Abstract
Context: Wound healing is a consequence of a complex process involving inflammatory, proliferative, and remodeling phases. Naringin, a flavanone glycoside, is associated with modulation of various oxido-inflammatory and growth factors.
Aim: The aim of this study is to evaluate the wound-healing activity of naringin ointment formulation (NOF) on experimental wound models.
Materials and methods: A soft paraffin-based cream containing 1, 2, and 4% (w/w) naringin was formulated and evaluated for physicochemical characters. Excision wounds and incisions wounds were used to study the topical effect of NOF for 20 d (once a day) on various biochemical, molecular, and histological parameters.
Results: NOF (2 and 4%, w/w) treatment showed a significant decrease (p < 0.05) in wound area and epithelization period whereas the rate of wound contraction increased significantly (p < 0.05). The altered levels of oxido-nitrosative stress (SOD, GSH, MDA, MPO, and NO) were significantly (p < 0.05) restored by NOF. Treatment produced a significant increase (p < 0.05) in tensile strength, hydroxyproline content, and protein content. TNF-α, IL-1β, IL-6, IL-8, NF-κB, smad-7, and Bax mRNA expression were significantly down-regulated (p < 0.05) by NOF, whereas polymerase gamma (pol-γ), smad-3, VEGF and TGF-β, and collagen-1 mRNA expressions were significantly up-regulated (p < 0.05) by NOF. Histological alterations in wound skin were also restored by NOF.
Conclusion: NOF exerts wound healing potential via down-regulated expression of inflammatory (NF-κB, TNF-α, and ILs), apoptotic (pol-γ and Bax), and up-regulated growth factor (VEGF and TGF-β) expression, thus modulating collagen-1 expression to induce angiogenesis leading to wound healing.
Introduction
Wounds are inescapable parts of life which arise due to any agent that induces stress and injury. Wound healing is an intricate process which involves an array of biochemical and cellular processes (Goswami et al., Citation2014). Healing of wounds is necessary for survival and it represents an attempt to maintain normal anatomical structure and function (Bhat et al., Citation2007). In normal skin, the epidermis and dermis exist in steady-state equilibrium, forming a protective barrier against the external environment (Diegelmann & Evans, Citation2004).
A wound advocates the twinge and grounds of prolific inflammation. It is defined as commotion of the normal structure, function, and architecture of skin (Atiyeh et al., Citation2002). An acute wound in normal wound physiology and healing is anticipated to progress through the normal stages of wound healing, through an orderly sequence of physiological events that include homeostasis, inflammation, epithelialization, fibroplasia, and maturation (Meyer et al., Citation2003). Whereas a chronic wound is defined as one that is physiologically impaired (Golinko et al., Citation2009) and when this process is tainted or subdued, a chronic wound may develop disorders such as peripheral artery disease, diabetes, venous insufficiency, nutritional deficiencies, and other disease states (Diegelmann & Evans, Citation2004; Shivakumar et al., Citation2014). Chronic ulceration pedestrian affects the lower extremities with a prevalence that ranges between 0.18 and 1.3% in the adult population (Goswami et al., Citation2014).
Wound management is one of the major clinical dilemmas due to its early and late complications presenting an intermittent basis of morbidity and mortality (Natarajan et al., Citation2000). Global socio-economic impact of wounds is reported due to high rate of occurrence, complication with ageing, difficult to heal, and chronic wound associated with disease and abnormalities such as diabetes, pressure ulcer, and infection (Ghosh et al., Citation2012a–d). Estimation of chronic wounds affects 120 per 100 000 people between 45 and 65 years and rises to 800 per 100 000 people >75 years of age (Robson et al., Citation2001).
Healing is multifaceted process and includes fundamental four highly integrated and overlapping phases of cellular and biochemical activities: inflammation, new tissue formation, and remodeling (Gosain & DiPietro, Citation2004). Inflammation is early event that occurs immediately after tissue damage. Mechanisms of the coagulation cascade, inflammatory pathways, and immune system are desirable to avert enduring blood and fluid losses and to thwart infection. Homeostasis is assisted by the formation of a platelet plug which is further preceded by a fibrin matrix that serves as a scaffold for infiltrating cells. Neutrophils degranulate the platelets and form bacterial degradation (Grose & Werner, Citation2004). Mononuclear leukocytes collect into macrophages within 2–3 d and the conversion is heralded by several events, including secretion of vimentin, which is a structural filament protein involved in wound healing (Ross et al., Citation1970).
The second phase of wound repair is new tissue formation which occurs 2–10 d after injury and is characterized by cellular proliferation and migration of different cell types (Hart, Citation2002). The primary event is the transport of keratinocytes over the injured dermis. New blood vessels then form by angiogenesis and the sprouts of capillaries allied with fibroblasts and macrophages reinstate the fibrin matrix with granulation tissue, which serves as a new substrate for keratinocyte migration at later stages of the repair process (Tonnesen et al., Citation2000). The keratinocytes that are found after the leading edge proliferate and mature and, finally, restore the barrier function of the epithelium. The most essential positive regulators of angiogenesis are vascular endothelial growth factor-A (VEGF-A) and fibroblast growth factor 2 (FGF2; also known as bFGF; Werner & Grose, Citation2003). In the latter part of this stage, fibroblasts are attracted from the edge of the wound and are stimulated by macrophages, and some differentiate into myofibroblasts (Opalenik & Davidson, Citation2005). Myofibroblasts are contractile cells that, over time, bring the edges of a wound together. Fibroblasts and myofibroblasts interact and produce an extracellular matrix, mainly in the form of collagen, which ultimately form the bulk of the mature scar (Werner et al., Citation2007).
The final stage of wound repair is remodeling, usually beginning 2–3 weeks after injury and could last for a year or more (Monaco & Lawrence, Citation2003). During this stage, the entire process of healing stops. Most of the endothelial cells, macrophages, and myofibroblasts undergo apoptosis or exile from the wound, parting a heap that contains few cells and consists mostly of collagen and other extracellular matrix proteins. Epithelial–mesenchymal interactions most likely incessantly authorize skin integrity and homeostasis (Szabowski et al., Citation2000). In addition, over 6–12 months, the extracellular matrix is actively remodeled from mainly a type III collagen backbone to one predominantly composed of type I collagen (Lovvorn et al., Citation1999). This process is carried out by matrix metalloproteinases that are secreted by fibroblasts, macrophages, and endothelial cells, and it strengthens the repaired tissue.
Management of poorly healing wounds is a complicated and expensive program. Research on drugs that increase wound healing is a developing area in modern biomedical sciences (Bairy & Rao, Citation2001; Gosavi et al., Citation2011a; Shirwaikar et al., Citation2003). The central dogma for optimal wound healing includes decreased tissue damage, removal of non-viable tissue, maximizing tissue perfusion oxygenation, and proper nutrition (Pierce et al., Citation1995). In various conditions such as severe burns, trauma, diabetic decubitus, and venus stasis ulcers, there is a need of rapid and proper healing (Albina, Citation1994). Hence there is a need for rapid wound healing treatment with minimal pain, discomfort, and scarring for patients within the physiologic environment that is favorable to tissue repair and regeneration (Bowler, Citation2002).
