica gel (20 g) using CHCl3:MeOH (8:2) to 100% MeOH to give 33 subfractions (F1a–F33a). Fractions F6a–F8a (0.32 g) were purified by column chromatography on Sephadex LH-20 (St. Louis, MO) using 100% MeOH to obtain F1b–F9b. Fraction F3b was chromatographed on Sephadex LH-20 using 100% MeOH to afford (+)-catechin (1) (white solid, 13.3 mg, 0.09% w/w). Fractions F6–F13 (0.21 g) were purified by column chromatography on silica gel using CHCl3:MeOH (9:1) to give F1d–F7d. Fractions F6d–F7d (15 mg) were chromatographed on Sephadex LH-20 using 100% MeOH to give protocatechuic acid (2) (white solid, 10.8 mg, 0.07% w/w).
The structures of compounds 1 and 2 were elucidated using spectroscopic techniques and compared with reported spectral data (Davis et al., Citation1996).
Identifications of compounds 1 and 2
(+)-Catechin (1): white solid, UV (MeOH) λmax: 289, 375 nm. 1H-NMR (CD3OD, 500 MHz): δ 2.49 (1H, dd, J = 16.5, 8.0 Hz, H-4b), 2.80 (1H, dd, J = 16.5, 5.5 Hz, H-4a), 3.96 (1H, m, H-3), 4.55 (1H, d, J = 8.0 Hz, H-2), 5.84 (1H, d, J = 2.0 Hz, H-8), 5.92 (1H, d, J = 2.0 Hz, H-6), 6.71 (1H, d, J = 8.0 Hz, H-5′), 6.75 (1H, dd, J = 8.0, 2.0 Hz, H-6′), 6.83 (1H, d, J = 2.0 Hz, H-2′). 13C-NMR (CD3OD, 125 MHz): δ 28.5 (C-4), 68.2 (C-3), 82.9 (C-2), 95.5 (C-8), 96.3 (C-6), 100.8 (C-10), 115.2 (C-2′), 116.1 (C-6′), 120.0 (C-5′), 132.2 (C-1′), 146.2 (C-3′), 146.2 (C-4′), 156.9 (C-9), 157.6 (C-5), 157.8 (C-7).
Protocatechuic acid (2): white solid, UV (MeOH) λmax: 299 nm. 1H-NMR (CD3OD, 500 MHz): δ 6.78 (1H, d, J = 8.0 Hz, H-5), 7.42 (1H, dd, J = 8.0, 2.0 Hz, H-6), 7.43 (1H, d, J = 2.0 Hz, H-2). 13C-NMR (CD3OD, 125 MHz): δ 115.8 (C-5), 117.9 (C-2), 123.1 (C-1), 123.9 (C-6), 146.1 (C-3), 151.4 (C-4), 170.9 (CO).
Anti-HIV-1 IN assay
The anti-HIV-1 IN activity was evaluated according to the method previously reported (Tewtrakul et al., Citation2001). Briefly, a mixture (45 µL), composed of 12 µL of IN buffer [containing 150 mM 3-(N-morpholino) propane sulfonic acid, pH 7.2 (MOPS), 75 mM MnCl2, 5 mM dithiothritol (DTT), 25% glycerol and 500 µg/mL bovine serum albumin], 1 µL of 5 pmol/mL digoxigenin-labelled target DNA and 32 µL of sterilzed water, was added into each well of a 96-well plate. Subsequently, 6 µL of sample solution and 9 µL of 1/5 dilution of integrase enzyme were added to each well and incubated at 37 °C for 80 min. The wells were then washed with PBS four times, and 100 µL of 500 mU/mL alkaline phosphatase (AP)-labelled anti-digoxigenin antibody was then added to all wells and incubated at 37 °C for 1 h. The plate was washed again with washing buffer containing 0.05% Tween 20 in PBS four times and with PBS four times. Then, AP buffer (150 µL) containing 100 mM Tris-HCl (pH 9.5), 100 mM NaCl, 5 mM MgCl2, and 10 mM p-nitrophenyl phosphate was added to each well and incubated at 37 °C for 1 h. Finally, the plate was measured with a microplate reader at a wavelength of 405 nm. The control consisted of a reaction mixture, 50% DMSO and an integrase enzyme, while the blank was buffer-E containing 20 mM MOPS (pH 7.2), 400 mM potassium glutamate, 1 mM ethylenediaminetetraacetate disodium salt (EDTA. 2Na), 0.1% Non-idet-P 40 (NP-40), 20% glycerol, 1 mM DTT, and 4 M urea without the integrase enzyme. Suramin, a polyanionic HIV-1 IN inhibitor, was used as a positive control. The % inhibition against HIV-1 IN was calculated as follows:
where OD = absorbance detected from each well.
