Abstract
Context: Although humans are exposed to o-coumaric acid (OCA) in their diet, there is no available literature related to drug interaction and the carcinogen-activating potential of OCA in the HepG2 cell line.
Objective: This study was undertaken to determine the effects of OCA on the cytochrome P450 (CYP) 1A2, CYP2E1, CYP2C9, and CYP3A4 enzymes, which are primarily involved in carcinogen and drug metabolism.
Materials and methods: The cytotoxicity of OCA in HepG2 cells was investigated by measuring the cleavage of WST-1. The protein and mRNA levels of CYPs were determined by western blotting and RT-PCR, respectively.
Results: The EC10, EC25, and EC50 values of OCA were calculated to be 1.84, 3.91 and 7.39 mM, respectively. A sublethal dose of 5 mM was used throughout this study. The CYP1A2 protein and mRNA levels were increased by 52 and 40% (p < 0.05), as were the CYP2E1 levels by 225 and 424%, respectively (p < 0.05). However, OCA treatment caused 52 and 60% decreases in the levels of CYP3A4 protein and mRNA (p < 0.05), respectively. In contrast to CYP3A4, the CYP2C9 protein and mRNA levels increased by 110 and 130%, respectively.
Discussion and conclusion: Co-administration of OCA with some drugs may lead to undesirable food–drug interactions due to modulatory effects on CYP isozymes involved in drug metabolism. Moreover, exposure to OCA may cause an increase in carcinogenicity and toxicity due to the induction of the CYP isozymes involved in chemical carcinogenesis. Therefore, serious precautions should be taken when using OCA as a supplement.
Introduction
In recent years, the use of herbal preparations and phytochemicals isolated from herbs as complementary and alternative therapies has grown considerably in the Western world. Many herbs and herbal phytochemicals are natural and are thus considered to be safe. In addition, their usage has increased in diseases such as cancer, for which conventional methods have often failed to provide satisfactory solutions. However, there are problems regarding the use of such preparations, mainly the lack of critical information regarding the toxicity, allergenicity, mutagenicity, and possible drug interactions of the compounds.
A number of herbs and phytochemicals, including Ginkgo biloba L. (Ginkgoaceae) (Rowin & Lewis, Citation1996), Allium sativum L. (Amaryllidaceae) (Piscitelli et al., Citation2002), Hypericum perforatum L. (Hypericaceae) (Markowitz et al., Citation2000), Urtica urens L. (Urticaceae) (Agus et al., Citation2009; Ozkarsli et al., Citation2008), resveratrol (Detampel et al., Citation2012), curcumin (Cho et al., Citation2012), and silibinin (Li et al., Citation2010), have been reported to interact pharmacokinetically with drugs by altering both the expression and activities of drug-metabolizing enzymes, particularly cytochrome P450s (CYPs). Thus, in vitro and in vivo studies clarifying pharmacokinetic interactions, altered drug concentrations, and enhanced bioactivation of drugs to reactive intermediates, which may be attributable to the induction and/or inhibition of CYPs by co-administered herbs, have important clinical significance (Zhou et al., Citation2003).
The cytochrome P450 superfamily comprises hemoproteins that play key roles in the bioactivation and detoxification of a wide range of xenobiotic substances. Moreover, these enzymes also play important roles in the metabolism and synthesis of endogenous compounds. Multiple CYP isozymes show different substrate specificities and affinities toward both endogenous and exogenous compounds. Indeed, the CYP1A, CYP2E, CYP2C, and CYP3A subfamilies have received a great deal of attention in recent years because of their capacity to metabolize various pharmaceutical agents and their role in carcinogenicity (Arinç et al., Citation2005; Chung et al., Citation2004; Sen & Arinc, Citation1998; Sen et al., Citation2012). Among CYPs, the CYP1A and CYP2E enzymes are primarily involved in carcinogen metabolism, whereas the CYP3A, CYP2D, and CYP2C enzymes typically participate in drug metabolism. Therefore, it is not surprising to observe drug toxicity or carcinogen activation with herbal extracts and herb-based compounds used as complementary therapeutics, as they can be moderate-to-potent inhibitors or inducers of these CYPs.
Recently, more than 5000 different phytochemicals have been identified in fruits, vegetables, and other plants parts, but a large percentage remain unknown (Huang et al., Citation2010). One of the major families of phytochemicals in plants are phenolic compounds, representing a diverse group of compounds, including phenolic acids, flavonoids, tannins, stilbenes, curcuminoids, coumarins, lignans, and quinones (Dai & Mumper, Citation2010; Landete, Citation2012). Among these compounds, phenolic acids have the simplest structure; they are non-flavonoid polyphenolic compounds that are widely present in many fruits, vegetables, cereals and medicinal herbs (De et al., Citation2011; Plazonic et al., Citation2009; Robbins, Citation2003). Therefore, the dietary intake of these phenolic acids is quite high, ranging from 25 mg to 1 g/d depending on the diet (Clifford, Citation1999; Radtke et al., Citation1998; Scalbert & Williamson, Citation2000).