Plant flavonoids are one of the promising therapeutic drugs effective against an ample range of free radical-mediated diseases. Flavonoids are one of the most copious and prevalent groups of naturally occurring antioxidants and serve as potent inhibitors of lipid peroxidation in the biological membrane of the citrus family (Kandhare et al., Citation2012b, Citation2014a). They are also well documented to facilitate rapid wound healing as they possess excellent antimicrobial, antioxidant, anti-inflammatory, and astringent properties (Tsuchiya et al., Citation1996). Naringin (4′,5,7-trihydroxy flavanone 7-rhamnoglucoside) is a major and active flavanone glycoside which was reported to possess various pharmacological properties including antimicrobial, antimutagenic, anti-inflammatory, cholesterol lowering, antioxidant, antiulcer, anticancer, antiatherogenic, hepatoprotective, cardioprotective, renoprotective, and neuroprotective effects (Adil et al., Citation2014; Jeon et al., Citation2001; Kandhare et al., Citation2012b, Citation2014a; Kumar et al., Citation2014). It has inhibitory potential against tumor necrosis factor-α (TNF-α) and transforming growth factor-β (TGF-β) which is involved in the pathogenesis of various interstitial diseases (Chen et al., Citation2013). It was shown to possess anti-apoptotic potential via modulating the Bax-Bcl-2 pathway thus decreased DNA damage and neuronal apoptosis (Gopinath et al., Citation2011). It has been documented that free radicals are dynamically involved in the delayed healing process (Patil et al., Citation2011, Citation2012d). The action of naringin in wound healing may be due to antioxidant and radical trapping and scavenging. Moreover, ointment is considered as the simplest and convenient for application leading to high compliance in using the medication dosage form (Patil et al., Citation2012d). Hence, the aim of present investigation was to unravel therapeutic potential of naringin ointment formulation with an excision and incision wound model by assessing various biochemical, molecular, and histological parameters in laboratory rats.
Materials and methods
Animals
Adult male Wistar rats (180–200 g) and male Swiss albino mice (18–22 g) were purchased from the National Institute of Biosciences, Pune, India. They were maintained at 24 ± 1 °C, with a relative humidity of 45–55% and 12:12 h dark/light cycle. The animals had free access to standard pellet chow (Pranav Agro Industries Ltd., Sangli, India) and water throughout the experimental protocol. All experiments were carried out between 09:00 and 17:00 h. The experimental protocol was approved by the Institutional Animal Ethics Committee (IAEC) of Poona College of Pharmacy, Pune, India (CPCSEA/83/2014) and performed in accordance with the guidelines of Committee for Control and Supervision of Experimentation on Animals (CPCSEA).
Chemicals
Naringin was purchased from Sigma Chemical Co. (St Louis, MO). 1,1′,3,3′-Tetraethoxypropane, crystalline beef liver catalase, reduced glutathione (GSH), and 5,5′-dithiobis (2-nitrobenzoic acid) were purchased from S.D. Fine Chemicals, Mumbai, India. Total RNA Extraction kit and One-step RT-PCR kit were purchased from MP Biomedicals India Private Limited, Mumbai, Maharashtra, India.
Ointment preparation for topical application
Naringin ointment (1, 2, and 4%) for topical application was prepared as described previously (Patil et al., Citation2011, Citation2012d).
Physicochemical evaluations
Preliminary evaluation of naringin ointment formulations including pH, viscosity, spreadability, acute skin irritation study, and extrudability at different concentrations was carried out as described by Patil et al. (Citation2011, Citation2012d).
Excision wound model
Excision wounds were used to study the rate of contraction of wound and epithelization (Patil et al., Citation2011). Animals were anaesthetized with 80 mg/kg dose of ketamine (i.p.) and the back hairs of the animals were depilated by shaving. An impression was made on the back of neck on the anaesthetized rat. Excision wounds sized 300 mm2 and 2 mm depth were made by cutting out layer of the skin from the shaven area. Hemostasis was achieved by blotting the wound with cotton swab soaked in normal saline. The entire wound was left open. The study comprised the following different groups of nine animals in each groups and the treatment was done topically in all the cases:
Group I: Normal animals (N): did not receive injury for wound formation.
Group II: Vehicle control animals (VC): received injury for wound formation but did not receive any drug treatment.
Group III: FSC-treated animals (FSC): received injury for wound formation and treatment with framycine sulfate ointment (1% w/w).
Group IV: Naringin (1% w/w)-treated animals [N (1%)]: received injury for wound formation and treatment with naringin ointment (1% w/w).
Group V: Naringin (2% w/w)-treated animals [N (2%)]: received injury for wound formation and treatment with naringin ointment (2% w/w).
Group VI: Naringin (4% w/w)-treated animals [N (4%)]: received injury for wound formation and treatment with naringin ointment (4% w/w).
Measurement of wound area
The progressive changes in wound area were monitored with a camera (Fuji, S20 Pro, Japan) on predetermined days, i.e., 0, 4, 8, 12, 16, and 20. Later on, the wound area was measured by using image J software (NIH, Bethesda, MD) and Adobe Photoshop to determine area.
Measurement of wound contraction
Wound contraction was calculated as percentage of the reduction in original wound area size using formula as reported elsewhere (Patil et al., Citation2012d).
Measurement of wound index
Wound index was measured daily with an arbitrary scoring system as reported elsewhere (Patil et al., Citation2012d).
Determination of period of epithelization
Falling of scab leaving no raw wound behind was taken as an end point of complete epithelization and the days required for this were taken as a period of epithelization.
Endogenous antioxidant enzymes in wound
The skin samples from four animals were cut into small pieces, placed in chilled 0.25 M sucrose solution and blotted on a filter paper. The tissues were then homogenized in 10% chilled Tris hydrochloride buffer (10 mM, pH 7.4) by tissue homogenizer (Remi Motors, Mumbai, India) and centrifuged at 5200g for 15 min at 0 °C using an Eppendorf 5810-R high speed cooling centrifuge. The clear supernatant was used for the assays of various antioxidant enzymes. Supernatant of homogenates was employed to estimate superoxide dismutase (SOD), reduced glutathione (GSH), lipid peroxidation (MDA content), and nitric oxide (NO) as described previously (Kandhare et al., Citation2011a, Citation2012e, Citation2013a; Gosavi et al., Citation2012a).
Myeloperoxidase activity
Myeloperoxidase (MPO) activity was measured in tissues by a procedure similar to the previously documented method (Kandhare et al., Citation2013c).
Determination of TNF-α, IL-1β, IL-6, IL-8, NF-κB, smad-3, smad-7, VEGF, polymerase gamma (pol-γ), TGF-β, and BAX by reverse transcriptase PCR in skin
The levels of mRNA were analyzed in skin tissue using a reverse transcription (RT)-PCR approach as described previously (Kandhare et al., Citation2014b; Visnagri et al., Citation2014). The primer sequences for tumor necrosis factor-α (TNF-α), interleukin-1 beta (IL-1β), IL-6, IL-8, smad-3, smad-7, nuclear factor kappa-light-chain-enhancer of activated B cells (NF-κB), vascular endothelial growth factor (VEGF), pol γ, transforming growth factor-beta (TGF-β), Bcl-2-associated X protein (BAX), and β-actin are presented in . Amplification of β-actin served as a control for sample loading and integrity. PCR products were detected by electrophoresis on a 1.5% agarose gel containing ethidium bromide. The size of amplicons was confirmed using a 100-bp ladder as a standard size marker. The amplicons were visualized and images were captured using a gel documentation system (Alpha Innotech Inc., San Leandro, CA). Expression of all the genes was assessed by generating densitometry data for band intensities in different sets of experiments and was generated by analyzing the gel images on the Image J program (Version 1.33, Wayne Rasband, NIH, Bethesda, MD) semi-quantitatively. The band intensities were compared with constitutively expressed β-actin. The intensity of mRNAs was standardized against that of the β-actin mRNA from each sample, and the results were expressed as PCR-product/β-actin mRNA ratios.
Table 1. Primer sequences for TNF-α, IL-1β, IL-6, IL-8, NF-κB, smad-3, smad-7, VEGF, pol-γ, TGF-β, Bax, collagen-1, and β-actin.
Histopathological examination
A specimen sample of tissue was isolated from the skin of each group of rat and collected at the end of the experiment to evaluate the histopathological alterations. Samples were fixed in 10% buffered formalin, processed, and blocked with paraffin and then sectioned into 5 μm and stained with hematoxylin and eosin (H & E). Photomicrographs were captured at a magnification of 100×. Re-epithelization or ulcus in epidermis, fibroblast proliferation, mononuclear and polymorphonuclear cells, neovascularization, and collagen depositions in dermis were analyzed to score the epidermal or dermal re-modeling. At the end of the examination, all the wound healing processes were combined and staged for wound healing phases as inflammation, proliferation, and re-modeling in both groups.