Molecular docking method
Ligand preparation
The three-dimensional structure of pure compounds was generated using Hyperchem professional 8.0 (Gainesville, FL). Energy minimization of each compound was performed using the PM3 semi-empirical method. Subsequently, geometry optimization was done for each compound using energy 0.05 kcal/mol conjugated gradients algorithm. Before docking, Gasteiger charges were assigned to each compound.
HIV-1 IN preparation
The crystal structure of the core domain of HIV-1 IN, residues CYS56–GLN209, complexed with inhibitor 1-(5-chloroindol-3-yl)-3-hydroxy-3-(2H-tetrazol-5-yl)-propenone (5CITEP) in the active site was obtained from Protein Data Bank (PDB code 1QS4). Only Chain A that co-crystallized with 5-CITEP was selected, while chains B and C were deleted. All the water molecules and 5CITEP were removed, while a magnesium ion at the active site was maintained. The missing residues in the loop at positions 141–144 were incorporated and polar hydrogen atoms were added to this chain (Vajragupta et al., Citation2005). The second magnesium ion was placed in the same relative position according to the two metal structures of the Prototype Foamy Virus integrase (PDB code 3OYA), a high structural homolog to HIV-1 IN (Krishnan & Engelman, Citation2012).
Molecular docking
Molecular docking was performed with version 4.2. of the AutoDock program (La Jolla, CA). Before docking, the grid boxes were prepared for IN structures using AutoGrid 4.0 (La Jolla, CA). The center of the grid boxes was set on the location of the co-crystallized inhibitor. The grid dimensions were set to 60 × 60 × 60 Å in each dimension, with a grid spacing of 0.375 Å which is large enough for the free rotation of the ligand.
The docking calculations were carried out using the Lamarckian genetic algorithm (LGA). The optimized docking parameters were set as follows: the number of GA runs was 100, the population size was 150, the maximum number of energy evaluations was increased to 2 500 000 per run and the maximum number of generations in the genetic algorithm was increased to 100 000. All other docking parameters were set at their default.
A cluster analysis was used to group similar conformation, all 100 independent conformations for each compound with the root mean square deviation (RMSD) differing less than 2 Å were clustered together. The best docked conformations were the lowest binding energy and the greatest number of conformations in the cluster (Healy et al., Citation2009). Amino acid residue within 6 Å of the ligand in the IN active site was chosen for H-bond interaction analysis using the HBond monitor in the DS Viewer Pro program (Roseau Valley, Dominica).
Statistical analysis
The values are expressed as mean ± S.E.M of four determinations. The IC50 values were calculated using the Microsoft Excel program (Redmond, WA).
Results and discussion
Since the EtOH extract of Albizia procera possessed potent anti-HIV-1 IN effect (IC50 value = 19.5 µg/mL), the hexane, chloroform, ethyl acetate, and water fractions of Albizia procera bark were tested for anti-HIV-1 IN activity. Among the tested samples, the ethyl acetate fraction exhibited the most potent inhibitory activity with an IC50 value of 19.1 µg/mL, followed by water fraction (IC50 value = 21.3 µg/mL), hexane, and chloroform fractions (IC50 > 100 µg/mL) (). From bioassay-guided fractionation, (+)-catechin (1) and protocatechuic acid (2) were isolated from the ethyl acetate fraction of Albizia procera (). Of the tested samples, (+)-catechin (1) exhibited the potent activity against HIV-1 IN with an IC50 value of 46.3 µM, whereas protocatechuic acid (2) showed mild activity (IC50 value > 100 µM) with 46.0% inhibition at a concentration of 100 µM (). This is the first report on the anti-HIV-1 IN activity of Albizia procera bark. These results may suggest that Albizia procera bark has potential for treating AIDS patients.