Phenolic acids can be divided into two main groups based on their C1–C6 and C3–C6 backbones: hydroxybenzoic acid (e.g., gallic, vanillic, and syringic acids) and hydroxycinnamic acid (ferulic, caffeic, and p-coumaric acid) (Robbins, Citation2003). o-Coumaric acid is a hydroxycinnamic acid found in many food and plant products, such as coffee, tea, nuts, Mikania laevigata Sch.Bip. ex Baker (Compositae), Mikania glomerata Spreng, Medicago sativa L. (Leguminosae), Caucalis platycarpos L. (Apiaceae), and Urtica urens (Abu Bakar et al., Citation2012; Chon & Kim, Citation2002; Dos Santos et al., Citation2006; Perrone et al., Citation2008; Plazonic et al., Citation2009). Studies have shown that o-coumaric acid (OCA) () has different biological activities, such as antilipidemic, antioxidant, and anticarcinogenic activities (Hsu et al., Citation2009; Yeh et al., Citation2005). Moreover, the effect of OCA treatment on the mRNA levels of CYP isozymes in a human breast cancer cell line (MCF7) was examined recently in our laboratory (Sen et al., Citation2013). Regardless, the liver is the main organ for the metabolism of xenobiotics; drug interactions and the carcinogen-activating potential of OCA for this organ are not known. Therefore, in this study, the effect of OCA on drug-metabolizing CYP enzymes at the mRNA and protein expression levels was investigated in a human hepatocarcinoma cell line (HepG2 cells).
Materials and methods
Chemicals
The following chemicals and reagents were purchased from Sigma-Aldrich Chemical Company (St Louis, MO): o-coumaric acid (OCA), Dulbecco's Modified Eagle Medium (DMEM), fetal bovine serum (FBS), trypsin, penicillin/streptomycin mixture, aniline, bovine serum albumin (BSA), bicinchoninic acid (BCA), glycine, N-2-hydroxyethylpiperazine-N′-2, ethanesulfonic acid (HEPES), phenylmethylsulfonyl fluoride (PMSF), ɛ-aminocaproic acid (ɛ-ACA), sodium dodecyl sulfate (SDS), and 2-amino-2-(hydroxymethyl)-1,3-propanediol (TRIS). WST-1 cell proliferation and cytotoxicity assay kits were purchased from Boster Biological Technology (Pleasanton, CA).
A polyclonal anti-human CYP2E1 antibody was obtained from Oxford Biomedical Research (Rochester Hills, MI), and polyclonal anti-human CYP1A2 and CYP3A4 antibodies were purchased from Santa Cruz Biotechnology (Santa Cruz, CA). Anti-human CYP2C9 was obtained from Abcam PLC (Cambridge, UK). All other chemicals and solvents were obtained from commercial sources at the highest grade of purity available.
Cell culture
A human hepatocellular liver carcinoma cell line (HepG2) was obtained from the European Collection of Cell Cultures (ECACC, UK). The cells were cultured in DMEM supplemented with 10% FBS and a 1% penicillin/streptomycin mixture in a humidified atmosphere of 95% air with 5% CO2 at 37 °C and were subcultured twice a week.
Cytotoxicity assay
HepG2 cells were grown in 96-well plates at a density of 1 × 103 cells/ml culture medium. After a 24 h pre-exposure incubation, the cells were treated with a range of concentrations of OCA between 1 and 10 mM. OCA was dissolved in dimethyl sulfoxide (DMSO), and the final concentration of DMSO did not exceed 0.1%. Control cultures were also treated with 0.1% DMSO. An equal volume of medium without OCA was added to untreated cells (control). The OCA-treated and control cells were incubated for 48 h at 37 °C in a humidified 5% CO2 atmosphere. After 48 h, cell proliferation was assessed using 10% WST-l (4-(3-(4-iodophenyl)-2-(4-nitrophenyl)-2H-5-tetrazolio)-1,3-benzene disulfonate). Briefly, 10 µl WST-l was added to the wells and incubated for 1 h, and then, cell proliferation was assessed by measuring the absorbance at 450 nm. Three replicated wells were used for each experimental condition. Viability was expressed as a percentage of the control.
Cell homogenate preparation
HepG2 cells (1 × 107 cells per 75 cm2 flask) were exposed to 5 mM OCA and harvested after a 24 h treatment. The cells were then washed twice with PBS, scraped from culture dishes into lysis buffer (0.1 M phosphate buffer Tris-HCl, pH 7.8, 0.2% Triton X-100, 2 mM EDTA, 0.5 mM PMFS, 0.3 mM ɛ-ACA, and 1 mM 1,4-dithiothreitol (DTT)) and homogenized mechanically by sonication. The total cellular protein concentration was determined using the BCA assay with BSA as the standard.