Incision wound model
All the animals were anaesthetized with ketamine and the back hair of the rats was shaved by using a shaving machine. Six centimeter long, two linear-paravertebral incisions were made with a sterile surgical blade through the full thickness of the skin at the distance of 1.5 cm from the midline of each side of the vertebral column. The wounds were closed with three surgical interrupted sutures of 1 cm apart (Patil et al., Citation2011). All the sutures used in the experiments were non-absorbable braided non-capillary and siliconized. The study comprised two different groups of nine animals in each groups and the treatment was done topically in all the cases:
Group I: Normal animals (N): did not receive injury for wound formation.
Group II: Vehicle control animals (VC): received injury for wound formation but did not receive any drug treatment.
Group III: FSC-treated animals (FSC): received injury for wound formation and treatment with framycine sulfate ointment (1% w/w).
Group IV: Naringin (1% w/w)-treated animals [N (1%)]: received injury for wound formation and treatment with naringin ointment (1% w/w).
Group V: Naringin (2% w/w)-treated animals [N (2%)]: received injury for wound formation and treatment with naringin ointment (2% w/w).
Group VI: Naringin (4% w/w)-treated animals [N (4%)]: received injury for wound formation and treatment with naringin ointment (4% w/w).
Measurement of tensile strength
On the 10th day, the rats were sacrificed and their tensile strength was measured as described previously (Akkol et al., Citation2009). Briefly, all the rats were anaesthetized with ketamine, and sutures were gently pulled out. Animals were mounted on a tensiometer. Both ends of skin were fixed with the help of a pair of steel clips. One clip was hanging on a stand and a polyethylene bottle was then filled with water gradually until the wound was broken at the site of wound. The amount of water required to break the wound was noted and expressed as tensile strength of wound in grams.
Estimation of collagen (hydroxyproline content)
Measurement of hydroxyproline level was carried out according to a method described elsewhere (Sarma et al., Citation1990).
Estimation of total protein
Protein concentration was estimated according to the method of Lowry et al. (Citation1951), using BSA (bovine serum albumin) as a standard.
Determination of collagen-1 by reverse transcriptase PCR in skin
The levels of mRNA were analyzed in skin tissue using a RT-PCR approach as described previously (Kandhare et al., Citation2014b; Visnagri et al., Citation2014). The primer sequences for collagen-1 and β-actin are presented in . Amplification of β-actin served as a control for sample loading and integrity. PCR products were detected by electrophoresis on a 1.5% agarose gel containing ethidium bromide. The size of amplicons was confirmed using a 100-bp ladder as a standard size marker. The amplicons were visualized and images were captured using a gel documentation system (Alpha Innotech Inc., San Leandro, CA). Expression of all the genes was assessed by generating densitometry data for band intensities in different sets of experiments and was generated by analyzing the gel images on the Image J program (Version 1.33, Wayne Rasband, NIH, Bethesda, MD) semi-quantitatively. The band intensities were compared with constitutively expressed β-actin. The intensity of mRNAs was standardized against that of the β-actin mRNA from each sample, and the results were expressed as PCR-product/β-actin mRNA ratio.
Statistical analysis
All the results were expressed as mean ± SEM. Data analysis was performed using GraphPad Prism 5.0 software (GraphPad, San Diego, CA). Data of biochemical parameters were analyzed using one-way ANOVA following that Dunnett's test was applied for post hoc analysis. Data of wound area and % wound contraction were analyzed using two-way repeated ANOVA, and Bonferroni's test was applied for post hoc analysis. The gel image was analyzed by using the Image J program (Version 1.33, Wayne Rasband, National Institutes of Health (NIH), Bethesda, MD) semi-quantitatively. A value of p < 0.05 was considered to be statistically significant.
Results
Physicochemical evaluations of different formulations of naringin ointment
The pH of naringin ointment (1, 2, and 4%) was 6.55 ± 0.12, 6.84 ± 0.21, and 7.45 ± 0.15, respectively, which lies in the normal pH range of human skin, whereas the viscosity was 14.2 ± 0.52, 15.01 ± 1.22, and 16.78 ± 1.84 cps, respectively, which indicated that as the torque increases, shear stress also increases. The spread ability time of naringin ointment (1, 2, and 4%) was 23.11 ± 1.02, 23.15 ± 0.89, and 24.52 ± 1.11 s, respectively, which indicates they were nearly identical in the terms of applicability or spreading capacity (). Acute skin irritation study of naringin ointment did not produce any skin irritation, i.e., erythema and edema, for about a week when applied over the skin. Naringin ointment also showed good homogeneity and extrudability.
Table 2. Physicochemical evaluations of different formulations of naringin ointment.
Effect of naringin ointment treatment on wound area, rate of wound contraction and CT50 of rats
The effect of naringin ointment (1, 2, and 4%, w/w) treatment on the various phases of wound healing is depicted in . Treatment with naringin ointment (1, 2, and 4% w/w) resulted in a significance decrease (p < 0.05) in wound area as compared with vehicle control group animals. The % wound contraction rate was increased significantly (p < 0.05) in naringin ointment (1, 2, and 4% w/w)-treated animals as compared with vehicle control group animals (). FSC (1% w/w) treatment also significantly (p < 0.05) decreased wound area and significantly (p < 0.05) increased the rate of wound contraction when compared with vehicle control group animals. The CT50 value for vehicle control rats was 58.44 d indicating a delayed wound healing process. CT50 values for naringin ointment (1, 2, and 4% w/w) treatment groups were 25.03, 15.19, and 9.81 d, respectively. The CT50 value for the FSC (1% w/w)-treated group was 9.12 ().
Figure 2. Effect of naringin ointment treatment (1, 2, and 4%) on wound area (mm2) (A) and rate of wound contraction (%) (B) in rats. Data are expressed as mean ± SEM and analyzed by two-way analysis of variance followed by Bonferroni's test. *p < 0.05 as compared with the vehicle control group and $p < 0.05 as compared with one another. VC, vehicle control; FSC (1%), framycine sulfate ointment (1% w/w)-treated group; N (1%), naringin ointment (1%, w/w)-treated group; N (2%), naringin ointment (2%, w/w)-treated group; N (4%), naringin ointment (4%, w/w)-treated group.
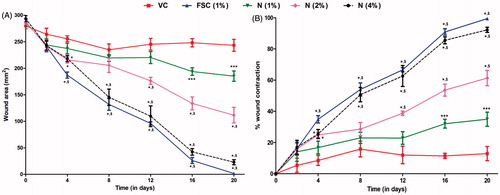
Table 3. Effect of naringin ointment treatment on the period of epithelization, wound index, scar width, CT-50, tensile strength, hydroxyproline content and protein content of rats.
Effect of naringin ointment treatment on period of epithelization, wound index, scar width, tensile strength, hydroxyproline content, and protein content of rats
There was significant decreased (p < 0.05) in the period of epithelization, wound index, and scar width of naringin ointment (2 and 4% w/w)-treated groups as compared with the vehicle control group. Tensile strength was increased significantly (p < 0.05) in naringin ointment (2 and 4% w/w)-treated groups as compared with the vehicle control group. Hydroxyproline and protein contents were decreased significantly (p < 0.05) in the vehicle control group as compared with the normal group. This decreased hydroxyproline and protein level in wound tissue was significantly restored (p < 0.05) by the naringin ointment (2 and 4% w/w)-treated group as compared with the vehicle control group. When compared with the vehicle control group, the FSC (1% w/w)-treated group showed significant restoration (p < 0.05) in the altered period of epithelization, wound index, scar width, tensile strength, hydroxyproline content, and protein content ().
Effect of naringin ointment treatment on SOD, GSH, MDA, MPO, and NO levels in wound tissue of rats
There was significant decrease (p < 0.05) in the SOD as well as GSH levels and significant increase (p < 0.05) in the MDA, MPO, as well as NO levels in vehicle control rats as compared with normal rats. Naringin ointment (2% and 4% w/w) treatment showed significant increase (p < 0.05) in the SOD and GSH levels whereas MDA, MPO, as well as NO levels were significantly decreased (p < 0.05) as compared with vehicle control rats. FSC (1% w/w) treatment significantly restored (p < 0.05) the altered level of SOD, GSH, MDA, MPO, and NO as compared with vehicle control rats ().