Table 1. IC50 valuesa of the extract and fractions of Albizia procera against HIV-1 IN activity.
Table 2. % Inhibitiona and IC50 values of isolated compounds from ethyl acetate fraction of Albizia procera against HIV-1 IN activity.
From molecular docking, it was found that (+)-catechin (1) interacts with Thr66, Gly148, and Glu152 in the core domain of IN enzyme ( and ), whereas protocatechuic acid (2) could bind with Thr66, His67, Glu152, Asn155, and Lys159 ( and ). The result showed that these two compounds bind with the Glu152, which is one of the amino acid residues in the catalytic triad of HIV-1 IN core domain. However, (+)-catechin (1) showed lower binding energy (−5.16 kcal/mol) than that of protocatechuic acid (2, −4.85 kcal/mol), thereby (+)-catechin (1) could have good interaction with HIV-1 IN and possessed higher activity than protocatechuic acid (2).
Figure 2. Molecular docking of (+)-catechin (1) with HIV-1 IN. The ribbon model shows the backbone of HIV-1 IN catalytic domain with all interacting amino acid residues shown as stick models and colored by element. (+)-Catechin is shown pink stick model. H-bond interactions are shown as blue dash lines and represent bond length in angstrom (Å). Mg2+ ions are shown as red balls.
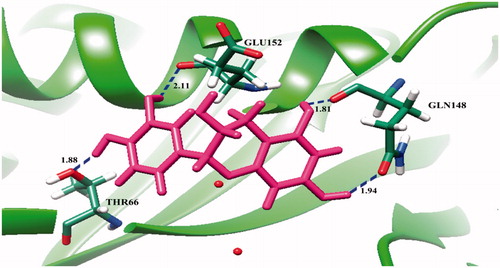
Figure 3. Molecular docking of protocatechuic acid (2) with HIV-1 IN. The ribbon model shows the backbone of HIV-1 IN catalytic domain with all interacting amino acid residues shown as stick models and colored by element. Protocatechuic acid is shown as yellow stick model. H-bond interactions are shown as blue dash lines and represent the bond length in angstrom (Å). Mg2+ ions are shown as red balls.
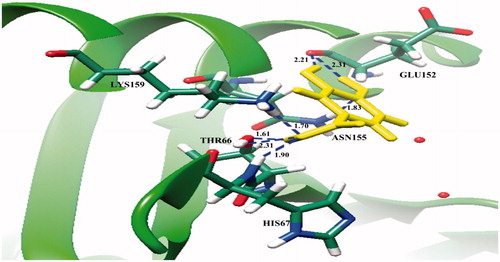
Table 3. Anti-HIV 1 IN activity and molecular docking study of pure compounds from Albizia procera bark.
Regarding the compounds isolated from Albizia procera bark, triterpenoid saponins with N-acetyl sugar were reported to exhibit cytotoxic effect against HEPG2 cell line (Melek et al., Citation2007). Echinocystic acid bisglycosides, the triterepenoid glycoside derivatives, isolated from this plant bark also possessed anticancer activity against HEPG2, HT-29, and MCF-7 cells (Miyase et al., Citation2010). (+)-Catechin derived from tea has been reported to exhibit antibacterial effect against Helicobactor pylori (Mabe et al., Citation1999). Catechins isolated from green tea also had antiviral activity on influenza virus (Song et al., Citation2005) and epigallocatechin gallate was found to inhibit HIV-1 replication by blocking gp120–CD4 interaction (Nance et al., Citation2009). It has been reported that the mixture of gallocatechin and epigallocatechin isolated from Mimusops elengi leaf extract possess good anti-HIV-1 IN effect (Suedee et al., Citation2014). Protocatechuic acid has been shown to have anti-inflammatory and analgesic properties in rats and mice (Lende et al., Citation2011). This compound also inhibited cancer cell metastasis involving down regulation of NF-κB pathway (Lin et al., Citation2011).