Gel electrophoresis and western blotting
SDS-PAGE and western blotting were performed as previously described (Sen & Arinc, Citation1998). HepG2 total protein was extracted with the TRIzol reagent (Invitrogen, Waltham, MA) as described (Lee et al., Citation2001). Protein samples (150 μg) were separated on 8.5 or 12% polyacrylamide gels using a discontinuous buffer system (Laemmli, Citation1970) and transferred to nitrocellulose membranes using the iBlot (Invitrogen, Waltham, MA) dry blotting system (20 V, 12 min) by employing iBlot gel-transfer stacks. Following transfer, the membranes were blocked with 5% non-fat dry milk in TBS-T [20 mM Tris-HCl, pH 7.4, 400 mM NaCl, and 0.1% (v/v) Tween-20] for 60 min and incubated with polyclonal anti-human CYP2E1, CYP1A2, CYP2C9, or CYP3A4 antibody (diluted 1:200–1:500 in blocking solution) overnight at room temperature. The membranes were then washed with TBS-T (3 × 5 min), incubated with the secondary antibody (HRP-conjugated secondary antibody at a 1:5000 or 1:10 000 dilution) for 60 min and again washed with TBST (3 × 5 min). The proteins were detected using SuperSignal® West Pico chemiluminescent substrate (Pierce, Rockford, IL). The final images were photographed using a computer-based gel imaging DNR LightBIS Pro Image Analysis System (Invitrogen, San Diego, CA), and the protein bands were quantified using Scion Image Version Beta 4.0.2 software (National Institutes of Health, Bethesda, MD).
RNA isolation and RT-PCR of CYP mRNAs
Total RNA was extracted from HepG2 cells using the TRIzol reagent. The extracted RNA was quantified spectrophotometrically at 260/280 nm, and the integrity was checked using a 1% agarose gel. For cDNA synthesis, 2.5 µg of RNA was incubated at 70 °C for 10 min with 0.5 µg of oligo (dT). After 5 min on ice, 50 U of Moloney murine leukemia virus reverse transcriptase, 1 mM dNTPs, and 5X reaction buffer were added to the previous mixture and incubated at 42 °C for 60 min. The reaction was stopped by heating at 70 °C for 10 min, and the cDNA was stored at −80 °C for further use.
A semi-quantitative two-step RT-PCR assay was performed using gene-specific primers. The oligonucleotide sequences used as forward and reverse primers for human CYP isozymes were based on those reported in the literature (Maruyama et al., Citation2007; Wilkening et al., Citation2003), and the 18S rRNA gene was used as a control housekeeping gene. Preliminary control experiments (data not shown) were carried out to verify the RT-PCR conditions that allowed for linear amplification. The PCR products were analyzed by electrophoresis on 1.5–2% agarose gels containing ethidium bromide, and the intensity of the bands was measured using Scion Image Version Beta 4.0.2 software (National Institutes of Health, Bethesda, MD). The mRNA levels of the CYP genes were determined by measuring the intensity of the RT-PCR product bands on each agarose gel and are reported relative to 18S rRNA expression ().
Table 1. Oligonucleotide primers sequences and amplification conditions.
Statistical analysis
Statistical analyses were performed using the Minitab statistical software package (Minitab Inc., State College, PA). All results are expressed as the means including the standard error of means (SEM). A comparison between groups was performed using Student’s t test, and p < 0.05 was selected as the level required for statistical significance.
Results
The cytotoxicity of OCA in HepG2 cells was investigated by measuring the cleavage of WST-1 to formazan by metabolically active cells. As shown in , OCA treatment showed a dose-dependent cytotoxic effect on HepG2 cells. The viability of the treated HepG2 cells was reduced by 11.1 and 31.3% after incubation with 2.5 and 5 mM OCA, respectively, compared with the untreated control cells. The EC10, EC25, and EC50 values of OCA were found to be 1.84, 3.91, and 7.39 mM, respectively. A sublethal dose of 5 mM was used throughout this study.
Figure 2. Cytotoxicity of o-coumaric acid (OCA) on HepG2 cells after 48 h. The results are expressed as the means of two independent experiments, with each experiment performed in triplicate. *Significantly different from the respective control value (p < 0.05).
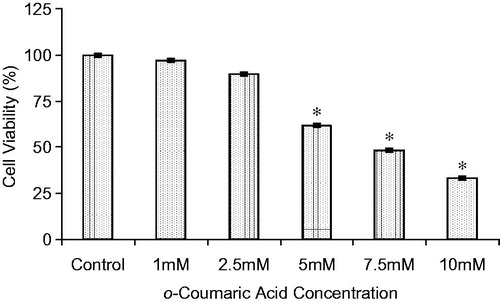
The effect of OCA on the expression of the main CYP isozymes participating in both drug and carcinogen metabolism was determined in this study (). Densitometric scanning of western blots revealed that the CYP1A2 protein level was increased by 52% in OCA-treated cells relative to untreated cells (). Similarly, the level of CYP1A2 mRNA was increased by 40% following 5 mM OCA treatment (p < 0.05). In addition, CYP2E1 protein levels were also found to be increased by 225% following OCA treatment (). As presented in , the mRNA level of CYP2E1 was increased by 424% in OCA-treated cells. In contrast to CYP2E1 and CYP1A2, the CYP3A4 protein level decreased by 52% in the OCA-treated cells relative to the untreated control cells (, p < 0.05). Correspondingly, the level of CYP3A4 mRNA was significantly decreased by 60% in the OCA-treated cells (). In contrast, with respect to untreated cells, CYP2C9 protein and mRNA levels were increased following OCA treatment (), causing a 110% increase in the protein level (p < 0.05) and a 130% increase in the mRNA level.
Figure 3. Effects of o-coumaric acid (OCA) on CYP1A2 protein and mRNA levels in HepG2 cells. Representative images for (a) immunoblot and (b) RT-PCR (agarose gel) results showing CYP1A2 protein and mRNA expression, respectively. (c) Comparison of CYP1A2 protein and mRNA levels among experimental groups. The bar graphs represent the relative intensity of the bands obtained from western blotting and RT-PCR. The experiments were repeated at least three times. *Significantly different from the respective control value (p < 0.05).