Table 4. Effect of naringin ointment treatment on SOD, GSH, MDA, MPO, and NO levels in wound tissue of rats.
Effect of naringin ointment treatment on mRNA expression of TNF-α, IL-1β, IL-6, IL-8, NF-κβ, and pol-γ in wound tissue of rats
The mRNA expression of TNF-α, IL-1β, IL-6, IL-8, and NF-κB was significantly (p < 0.05) up-regulated and pol-γ mRNA expression was significantly (p < 0.05) down-regulated in the vehicle control rats as compared with normal rats. Treatment with naringin ointment (2 and 4% w/w) significantly (p < 0.05) down-regulated TNF-α, IL-1β, IL-6, IL-8, and NF-κB mRNA expression as compared with vehicle control rats. When compared with vehicle control rats, pol-γ mRNA expression was significantly (p < 0.05) up-regulated by naringin ointment (4% w/w) treatment. FSC (1%, w/w) treatment significantly (p < 0.05) restored the altered mRNA expression of TNF-α, IL-1β, IL-6, IL-8, NF-κB, and pol-γ as compared with vehicle control rats ().
Figure 3. Effect of naringin ointment treatment (1, 2, and 4%) on mRNA expression of TNF-α, IL-1β, IL-6, IL-8, NF-κB, and pol-γ in wound tissue as determined by relative quantification by reverse transcriptase polymerase chain reaction analysis (A), quantitative representation of mRNA expression of TNF-α (B), IL-1β (C), IL-6 (D), IL-8 (E), NF-κB (F), and Pol-γ (G). Data are expressed as mean ± SEM and analyzed by one-way analysis of variance followed by Tukey's multiple range test. #p < 0.05 as compared with the normal group; *p < 0.05 as compared with the vehicle control group; $p < 0.05 as compared with one another. VC, vehicle control; FSC (1%), framycine sulfate ointment (1%, w/w)-treated group; N (1%), naringin ointment (1%, w/w)-treated group; N (2%), naringin ointment (2% w/w)-treated group; N (4%), naringin ointment (4%, w/w)-treated group.
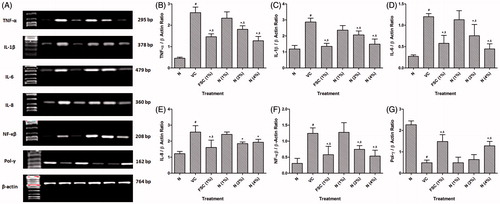
Effect of naringin ointment treatment on mRNA expression of smad-3, smad-7, TGF-β, VEGF, Bax, and collagen-1 in wound tissue of rats
There was significant (p < 0.05) down-regulation in smad-3, TGF-β, VEGF, and collagen-1 mRNA expressions and significant (p < 0.05) up-regulation in smad-7 and Bax mRNA expressions in vehicle control rats as compared with normal rats. Naringin ointment (2 and 4% w/w) treatment significantly (p < 0.05) up-regulated smad-3, TGF-β, VEGF, and collagen-1 mRNA expressions whereas it significantly (p < 0.05) down-regulated smad-7 and Bax mRNA expressions as compared with vehicle control rats. This altered mRNA expression of TGF-β, VEGF, Bax, and collagen-1 was significantly (p < 0.05) restored by the FSC (1% w/w) treatment when compared with vehicle control rats ().
Figure 4. Effect of naringin ointment treatment (1, 2, and 4%) on mRNA expression of smad-3, smad-7, TGF-β, VEGF, Bax, and collagen-1 in wound tissue as determined by relative quantification by reverse transcriptase polymerase chain reaction analysis (A), quantitative representation of mRNA expression of smad-3 (B), smad-7 (C), TGF-β (D), VEGF (E), Bax (F), and collagen-1 (G). Data are expressed as mean ± SEM and analyzed by one-way analysis of variance followed by Tukey's multiple range test. #p < 0.05 as compared with the normal group; *p < 0.05 as compared with the vehicle control group; $p < 0.05 as compared with one another. VC, vehicle control; FSC (1%), framycine sulfate ointment (1% w/w)-treated group; N (1%), naringin ointment (1% w/w)-treated group; N (2%), naringin ointment (2%, w/w)-treated group; N (4%), naringin ointment (4% w/w)-treated group.
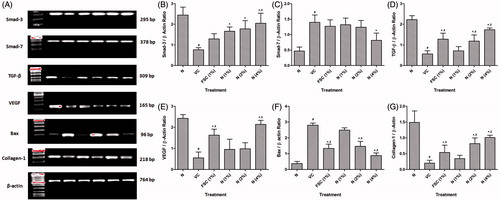
Effect of naringin ointment treatment on histopathological examination of wound tissue of rats
depicted the normal architecture of skin tissue without any inflammatory infiltration and cellular necrosis. Whereas the presence of edema, monocyte cells, cellular necrosis, aggregation of macrophages, and poor collagenation with large numbers of fibroblasts were characteristic features of the delayed in wound healing processes which were observed in the skin tissue from vehicle control rats. It showed ulceration (black arrow), scab formation (yellow arrow), connective tissues formation (white arrow), capillary formation (red arrow), and leukocyte infiltration (blue arrow) (). A similar distinct feature was observed in the skin tissue from the naringin ointment (1% w/w)-treated group revealed by the presence of ulceration (black arrow), scab (yellow arrow), connective tissues (white arrow), and leukocyte infiltration (blue arrow) (). Treatment with naringin ointment (2 and 4% w/w) resulted in decreased inflammation and ulceration (black arrow), increasing the rate of tissue perfusion with capillary formation (red arrow) along with re-epithelization. It also showed reduced macrophage and leukocyte infiltration (blue arrow) (). The skin tissue from the FSC (1% w/w)-treated group also showed decreased ulceration (black arrow) with increased scab formation (yellow arrow) and capillary formation (red arrow). The inflammatory infiltration of leukocyte and macrophages was decreased ( and ).
Figure 5. Photomicrographs of sections of hematoxylin and eosin-stained wound skin tissues. Microscopic image of wound skin of normal rat (A), vehicle control group (B), framycine sulfate ointment (1% w/w)-treated group (C), naringin ointment (1%, w/w)-treated group (D), naringin ointment (2%, w/w)-treated group (E); naringin ointment (4%, w/w)-treated group. Images (×100 magnification) are typical and are representative of each study group.
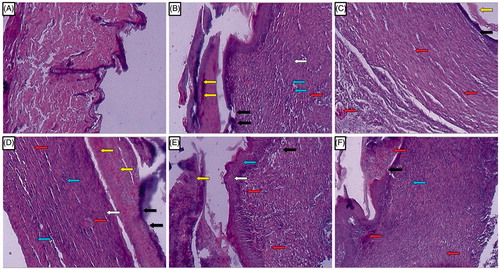
Table 5. Effect of naringin ointment treatment on wound healing processes and healing phases of rats.
Discussion
Wound healing is a complex process of restoring damaged tissue structure to its near normal state. The process of healing essentially depends on the repairing capability of tissue along with the degree of damage and existing vigor of the tissue. No single method is adequate to represent the wound healing process (Goswami et al., Citation2014). Hence, incision and excision wound models are well-established and reproducible animal models that are used regularly in preclinical studies for drugs in wound healing (Kandhare et al., Citation2011b; Patil et al., Citation2011). The multitude of cellular events that occur in animals including cell proliferation, cell migration, contraction and extracellular matrix degradation, synthesis, etc., have great clinical relevance in the wound healing process (Singer & Clark, Citation1999). Moreover, ointment is reported as the most preferential dosage form due to its ease of tropical application (Shetty et al., Citation2008). Therefore, the present investigation was undertaken to evaluate the effect of naringin ointment in an incision as well as an excision wound model in laboratory rats.
For the application of ointment suitability, spread ability and consistency are important physical parameters and these parameters are directly involved in the release of the drug from the base to the local site of action (Patil et al., Citation2012d). Therefore, it is quite important that all rheological and physical parameters must be under the desirable monographic ranges. In the present investigation, 1, 2, and 4% (w/w) of naringin ointment were formulated and evaluated for various pharmaceutical parameters such as rheological properties, skin irritation, and physical characters including spread ability and pH. The results of the present investigation indicated that physicochemical properties of naringin ointment were identical in the terms of applicability or spreading capacity. Moreover, it was found to be safe in terms of a skin irritability test.