Conclusions
Two compounds which are (+)-catechin (1) and protocatechuic acid (2) were isolated from the bark of Albizia procera. (+)-Catechin (1) exhibited marked activity against HIV-1 IN with an IC50 value of 46.3 µM, whereas protocatechuic acid (2) possessed mild effect (IC50 value >100 µM). This is the first report on anti-HIV-1 IN activity of Albizia procera bark. These results suggest that Albizia procera bark has potential as anti-HIV-1 IN agent which may support the use of this plant in treating HIV infection.
Acknowledgements
We thank Dr. Robert Craigie, National Institute of Health (NIH), Bethesda, Maryland, USA, for providing an HIV-1 IN enzyme, the Faculty of Pharmaceutical Sciences, Prince of Songkla University, for providing laboratory facilities, and Mr Sraupsin Thongnoppakhun for plant material support.
Declaration of interest
The authors report that they have no conflicts of interest. The authors are grateful to the Center of Excellence for Innovation in Chemistry (PERCH-CIC) and the Thailand Research Fund (RSA5680012) for the financial support.
References
- Craigie R. (2001). HIV-1 integrase, a brief overview from chemistry to therapeutics. J Biol Chem 276:23213–16
- Davis AL, Cai Y, Davies AP, Lewis JR. (1996). 1H and 13C NMR assignments of some green tea polyphenols. Magn Reson Chem 34:887–90
- Healy EF, Sanders J, Peter J, et al. (2009). A docking study of L-chicoric acid with HIV-1 integrase. J Mol Graphics Model 27:584–9
- Katz RA, Skalka AM. (1994). The retroviral enzymes. Annu Rev Biochem 63:133–73
- Kokila K, Priyadharshini SD, Sujatha V. (2013). Phytopharmacological properties of Albizia species: A review. Int J Pharm Pharm Sci 5:70–3
- Krishnan L, Engelman A. (2012). Retroviral integrase proteins and HIV-1 DNA integration. J Biol Chem 287:40858–66
- Lende AB, Kshirsagar AD, Deshpande AD, et al. (2011). Anti-inflammatory and analgesic activity of protocatechuic acid in rats and mice. Immunopharmacology 19:255–63
- Lin HH, Chen JH, Chou FP, Wang CJ. (2011). Protocatechuic acid inhibits cancer cell metastasis involving the down regulation of Ras/Akt/NF-κB pathway and MMP-2 production by targeting RhoB activation. Br J Pharmacol 162:237–54
- Lucia P. (2007). Role of integrase inhibitors in the treatment of HIV disease. Expert Rev Anti-Infect Ther 5:67–75
- Mabe K, Yamada M, Oguni I, Takahashi T. (1999). In vitro and in vivo activities of tea catechins against Helicobactor pylori. Antimicrob Agents Chemother 43:1788–91
- Melek FR, Miyase T, Ghaly NS, Nabil M. (2007). Triterpenoid saponins with N-acetyl sugar from the bark of Albizia procera. Phytochemistry 68:1261–6
- Miyase T, Melek FR, Ghaly NS, et al. (2010). Echinocystic acid 3, 16-o-bisglycosides from Albizia procera. Phytochemistry 71:1375–80
- Nance CL, Siwak EB, Shearer WT. (2009). Preclinical development of the green tea catechin, epigallocatechin gallate, as an HIV-1 therapy. J Allergy Clin Immunol 123:459–65
- Song JM, Lee KH, Seong BL. (2005). Antiviral effect of catechins in green tea on influenza virus. Antiviral Res 68:66–74
- Suedee A, Tewtrakul S, Panichayupakaranant P. (2014). Anti-HIV-1 integrase activity of Mimusops elengi leaf extracts. Pharm Biol 52:58–61
- Tewtrakul S, Miyashiro H, Hattori M, et al. (2001). Inhibitory effects of flavonoids on human immunodeficiency virus type-1 integrase. J Tradit Med 18:229–38
- Vajragupta O, Boonchoong P, Morrisc GM, Olsonc AJ. (2005). Active site binding modes of curcumin in HIV-1 protease and integrase. Bioorg Med Chem Lett 15:3364–8