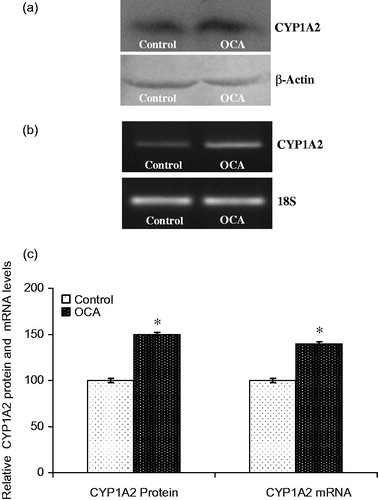
Figure 4. Effects of o-coumaric acid (OCA) on CYP2E1 protein and mRNA levels in HepG2 cells. Representative images for (a) immunoblots and (b) RT-PCR (agarose gel) results showing CYP2E1 protein and mRNA expression, respectively. (c) Comparison of CYP2E1 protein and mRNA levels among experimental groups. The bar graphs show the relative intensity of the bands obtained from western blotting and RT-PCR. The experiments were repeated at least three times. *Significantly different from the respective control value (p < 0.05).
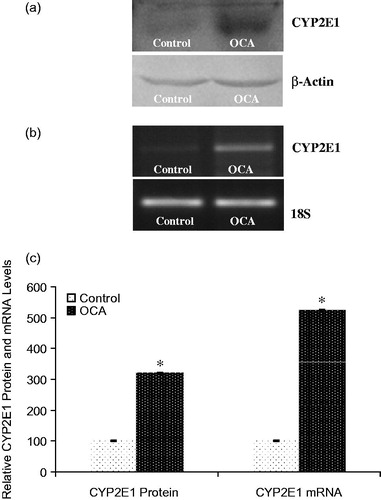
Figure 5. Effects of o-coumaric acid (OCA) on CYP3A4 protein and mRNA levels in HepG2 cells. Representative images for (a) immunoblots and (b) RT-PCR (agarose gel) results showing CYP3A4 protein and mRNA expression, respectively. (c) Comparison of CYP3A4 protein and mRNA levels among experimental groups. The bar graphs represent the relative intensity of the bands obtained from western blotting and RT-PCR. The experiments were repeated at least three times. *Significantly different from the respective control value (p < 0.05).
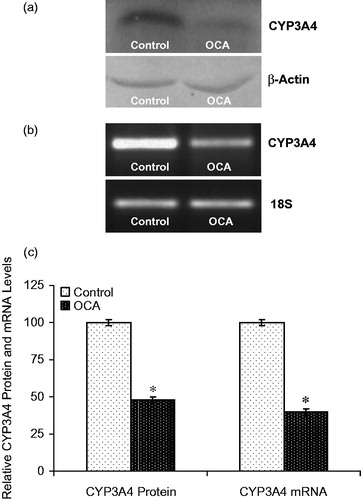
Figure 6. Effects of o-coumaric acid (OCA) on CYP2C9 protein and mRNA levels in HepG2 cells. Representative images for (a) immunoblots and (b) RT-PCR (agarose gel) results showing CYP3A4 protein and mRNA expression, respectively. (c) Comparison of CYP2C9 protein and mRNA levels among experimental groups. The bar graphs show the relative intensity of the bands obtained from western blotting and RT-PCR. The experiments were repeated at least three times. *Significantly different from the respective control value (p < 0.05).
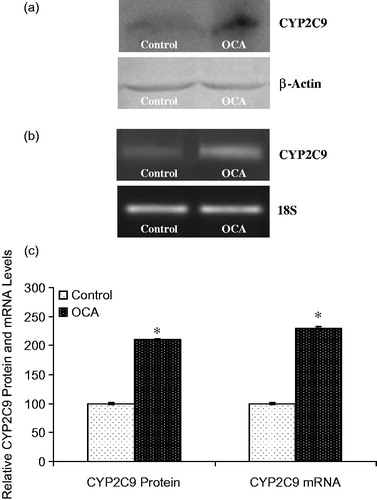
Discussion
Increasing interest in herbal remedies is being observed in Western countries. However, toxicity to the heart, liver, blood, kidneys, central nervous system, and skin and carcinogenesis due to adverse drug reactions and poisoning associated with the use of herbal medicines have also raised (Deng, Citation2002; Klesper & Klepser, Citation1999). The most important factor in the development of these undesired effects of several herbs is the induction and inhibition of CYP-dependent drug-metabolizing enzymes (Zhou et al., Citation2003). This is the first study to reveal the effects of OCA on xenobiotic metabolism and possible drug interactions and carcinogen-activating potential in a human cancer cell line. Moreover, the cytotoxic effect of OCA was determined for the first time in a human hepatocarcinoma (HepG2) cell line.