Wound healing generally requires support at three levels. First, improving general resistance and support mechanisms that could be obtained from rejuvenative, adaptogenic, palliative, antioxidant, cleansing, detoxifying, buffering, and lubricous activities. Second, stimulating the repair and regenerative mechanisms to prolong cell life, cell migration and binding, remove skin blemishes, and improve tensile strength or elasticity of the skin, improved moisture holding capacity of skin. Third, therapeutic and nutritional activities including anti-inflammatory, antiseptic, and antimicrobial, protein and collagen synthesis and increased stability of biomembranes (Goswami et al., Citation2014). Hence, excision and incision models were utilized to evaluate the healing efficacy of naringin ointments in terms of wound contraction, tensile strength, and hydroxyproline content.
In the excision wound model, assessment of parameters like epithelization period, wound area, wound area contraction rate, wound index, and scar width was reflected as the hallmarks of tissue repairing and regeneration. Closure of wound by neo tissue genesis, effectual functional barrier is the triumph by the various phases of wound healing. In fact, wound healing is an innate phenomenon of regeneration of dermal and epidermal tissue of the body (Panda et al., Citation2009). It is also reported that the rate of contraction determines the period of epithelialization which can be defined as the centripetal movement of the edges of a full-thickness wound to facilitate closure of the defect (Suguna et al., Citation2002). Granulation, collagen maturation, and scar formation are some of the many phases of wound healing which run concurrently, but independent of each other. The inflammatory phase plays a vital role in wound healing and it is characterized by hemostasis and inflammation, whereas the proliferative phase is characterized by epithelization, angiogenesis, and collagen deposition (Nirmala & Karthiyayini, Citation2011). Treatment with naringin significantly decreased wound area and thus increased the rate of wound area contraction which may be by virtue of its anti-inflammatory potential.
Tensile strength represents the force per unit of cross-sectional area that is required for breaking down the wound. It is a hallmark for the intra- and the intermolecular cross-linking of collagen fibers within the newly deposited collage in the subdermal area (Rashed et al., Citation2003). Collagen, fibroblast, edema, and angiogenesis are the major regulatory keys in the granulation of wounded tissue. Shear of platelets by site of injury ignites the release of collagen to advocate the release of various clotting factors along with fibrin to form a provisional mass on which the healing process proceeds. In tissue repairing, collagen deposition is a crucial step which is supposed to be due to release of fibroblast (Nguyen et al., Citation2009). It is also reported that healing may be improved due to the development of fibroblasts because fibroblasts are essential cellular materials involved in the synthesis and deposition of the extracellular matrix (Margaret et al., Citation1998). Increase in the rate of wound contraction might be a result of the enhanced activity of fibroblasts mediated by specialized myofibroblasts found in granulated tissues (Moulin et al., Citation2000). Collagen is, therefore, considered as the predominant extracellular protein synthesized in the rough endoplasmic reticulum of fibroblast cells. Hydroxylation of proline and lysine is important for collagen synthesis, and it occurs with the help of various co-factors such as iron, copper, and vitamin C (Goswami et al., Citation2014). The increase in the collagen synthesis is determined by the estimation of hydroxyproline content in granulation tissue. In the present investigation, naringin treatment showed increased hydroxyproline synthesis which ensures an increase in the amount of collagen formation and deposition on the wounded site in order to provide rapid healing.
It has been documented that reactive oxygen species (ROS) plays a decisive role in an array of pathological disorders including respiratory disorders, diabetes, cancer, inflammatory disorders, and neurodegenerative diseases (Gosavi et al., Citation2011b, Citation2012b; Kamble et al., Citation2013; Patil et al., Citation2012c). ROS is also considered to be important causative factors in the aging process. Increased production of ROS with depletion in endogenous antioxidant levels resulting in elevated oxidative stress leads to tissue injury or cell death via necrosis and apoptosis (Patil et al., Citation2012a; Raygude et al., Citation2012a). SOD and GSH are two well-known antioxidants playing a major role in detoxifying free radicals and other cytotoxic chemical species (Raygude et al., Citation2012b; Visnagri et al., Citation2013), which results in the acceleration of the healing processes. Increased levels of these antioxidants accelerate vascular, inflammatory, and other phases of the healing processes by regulating vascular blood flow (Prasad et al., Citation2010). It has been reported that flavonoids, along with antioxidant or free radical scavenging activity, play a vital role in healing of wounds (Gutteridge & Halliwell, Citation1993). In the present study, treatment with naringin, a well-known flavanone glycoside, showed significant elevation in SOD and GSH levels. This elevation was achieved by rapidly donating a hydrogen atom from hydroxyl groups (at C-5 of A ring, C-40 of B ring) and carbonyl group (at C-4 of the C ring) to free radicals. The result of the present investigation is in accordance with the findings of previous research where naringin was shown to increase the endogenous antioxidant defense system via up-regulating mRNA gene expression of SOD and GSH (Kanno et al., Citation2003).
MDA indicates high oxidative stress because of the destruction of lipid membrane, particularly fatty acids of unsaturated bonds (Kandhare et al., Citation2012a; Visnagri et al., Citation2012). Inhibition of lipid peroxidation by flavonoids is supposed to increase the viability of collagen fibrils by activating DNA synthesis and preventing cell damage (Shetty et al., Citation2008). As peroxidation is also due to enzymatic activities, estimation of the cellular content of GSH is helpful in the quantification of cell formation which is not oxidized. Nitric oxide (NO) is an intracellular messenger and performs various pathological and physiological processes. It serves as an oxidant and traps ROS and protects the cell from lipid peroxidation, DNA damage, and cell death (Patil et al., Citation2012b, Citation2012e). Polymorphonuclear leukocyte (PMNL) accumulation is reflected by the elevated level of myeloperoxidase (MPO) (Kandhare et al., Citation2012d). The trend in increased PMNL infiltration was confirmed in the histological assessment of wound skin tissue from vehicle control rats. NO is an integral part of the inflammatory phase and possesses cytotoxic properties involved in regulatory mechanisms to mediate epithelialization, angiogenesis, and collagen deposition crucial to the proliferative phase (Childress & Stechmiller, Citation2002). Naringin treatment decreased MDA, NO, and MPO levels which favor inhibition of oxidative conditions and a faster rate of wound closure.
The inflammatory phase is an important phase of wound healing as pro-inflammatory cytokines such as TNF-α, IL-1β, IL-6, and IL-8 are involved in this phase. Release of this pro-inflammatory cytokines via activation of NF-kB, a transcription factor, resulted in an inflammatory response via recruitment of neutrophils and macrophages to the wound site (Kandhare et al., Citation2014a). It leads to release of ROS and reactive nitrogen species (RNS) including superoxide, hydrogen peroxide, hydroxyl radical, hypochlorous acid, nitric oxide, and peroxynitrite which contribute to the pathogenesis of wounds. It has been reported that elevated levels of TNF-α and IL-1β caused apoptosis and decreased fibroblasts proliferation resulting in delayed wound healing (Bashir et al., Citation2009). Moreover, TNF-α has potential to inhibit TGF-β and collagen gene expression in vivo (Buck et al., Citation1996). Furthermore, another cytokine, IL-8, is responsible for the activation of neutrophil chemotaxis which also in turn contributes to delay wound healing (Engelmayer et al., Citation2008). In the present study, naringin treatment suppressed the activation of NF-κB at the wound site and decreased production of pro-inflammatory cytokine mRNA levels, such as TNF-α, IL-1β, IL-6, and IL-8.
It has been reported that pol-γ reflected the activity of based excision repair (BER) enzyme. A programmed activation of DNA repair enzyme is regulated by BER involved in the repairing of oxidative neuronal DNA damage (Kandhare et al., Citation2012c, Citation2013b). Moreover, equilibrium between oxidative damage and BER regulated tissue survival. Various researchers have reported that elevated oxidative stress has been associated with reduced mtBER enzymes that lead to apoptosis (Lin et al., Citation2010). In the present investigation, pol-γ expression was decreased in the vehicle control group whereas treatment with naringin showed increased pol-γ mRNA expression indicated its antioxidative potential.