Human liver-derived cell lines can be ideal models for routine toxicological experiments because of their availability, unlimited life-span, stable phenotype and easy handling. Indeed, HepG2 cells were developed with the intention that they would replace fresh human hepatocytes in preliminary toxicology and drug screening. These cells have an obvious advantage with regard to their availability and experimental reproducibility. Moreover, HepG2 cells display genotypic and phenotypic characteristics that are similar to human hepatocytes (Sassa et al., Citation1987). This cell line also expresses certain drug-metabolizing enzymes, including CYPs (Choi et al., Citation2004; Hewitt & Hewitt, Citation2004). For these reasons, the HepG2 cell line was used throughout this study.
The cytotoxicity of OCA in human cancer was investigated using WST-1 assays. As shown in , OCA treatment caused a concentration-dependent cytotoxic effect in HepG2 cells. Moreover, OCA is able to exert a cytotoxic effect by stimulating multiple processes, including apoptosis, cell cycle regulation and tumor suppression (Sen et al., Citation2013). The profile of human exposure to phenolic acids remains complex, and the estimated daily intake of phenolic acids varies between 25 mg and 1 g/d depending on the diet (Clifford, Citation1999; Scalbert & Williamson, Citation2000; Radtke et al., Citation1998). The OCA concentration used in HepG2 cells in this study, 5 mM (821 µg/ml), was in the range of the human dietary intake value.
OCA treatment of 5 mM in HepG2 cells significantly increased CYP1A2 protein and mRNA levels (). CYP1A2 mRNA levels were reported to increase by 175% in OCA-treated cells relative to untreated control MCF7 cells (Sen et al., Citation2013). In contrast to OCA, ferulic acid and p-coumaric acid treatment decreased CYP1A1 enzyme activity in a human colon adenocarcinoma cell line (Ferguson et al., Citation2005). Conversely, various herbal extracts and phenolics, such as Cyclamen trochopteranthum O. Schwarz (Primulaceae), Salvia miltiorrhiza Burke (Lamiaceae), tanshinone, protocatechuic and tannic acids, caused increase in CYP1A2-related enzyme activity and protein levels (Arslan et al., Citation2011; Kuo et al., Citation2006; Lee et al., Citation2012; Szaefer et al., Citation2003). Among all the cytochrome CYP isoforms, CYP1A is considered to be the most critical for such studies due to its role in the metabolism of carcinogens, mutagens, and environmental pollutants. The results of the present study collectively suggest that the induction of CYP1A2 by OCA may stimulate the metabolic breakdown of many carcinogenic and pro-carcinogenic substrates, such as benzo(a)pyrene and aromatic and heterocyclic amines. It has been shown that when CYP1A2 is induced by cigarette smoke, carcinogenicity or hepatotoxicity due to heterocyclic amines is increased (Koide et al., Citation1999; Nishikawa et al., Citation2002). Therefore, the activation of this metabolic pathway due to OCA exposure may lead to increased organ toxicity, mutagenesis, and carcinogenesis in people.
A densitometric analysis of western blot results and RT-PCR showed that CYP2E1 protein and mRNA levels were significantly increased in OCA-treated cells relative to the control cells (). However, the regulation of CYP2E1 expression is complex, involving transcriptional, post-transcriptional, and post-translational events (Song, Citation1995), and it remains to be elucidated whether the increase in CYP2E1 protein and mRNA resulting from OCA treatment are transcriptional or post-transcriptional. Similar to the results obtained in this study, some other herbs or chemical compounds isolated from herbs induced not only CYP2E1 catalytic activity but also its protein level (Bray et al., Citation2002; Pass et al., Citation1999).
CYP2E1 has received more attention in recent years because of its vital role in the activation of many low-molecular-weight toxic chemicals, such as benzene, carbon tetrachloride, nitrosamines, thioacetamide, and pyridine (Arinç et al., Citation2000, Citation2007; Guengerich et al., Citation1991; Krishnan & Brodeur, Citation1991; Wang et al., Citation2000; Yang & Hong, Citation1995). An increase in toxicity was demonstrated when CYP2E1 inducers were administered 12–24 h prior to exposure to a toxic or carcinogenic chemical (Krishnan & Brodeur, Citation1991; Lorr et al., Citation1984; Yang & Hong, Citation1995). The results of the present study suggest that OCA can stimulate the metabolic activation of N-nitrosodimethylamine, pyridine, benzene, and other toxic chemicals that are metabolized by CYP2E1 by increasing its expression. This may, in turn, result in increased amounts of reactive metabolites, which may further potentiate the risk of organ toxicity, mutagenesis, and malignant transformation in individuals who use OCA as an herbal remedy for liver disease.
The effects of OCA on the protein and mRNA levels of CYP3A4 and CYP2C9 were also determined. The CYP3A4 mRNA and protein levels were decreased (), similar to the reported 75% decrease in the mRNA level in response to OCA treatment in MCF7 cells (Sen et al., Citation2013). The CYP2C9 protein and mRNA levels were increased in HepG2 cells after 24 h of OCA treatment (), whereas the mRNA level was significantly decreased in MCF7 cells (Sen et al., Citation2013). Tissue- or species-specific modifications have also been observed for CYP isozymes due to chemicals and pathophysiological situations, such as diabetes (Koide et al., Citation1999; Nishikawa et al., Citation2002; Song, Citation1995). Indeed, the results of the present work indicate different tissue-specific responses to OCA in HepG2 and MCF7 cell lines. The pregnane X receptor (PXR) and constitutive androstane receptor (CAR) serve as key regulators of CYP3A gene expression (Hiroi et al., Citation1998; Waxman, Citation1999; Willson & Kliewer, Citation2002), and the present data indicated that OCA may affect both, resulting in the inhibition or induction of CAR and PXR transcriptional activity, and thus changes in CYP3A4 and CYP2C9 expression. However, further, more detailed analyses must be performed to confirm this inference.
The CYP3A subfamily is thought to have the greatest overall impact on human pharmacotherapy because it comprises the most abundantly expressed CYPs in the human liver and small intestine and its members possess metabolic activity toward an extremely broad spectrum of xenobiotic substrates, including antibiotics, sedatives, immune system modulators, calcium channel blockers, HIV-directed antiviral agents, and HMG CoA reductase inhibitors (de Wildt et al., Citation2007; Sugimoto et al., Citation2006). The next most important group is the CYP2C subfamily, which accounts for approximately 20% of the entire liver cytochrome P450 content in humans. This family is responsible for the metabolism of approximately 20% of all clinically administered drugs, including anti-coagulants, anti-diabetic agents, non-steroidal anti-inflammatory drugs, and anticonvulsants (Carlile et al., Citation1999). It is well established that the modulation of these cytochrome P450 isozymes by plant extracts, chemicals, or pathophysiological situations results in impaired drug metabolism. For example, the administration of Sophora flavescens Aiton (Leguminosae) extract to rats altered the pharmacokinetic profile of theophylline by modulating CYP isozymes (Ueng et al., Citation2010). Therefore, on the basis of its nondiscriminatory effects on a number of significant drug-metabolizing enzymes, OCA could in theory influence the actions of many medicines. Moreover, the alteration of drug clearance and clinical drug toxicity may be observed due to changes in these CYP isozymes.
Conclusions
In conclusion, based on the observed alterations in CYP3A4 and CYP2C9 protein and mRNA levels, OCA would be expected to modify the metabolism of other essential medications, including antibiotics, antiarrhythmics, immune modulators, steroids, and antiepileptics. On the basis of its nondiscriminatory effects on a number of important drug-metabolizing enzymes, OCA could, in theory, affect the actions of many medicines and may result in food–drug interactions. Therefore, until further clinical drug interaction experiments are completed, the co-administration of drugs with OCA should be avoided. Moreover, the results of the present study suggest that OCA stimulates the metabolic activation of toxic chemicals metabolized by not only CYP2E1 but also CYP1A2 by inducing these enzymes, which results in increased formation of reactive metabolites. This may, in turn, further potentiate the risk of toxicity and carcinogenesis. Therefore, serious precautions should be taken in terms of extensive OCA exposure through diet or supplementation.
Declaration of interest
This study was supported by a grant from The Scientific and Technological Research Council of Turkey (111T011).
Notes
* The preliminary results reported herein were presented at the 19th International Symposium on Microsomes and Drug Oxidations, 12th European Regional ISSX Meeting in Noordwijkaan Zee, The Netherlands, 17–21 June 2012.
References
- Abu Bakar NB, Makahleh A, Saad B. (2012). In-vial liquid–liquid microextraction-capillary electrophoresis method for the determination of phenolic acids in vegetable oils. Anal Chim Acta 742:59–66
- Agus HH, Tekin P, Bayav M, et al. (2009). Drug interaction potential of the seed extract of Urtica urens L. (dwarf nettle). Phytother Res 23:1763–70
- Arinç E, Adali O, Gencler Ozkan AM. (2000). Stimulation of aniline, p-nitrophenol and N-nitrosodimethylamine metabolism in kidney by pyridine pretreatment of rabbits. Arch Toxicol 74:527–32
- Arinç E, Arslan S, Adali O. (2005). Differential effects of diabetes on CYP2E1 and CYP2B4 proteins and associated drug metabolizing enzyme activities in rabbit liver. Arch Toxicol 79:427–33
- Arinç E, Arslan S, Bozcaarmutlu A, Adali O. (2007). Effects of diabetes on rabbit kidney and lung CYP2E1 and CYP2B4 expression and drug metabolism and potentiation of carcinogenic activity of N-nitrosodimethylamine in kidney and lung. Food Chem Toxicol 45:107–18
- Arslan S, Ozgun O, Celik G, et al. (2011). Effects of Cyclamen trochopteranthum on hepatic drug-metabolizing enzymes. Arch Biol Sci 63:545–55
- Bray BJ, Perry NB, Menkes DB, Rosengren RJ. (2002). St. John's wort extract induces CYP3A and CYP2E1 in the Swiss Webster mouse. Toxicol Sci 66:27–33
- Carlile DJ, Hakooz N, Bayliss MK, Houston JB. (1999). Microsomal prediction of in vivo clearance of CYP2C9 substrates in humans. Br J Clin Pharmacol 47:625–35