It has been documented that apoptosis plays a decisive role in the maturation of a wound via elimination of different cell populations. Apoptosis reflects a rapid switch to the proliferative phase from the inflammatory phase of healing (Kandhare et al., Citation2014a). Bcl-2-associated X protein (BAX) is a pathological index of apoptotic changes in the cell under stressful conditions and the expression of Bax has been reported to be elevated with ROS (Kandhare et al., Citation2014a). Inhibition of apoptosis is necessary for enhanced collagen I mRNA expression and matrix formation. Collagen is an extracellular protein which is an important factor necessary for normal wound healing. Elevated amounts of collagen synthesis in a wound area suggest increased strength and integrity to the tissue matrix which is necessary for the homeostasis and epithelialization at the later phase of healing. Hydroxyproline serves as the backbone of collagen synthesis. Increased hydroxyproline synthesis at the wound site is reflected by up-regulated collagen expression (Goswami et al., Citation2014). Treatment with naringin significantly inhibited the apoptosis reflected by down-regulated BAX mRNA expression thus enhanced collagen I mRNA expression via hydroxyproline synthesis and improved matrix formation to exerts its wound healing potential.
Angiogenesis played a pivotal role in the matrix formation during the wound healing process. It involved development of endothelial cells including keratinocytes, thrombocytes, neutrophils, macrophages, and fibroblasts from parent blood vessels, followed by migration, proliferation, and anastomosis to other vessels (Ganeshkumar et al., Citation2012). VEGF has been reported to have an important role in the production and development of these endothelial cells. VEGF-R1 and VEGF-R2 receptors present on endothelial cell and its activation by VEGF leads to blood vessel formation. TGF-β is another family of fibrogenic growth factors known to play an important role in collagen synthesis and scar formation via activation of macrophage migration in the wound area (Ganeshkumar et al., Citation2012). It has been reported that administration of exogenous TGF-β significantly improved the strength in experimental wounds of rats (Lawrence et al., Citation1986). Furthermore, VEGF has been reported to promote the expression of endogenous TGF-β which in turn stimulates collagen I synthesis (Zhang et al., Citation2009). However, angiogenesis is a complex, multistep process and using an agent with the ability to stimulate a single growth factor is of value only for stable angiogenesis. In the present investigation, naringin treatment showed significant up-regulation in the mRNA expression of both growth factors, i.e., VEGF and TGF-β, which could be the basis for enhanced angiogenesis and collagen deposition at the wound site.
Mothers against decapentaplegic homolog, i.e., smad family, have been reported to mediate the signal transduction of TGF-β which regulates multiple cellular processes including cell proliferation and apoptosis (Sekelsky et al., Citation1995). Activation of TGF-β caused phosphorylation of cytosolic receptor-activated Smad 3 which in turn forms heteromeric complexes with Smad 4 which translocate into nucleus and bind to DNA, and regulate the actions of TGF-β specific transcriptional responses including cell growth and proliferation, angiogenesis, apoptosis, and extracellular matrix turnover (Brunner & Blakytny, Citation2004). Whereas Smad 7, which is an inhibitory Smad protein, blocks TGF-β signal transduction, although preventing Smad 2/3 interaction with TGF-β receptors (Attisano & Wrana, Citation2000). In the present investigation, the mRNA expression of Smad 3 was down-regulated and Smad 7 expression was up-regulated at the wound site which in turn decreased TGF-β expression. However, naringin significantly restored the altered expression of smad proteins, indicating that naringin may acts through modulation of Smads to up-regulated TGF-β expression.
In conclusion, naringin exerts its wound healing potential via down-regulating expression of inflammatory (NF-κB, TNF-α, IL-1β, IL-6, and IL-8) and apoptotic (pol-γ and Bax) mediators as well as up-regulating expression of growth factors (VEGF and TGF-β), thus modulating collagen-1 gene expression to induce angiogenesis leading to wound healing ().
Figure 6. Possible molecular mechanisms through which naringin ointment may improve wound healing. In the cascade of a normal wound healing process, activation of platelets after injury causes release of pro-inflammatory cytokines like TNF-α and IL-1β that activate macrophages. Further release of TNF-α, IL-1β, and IL-6 results in inhibition of fibroblast as well as keratinocytes proliferation and migration, which delays epithelization and granulation. Inhibition of fibroblast proliferation results in decreased VEGF-c synthesis and thus in down-regulation of TGF-β1 expression. This cascade leads to decreased endothelial cell proliferation and thus to delayed neoangiogenesis and vasculogenesis. Decreased collagen synthesis results in decreased epithelization. Together, these phenomena cause delayed wound healing. Naringin ointment reduces the elevated oxidative stress and modulates the expression of growth factors (VEGF-c and TGF-β1), inflammatory mediators (TNF-α, IL-1β, and IL-6), and apoptotic mediators (pol-γ and BAX) thereby up-regulated collagen-1 expression, which improves the wound healing process (black line reflects the delayed wound healing process whereas blue lines indicate the proposed mechanism of naringin in wound healing).
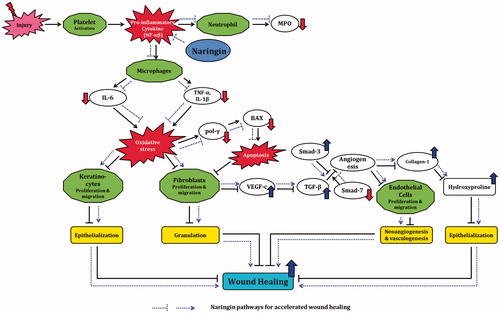
Acknowledgements
The authors would like acknowledge Prof. Dr. Shivajirao S. Kadam, Vice-Chancellor, Bharati Vidyapeeth University, Pune, India, and Prof. Dr. K. R. Mahadik, Principal, Poona College of Pharmacy, Bharati Vidyapeeth University, Pune, India, for providing necessary facilities to carry out the study.