- Cho YA, Lee W, Choi JS. (2012). Effects of curcumin on the pharmacokinetics of tamoxifen and its active metabolite, 4-hydroxytamoxifen, in rats: Possible role of CYP3A4 and P-glycoprotein inhibition by curcumin. Pharmazie 67:124–30
- Choi S, Nishikawa M, Sakoda A, Sakai Y. (2004). Feasibility of a simple double-layered coculture system incorporating metabolic processes of the intestine and liver tissue: Application to the analysis of benzo[a]pyrene toxicity. Toxicol In Vitro 18:393–402
- Chon SU, Kim JD. (2002). Biological activity and quantification of suspected allelochemicals from alfalfa plant parts. J Agric Crop Sci 188:281–5
- Chung WG, Sen A, Wang-Buhler JL, et al. (2004). cDNA-directed expression of a functional zebrafish CYP1A in yeast. Aquat Toxicol 70:111–21
- Clifford MN. (1999). Chlorogenic acids and other cinnamates – Nature, occurrence and dietary burden. J Sci Food Agric 79:362–72
- Dai J, Mumper RJ. (2010). Plant phenolics: Extraction, analysis and their antioxidant and anticancer properties. Molecules 15:7313–52
- De P, Baltas M, Bedos-Belval F. (2011). Cinnamic acid derivatives as anticancer agents – A review. Curr Med Chem 18:1672–703
- De Wildt SN, Berns MJ, Van Den Anker JN. (2007). 13C-erythromycin breath test as a noninvasive measure of CYP3A activity in newborn infants: A pilot study. Ther Drug Monit 29:225–30
- Deng JF. (2002). Clinical and laboratory investigations in herbal poisonings. Toxicology 181–2:571–6
- Detampel P, Beck M, Krahenbuhl S, Huwyler J. (2012). Drug interaction potential of resveratrol. Drug Metab Rev 44:253–65
- Dos Santos SC, Krueger CL, Steil AA, et al. (2006). LC characterisation of guaco medicinal extracts, Mikania laevigata and M. glomerata, and their effects on allergic pneumonitis. Planta Med 72:679–84
- Ferguson LR, Zhu ST, Harris PJ. (2005). Antioxidant and antigenotoxic effects of plant cell wall hydroxycinnamic acids in cultured HT-29 cells. Mol Nutr Food Res 49:585–93
- Guengerich FP, Kim DH, Iwasaki M. (1991). Role of human cytochrome P-450 IIE1 in the oxidation of many low molecular weight cancer suspects. Chem Res Toxicol 4:168–79
- Hewitt NJ, Hewitt P. (2004). Phase I and II enzyme characterization of two sources of HepG2 cell lines. Xenobiotica 34:243–56
- Hiroi T, Imaoka S, Chow T, Funae Y. (1998). Tissue distributions of CYP2D1, 2D2, 2D3 and 2D4 mRNA in rats detected by RT-PCR. Biochim Biophys Acta 1380:305–12
- Hsu CL, Wu CH, Huang SL, Yen GC. (2009). Phenolic compounds rutin and o-coumaric acid ameliorate obesity induced by high-fat diet in rats. J Agric Food Chem 57:425–31
- Huang WY, Cai YZ, Zhang Y. (2010). Natural phenolic compounds from medicinal herbs and dietary plants: Potential use for cancer prevention. Nutr Cancer 62:1–20
- Klesper TB, Klesper ME. (1999). Unsafe and potentially safe herbal therapies. Am J Health Syst Pharm 56:125–38
- Koide A, Fuwa K, Furukawa F, et al. (1999). Effect of cigarette smoke on the mutagenic activation of environmental carcinogens by rodent liver. Mutat Res 428:165–76
- Krishnan K, Brodeur J. (1991). Toxicological Consequences of Combined Exposure to Environmental Pollutants. Lafayette, USA: ACES Pub
- Kuo YH, Lin YL, Don MJ, et al. (2006). Induction of cytochrome P450-dependent monooxygenase by extracts of the medicinal herb Salvia miltiorrhiza. J Pharm Pharmacol 58:521–7
- Laemmli UK. (1970). Cleavage of structural proteins during the assembly of the head of bacteriophage T4. Nature 227:680–5
- Landete JM. (2012). Updated knowledge about polyphenols: Functions, bioavailability, metabolism, and health. Crit Rev Food Sci Nutr 52:936–48
- Lee SJ, Hedstrom OR, Fischer K, et al. (2001). Immunohistochemical localization and differential expression of cytochrome P450 3A27 in the gastrointestinal tract of rainbow trout. Toxicol Appl Pharmacol 177:94–102
- Lee WY, Zhou X, Or PM, et al. (2012). Tanshinone I increases CYP1A2 protein expression and enzyme activity in primary rat hepatocytes. Phytomedicine 19:169–76
- Li C, Lee MY, Choi JS. (2010). Effects of silybinin, CYP3A4 and P-glycoprotein inhibitor in vitro, on the bioavailability of loratadine in rats. Pharmazie 65:510–14
- Lorr NA, Miller KW, Chung HR, Yang CS. (1984). Potentiation of the hepatotoxicity of N-nitrosodimethylamine by fasting, diabetes, acetone, and isopropanol. Toxicol Appl Pharmacol 73:423–31
- Markowitz JS, Devane CL, Boulton DW, et al. (2000). Effect of St. John's wort (Hypericum perforatum) on cytochrome P-450 2D6 and 3A4 activity in healthy volunteers. Life Sci 66:133–9