Declaration of interest
The authors report that they have no conflicts of interest
References
- Adil M, Visnagri A, Kumar VS, et al. (2014). Protective effect of naringin on sodium arsenite induced testicular toxicity via modulation of biochemical perturbations in experimental rats. Pharmacologia 5:222–34
- Akkol EK, Koca U, Peşin I, et al. (2009). Exploring the wound healing activity of Arnebia densiflora (Nordm.) Ledeb. by in vivo models. J Ethnopharmacol 124:137–41
- Albina JE. (1994). Nutrition and wound healing. J Parenter Enteral Nutr 18:367–76
- Atiyeh BS, Ioannovich J, Al-Amm CA, El-Musa KA. (2002). Management of acute and chronic open wounds: The importance of moist environment in optimal wound healing. Curr Pharm Biotechnol 3:179–95
- Attisano L, Wrana JL. (2000). Smads as transcriptional co-modulators. Curr Opin Cell Biol 12:235–43
- Bairy K, Rao C. (2001). Wound healing profiles of Ginkgo biloba. J Nat Remedies 1:25–7
- Bashir M, Sharma M, Werth V. (2009). TNF-α production in the skin. Arch Dermatol Res 301:87–91
- Bhat RS, Shankrappa J, Shivakumar H. (2007). Formulation and evaluation of polyherbal wound treatments. Asian J Pharm Sci 2:11–17
- Bowler PG. (2002). Wound pathophysiology, infection and therapeutic options. Ann Med 34:419–27
- Brunner G, Blakytny R. (2004). Extracellular regulation of TGF-beta activity in wound repair: Growth factor latency as a sensor mechanism for injury. Thromb Haemost Stuttgart 92:253–61
- Buck M, Houglum K, Chojkier M. (1996). Tumor necrosis factor-alpha inhibits collagen alpha-1 (I) gene expression and wound healing in a murine model of cachexia. Am J Pathol 149:195--204
- Chen Y, Nie YC, Luo YL, et al. (2013). Protective effects of naringin against paraquat-induced acute lung injury and pulmonary fibrosis in mice. Food Chem Toxicol 58:133–40
- Childress BB, Stechmiller JK. (2002). Role of nitric oxide in wound healing. Biol Res Nurs 4:5–15
- Diegelmann RF, Evans MC. (2004). Wound healing: An overview of acute, fibrotic and delayed healing. Front Biosci 9:283–9
- Engelmayer J, Blezinger P, Varadhachary A. (2008). Talactoferrin stimulates wound healing with modulation of inflammation. J Surg Res 149:278–86
- Ganeshkumar M, Ponrasu T, Krithika R, et al. (2012). Topical application of Acalypha indica accelerates rat cutaneous wound healing by up-regulating the expression of Type I and III collagen. J Ethnopharmacol 142:14–22
- Ghosh P, Kandhare AD, Gauba D, et al. (2012a). Determination of efficacy, adverse drug reactions and cost effectiveness of three triple drug regimens for the treatment of H. pylori infected acid peptic disease patients. Asian Pac J Trop Dis 2:S783–9
- Ghosh P, Kandhare AD, Kumar VS, et al. (2012b). Determination of clinical outcome and pharmacoeconomics of anti-rheumatoid arthritis therapy using CDAI, EQ-5D-3L and EQ-VAS as indices of disease amelioration. Asian Pac J Trop Dis 2:S671–8
- Ghosh P, Kandhare AD, Raygude KS, et al. (2012c). Cigarette smoking and H. pylori infection: A meta-analysis of literature. Der Pharm Lett 4:128–34
- Ghosh P, Kandhare AD, Raygude KS, et al. (2012d). Determination of the long term diabetes related complications and cardiovascular events using UKPDS risk engine and UKPDS outcomes model in a representative western Indian population. Asian Pac J Trop Dis 2:S642–50
- Golinko MS, Clark S, Rennert R, et al. (2009). Wound emergencies: The importance of assessment, documentation, and early treatment using a wound electronic medical record. Ostomy Wound Manage 55:54–61
- Gopinath K, Prakash D, Sudhandiran G. (2011). Neuroprotective effect of naringin, a dietary flavonoid against 3-nitropropionic acid-induced neuronal apoptosis. Neurochem Intern 59:1066–73
- Gosain A, Dipietro LA. (2004). Aging and wound healing. World J Surg 28:321–6
- Gosavi T, Ghosh P, Kandhare A, Bodhankar S. (2011a). Unwrapping homeopathic principles in the wake of research: Serendipity, placebo or true therapeutic milestones. Pharmacologyonline 1:894–906
- Gosavi T, Kandhare A, Raygude K, et al. (2011b). A comparative study on the efficacy, safety and cost effectiveness of Viscum album and Rauwolfia serpentina mother tincture in hypertensive patients. Deccan J Nat Prod 2:29–35
- Gosavi TP, Ghosh P, Kandhare AD, et al. (2012a). Therapeutic effect of H. pylori nosode, a homeopathic preparation in healing of chronic H. pylori infected ulcers in laboratory animals. Asian Pac J Trop Dis 2:S603–11
- Gosavi TP, Kandhare AD, Ghosh P, Bodhankar SL. (2012b). Anticonvulsant activity of Argentum metallicum, a homeopathic preparation. Der Pharm Lett 4:626–37
- Goswami S, Kandhare A, Zanwar AA, et al. (2014). Oral l-glutamine administration attenuated cutaneous wound healing in Wistar rats. Int Wound J. [Epub ahead of print]. doi:10.1111/iwj.12246
- Grose R, Werner S. (2004). Wound-healing studies in transgenic and knockout mice. Mol Biotechnol 28:147–66
- Gutteridge JM, Halliwell B. (1993). Invited review free radicals in disease processes: A compilation of cause and consequence. Free Radic Res 19:141–58
- Hart J. (2002). Inflammation. 2: Its role in the healing of chronic wounds. J Wound Care 11:245–9
- Jeon SM, Bok SH, Jang MK, et al. (2001). Antioxidative activity of naringin and lovastatin in high cholesterol-fed rabbits. Life Sci 69:2855–66
- Kamble H, Kandhare AD, Bodhankar S, et al. (2013). Effect of low molecular weight galactomannans from fenugreek seeds on animal models of diabetes mellitus. Biomed Aging Pathol 3:145–51
- Kandhare AD, Bodhankar SL, Singh V, et al. (2013a). Anti-asthmatic effects of type-A procyanidine polyphenols from cinnamon bark in ovalbumin-induced airway hyperresponsiveness in laboratory animals. Biomed Aging Pathol 3:23–30
- Kandhare AD, Ghosh P, Bodhankar SL. (2014a). Naringin, a flavanone glycoside, promotes angiogenesis and inhibits endothelial apoptosis through modulation of inflammatory and growth factor expression in diabetic foot ulcer in rats. Chem-Biol Inter 219C:101–12
- Kandhare AD, Ghosh P, Ghule AE, Bodhankar SL. (2013b). Elucidation of molecular mechanism involved in neuroprotective effect of Coenzyme Q10 in alcohol induced neuropathic pain. Fund Clin Pharmacol 27:603–22
- Kandhare AD, Ghosh P, Ghule AE, et al. (2013c). Protective effect of Phyllanthus amarus by modulation of endogenous biomarkers and DNA damage in acetic acid induced ulcerative colitis: Role of phyllanthin and hypophyllanthin. Apollo Med 10:87–97
- Kandhare AD, Kumar VS, Adil M, et al. (2012a). Investigation of gastro protective activity of Xanthium strumarium L. by modulation of cellular and biochemical marker. Orient Pharm Exp Med 12:287–99
- Kandhare AD, Raygude KS, Ghosh P, et al. (2011b). Patentability of animal models: India and the globe. Int J Pharm Biol Arch 2:1024–32
- Kandhare AD, Raygude KS, Ghosh P, et al. (2012b). Neuroprotective effect of naringin by modulation of endogenous biomarkers in streptozotocin induced painful diabetic neuropathy. Fitoterapia 83:650–9
- Kandhare AD, Raygude KS, Ghosh P, et al. (2012c). Therapeutic role of curcumin in prevention of biochemical and behavioral aberration induced by alcoholic neuropathy in laboratory animals. Neurosci Lett 511:18–22
- Kandhare AD, Raygude KS, Ghosh P, et al. (2012d). Effect of hydroalcoholic extract of Hibiscus rosa sinensis Linn. leaves in experimental colitis in rats. Asian Pac J Trop Biomed 5:337–44
- Kandhare A, Raygude K, Ghosh P, Bodhankar S. (2011a). The ameliorative effect of fisetin, a bioflavonoid, on ethanol-induced and pylorus ligation-induced gastric ulcer in rats. Int J Green Pharm 5:236–43
- Kandhare AD, Raygude KS, Shiva Kumar V, et al. (2012e). Ameliorative effects quercetin against impaired motor nerve function, inflammatory mediators and apoptosis in neonatal streptozotocin-induced diabetic neuropathy in rats. Biomed Aging Pathol 2:173–86