- Maruyama M, Matsunaga T, Harada E, Ohmori S. (2007). Comparison of basal gene expression and induction of CYP3As in HepG2 and human fetal liver cells. Biol Pharm Bull 30:2091–7
- Nishikawa A, Furukawa F, Miyauchi M, et al. (2002). Enhancement by cigarette smoke exposure of 2-amino-3,8-dimethylimidazo[4,5-f]quinoxaline-induced rat hepatocarcinogenesis in close association with elevation of hepatic CYP1A2. Jpn J Cancer Res 93:24–31
- Ozkarsli M, Sevim H, Sen A. (2008). In vivo effects of Urtica urens (dwarf nettle) on the expression of CYP1A in control and 3-methylcholanthrene-exposed rats. Xenobiotica 38:48–61
- Pass GJ, Mclean S, Stupans I. (1999). Induction of xenobiotic metabolising enzymes in the common brushtail possum, Trichosurus vulpecula, by Eucalyptus terpenes. Comp Biochem Physiol C Pharmacol Toxicol Endocrinol 124:239–46
- Perrone D, Farah A, Donangelo CM, et al. (2008). Comprehensive analysis of major and minor chlorogenic acids and lactones in economically relevant Brazilian coffee cultivars. Food Chem 106:859–67
- Piscitelli SC, Burstein AH, Welden N, et al. (2002). The effect of garlic supplements on the pharmacokinetics of saquinavir. Clin Infect Dis 34:234–8
- Plazonic A, Bucar F, Males Z, et al. (2009). Identification and quantification of flavonoids and phenolic acids in burr parsley (Caucalis platycarpos L.), using high-performance liquid chromatography with diode array detection and electrospray ionization mass spectrometry. Molecules 14:2466–90
- Radtke J, Linseisen J, Wolfram G. (1998). Phenolic acid intake of adults in a Bavarian subgroup of the national food consumption survey. Z Ernahrungswiss 37:190–7
- Robbins RJ. (2003). Phenolic acids in foods: An overview of analytical methodology. J Agric Food Chem 51:2866–87
- Rowin J, Lewis SL. (1996). Spontaneous bilateral subdural hematomas associated with chronic Ginkgo biloba ingestion. Neurology 46:1775–6
- Sassa S, Sugita O, Galbraith RA, Kappas A. (1987). Drug metabolism by the human hepatoma cell, Hep G2. Biochem Biophys Res Commun 143:52–7
- Scalbert A, Williamson G. (2000). Dietary intake and bioavailability of polyphenols. J Nutr 130:2073S–85
- Sen A, Arinç E. (1998). Preparation of highly purified cytochrome P4501A1 from leaping mullet (Liza saliens) liver microsomes and its biocatalytic, molecular and immunochemical properties. Comp Biochem Physiol C Pharmacol Toxicol Endocrinol 121:249–65
- Sen A, Atmaca P, Terzioglu G, Arslan S. (2013). Anticarcinogenic effect and carcinogenic potential of the dietary phenolic acid: o-Coumaric acid. Nat Prod Commun 8:1269–74
- Sen A, Ozgun O, Arinç E, Arslan S. (2012). Diverse action of acrylamide on cytochrome P450 and glutathione S-transferase isozyme activities, mRNA levels and protein levels in human hepatocarcinoma cells. Cell Biol Toxicol 28:175–86
- Song BJ. (1995). Gene Structure and Multiple Regulations of the Ethanol-Inducible Cytochrome P4502E1 (CYP2E1) Subfamily. New Jersey, USA: Humana Press
- Sugimoto K, Araki N, Ohmori M, et al. (2006). Interaction between grapefruit juice and hypnotic drugs: Comparison of triazolam and quazepam. Eur J Clin Pharmacol 62:209–15
- Szaefer H, Jodynis-Liebert J, Cichocki M, et al. (2003). Effect of naturally occurring plant phenolics on the induction of drug metabolizing enzymes by o-toluidine. Toxicology 186:67–77
- Ueng YF, Tsai CC, Lo WS, Yun CH. (2010). Induction of hepatic cytochrome P450s by the herbal medicine Sophora flavescens extract in rats: Impact on the elimination of theophylline. Drug Metab Pharmacokinet 25:560–7
- Wang T, Shankar K, Ronis MJ, Mehendale HM. (2000). Potentiation of thioacetamide liver injury in diabetic rats is due to induced CYP2E1. J Pharmacol Exp Ther 294:473–9
- Waxman DJ. (1999). P450 gene induction by structurally diverse xenochemicals: Central role of nuclear receptors CAR, PXR, and PPAR. Arch Biochem Biophys 369:11–23
- Wilkening S, Stahl F, Bader A. (2003). Comparison of primary human hepatocytes and hepatoma cell line Hepg2 with regard to their biotransformation properties. Drug Metab Dispos 31:1035–42
- Willson TM, Kliewer SA. (2002). PXR, CAR and drug metabolism. Nat Rev Drug Discov 1:259–66
- Yang CS, Hong JY. (1995). Molecular Aspects of Cytochrome P4502E1 and its Roles in Chemical Toxicology. Heidelberg, Germany: Springer-Verlag
- Yeh CT, Huang SM, Yen GC. (2005). Induction of phenolsulfotransferase expression by phenolic acids in human hepatoma HepG2 cells. J Agric Food Chem 53:4766–73
- Zhou S, Gao Y, Jiang W, et al. (2003). Interactions of herbs with cytochrome P450. Drug Metab Rev 35:35–98