- Kandhare AD, Shivakumar V, Rajmane A, et al. (2014b). Evaluation of the neuroprotective effect of chrysin via modulation of endogenous biomarkers in a rat model of spinal cord injury. J Nat Med 68:1–18
- Kanno S-I, Shouji A, Asou K, Ishikawa M. (2003). Effects of naringin on hydrogen peroxide-induced cytotoxicity and apoptosis in P388 cells. J Pharmacol Sci 92:166–70
- Kumar VS, Rajmane AR, Adil M, et al. (2014). Naringin ameliorates acetic acid induced colitis through modulation of endogenous oxido-nitrosative balance and DNA damage in rats. J Biomed Res 28:132–45
- Lawrence WT, Norton J, Sporn M, et al. (1986). The reversal of an adriamycin induced healing impairment with chemoattractants and growth factors. Ann Surg 203:142--7
- Lin Y, Xu J, Cao L, et al. (2010). Mitochondrial base excision repair pathway failed to respond to status epilepticus induced by pilocarpine. Neurosci Lett 474:22–5
- Lovvorn HN, Nance ML, Ferry RJ, et al. (1999). Congenital hyperinsulinism and the surgeon: Lessons learned over 35 years. J Pediatr Surg 34:786–92
- Lowry O, Rosebrough N, Farr A, Randall R. (1951). Protein measurement with the Folin phenol reagent. J Biol Chem 193:265--75
- Margaret I, Srinivasa RP, Kaiser J. (1998). Antiinflammatory profile of Tridax procumbens in animal and fibroblast cell models. Phytother Res 12:285–7
- Meyer NL, Hosier KV, Scott K, Lipscomb GH. (2003). Cefazolin versus cefazolin plus metronidazole for antibiotic prophylaxis at cesarean section. South Med J 96:992–5
- Monaco JL, Lawrence WT. (2003). Acute wound healing: An overview. Clin Plast Surg 30:1–12
- Moulin V, Auger FA, Garrel D, Germain L. (2000). Role of wound healing myofibroblasts on re-epithelialization of human skin. Burns 26:3–12
- Natarajan S, Williamson D, Stiltz AJ, Harding K. (2000). Advances in wound care and healing technology. Am J Clin Dermatol 1:269–75
- Nguyen D, Orgill D, Murphy G. (2009). The pathophysiologic basis for wound healing and cutaneous regeneration. In: Orgill DP, Blanco C, eds. Biomaterials for Treating Skin Loss. Boca Raton (FL): CRC Press, 25–57
- Nirmala S, Karthiyayini T. (2011). Wound healing activity on the leaves of Achillea millefolium L. by excision, incision, and dead space model on adult Wistar albino rats. Int Res J Pharm 2:240–5
- Opalenik SR, Davidson JM. (2005). Fibroblast differentiation of bone marrow-derived cells during wound repair. FASEB J 19:1561–3
- Panda P, Nayak S, Mohanty A, et al. (2009). Formulation and evaluation of topical dosage form of Pandanus fascicularis Lamk. and their wound healing activity. J Pharm Res 2:630–5
- Patil M, Kandhare A, Bhise S. (2011). Pharmacological evaluation of ameliorative effect of aqueous extract of Cucumis sativus L. fruit formulation on wound healing in Wistar rats. Chron Young Sci 2:207–13
- Patil M, Kandhare A, Bhise S. (2012a). Anti-inflammatory effect of Daucus carota root on experimental colitis in rats. Int J Pharm Pharm Sci 4:337–43
- Patil MVK, Kandhare AD, Bhise SD. (2012b). Anti-arthritic and anti-inflammatory activity of Xanthium srtumarium L. ethanolic extract in Freund's complete adjuvant induced arthritis. Biomed Aging Pathol 2:6–15
- Patil MVK, Kandhare AD, Bhise SD. (2012c). Effect of aqueous extract of Cucumis sativus Linn. fruit in ulcerative colitis in laboratory animals. Asian Pac J Trop Biomed 2:S962–9
- Patil MVK, Kandhare AD, Bhise SD. (2012d). Pharmacological evaluation of ethanolic extract of Daucus carota Linn root formulated cream on wound healing using excision and incision wound model. Asian Pac J Trop Biomed 2:S646–55
- Patil MVK, Kandhare AD, Ghosh P, Bhise SD. (2012e). Determination of role of GABA and nitric oxide in anticonvulsant activity of Fragaria vesca L. ethanolic extract in chemically induced epilepsy in laboratory animals. Orient Pharm Exp Med 12:255–64
- Pierce PD, Md, Glenn F, Mustoe M, Thomas A. (1995). Pharmacologic enhancement of wound healing. Ann Rev Med 46:467–81
- Prasad SK, Kumar R, Patel DK, Hemalatha S. (2010). Wound healing activity of Withania coagulans in streptozotocin-induced diabetic rats. Pharm Biol 48:1397–404
- Rashed A, Afifi F, Disi A. (2003). Simple evaluation of the wound healing activity of a crude extract of Portulaca oleracea L. (growing in Jordan) in Mus musculus JVI-1. J Ethnopharmacol 88:131–6
- Raygude KS, Kandhare AD, Ghosh P, Bodhankar SL. (2012a). Anticonvulsant effect of fisetin by modulation of endogenous biomarkers. Biomed Prev Nutr 2:215–22
- Raygude KS, Kandhare AD, Ghosh P, et al. (2012b). Evaluation of ameliorative effect of quercetin in experimental model of alcoholic neuropathy in rats. Inflammopharmacology 20:331–41
- Robson MC, Steed DL, Franz MG. (2001). Wound healing: Biologic features and approaches to maximize healing trajectories. Curr Probl Surg 38:72–140
- Ross R, Everett NB, Tyler R. (1970). Wound healing and collagen formation VI. The origin of the wound fibroblast studied in parabiosis. J Cell Biol 44:645–54
- Sarma SP, Aithal KS, Srinivasan K, et al. (1990). Anti-inflammatory and wound healing activities of the crude alcoholic extract and flavonoids of Vitex leucoxylon. Fitoterapia 61:263–5
- Sekelsky JJ, Newfeld SJ, Raftery LA, et al. (1995). Genetic characterization and cloning of mothers against dpp, a gene required for decapentaplegic function in Drosophila melanogaster. Genetics 139:1347--58
- Shetty S, Udupa S, Udupa L. (2008). Evaluation of antioxidant and wound healing effects of alcoholic and aqueous extract of Ocimum sanctum Linn in rats. Evid Based Complement Alternat Med 5:95–101
- Shirwaikar A, Shenoy R, Udupa A, et al. (2003). Wound healing property of ethanolic extract of leaves of Hyptis suaveolens with supportive role of antioxidant enzymes. Ind J Exp Biol 41:238–41
- Shivakumar V, Kandhare A, Rajmane A, et al. (2014). Estimation of the long-term cardiovascular events using ukpds risk engine in metabolic syndrome patients. Ind J Pharm Sci 76:174--8
- Singer AJ, Clark R. (1999). Cutaneous wound healing. N Engl J Med 341:738–46
- Suguna L, Singh S, Sivakumar P, et al. (2002). Influence of Terminalia chebula on dermal wound healing in rats. Phytother Res 16:227–31
- Szabowski A, Maas-Szabowski N, Andrecht S, et al. (2000). c-Jun and JunB antagonistically control cytokine-regulated mesenchymal–epidermal interaction in skin. Cell 103:745–55
- Tonnesen MG, Feng X, Clark RA. (2000). Angiogenesis in wound healing. J Investig Dermatol Symp Proc 5:40–6
- Tsuchiya T, Suzuki O, Igarashi K. (1996). Protective effects of chlorogenic acid on paraquat-induced oxidative stress in rats. Biosci Biotechnol Biochem 60:765–8
- Visnagri A, Kandhare AD, Chakravarty S, et al. (2014). Hesperidin, a flavanoglycone attenuates experimental diabetic neuropathy via modulation of cellular and biochemical marker to improve nerve functions. Pharm Biol 52:814–28
- Visnagri A, Kandhare AD, Ghosh P, Bodhankar SL. (2013). Endothelin receptor blocker bosentan inhibits hypertensive cardiac fibrosis in pressure overload-induced cardiac hypertrophy in rats. Cardiovasc Endocrinol 2:85–97
- Visnagri A, Kandhare AD, Shiva Kumar V, et al. (2012). Elucidation of ameliorative effect of Co-enzyme Q10 in streptozotocin-induced diabetic neuropathic perturbation by modulation of electrophysiological, biochemical and behavioral markers. Biomed Aging Pathol 2:157–72
- Werner S, Grose R. (2003). Regulation of wound healing by growth factors and cytokines. Physiol Rev 83:835–70
- Werner S, Krieg T, Smola H. (2007). Keratinocyte-fibroblast interactions in wound healing. J Invest Dermatol 127:998–1008
- Zhang GY, Li X, Yi CG, et al. (2009). Angiotensin II activates connective tissue growth factor and induces extracellular matrix changes involving Smad/activation and p38 mitogen-activated protein kinase signalling pathways in human dermal fibroblasts. Exp Dermatol 18:947–53