Abstract
Context: Thyme has been used in traditional medicine for medicinal purposes since ancient times.
Objective: The objective of this study was to investigate the effects of thymol and carvacrol as two major constituents of thyme on dendritic cells (DCs) maturation and T cell activation.
Materials and methods: Splenic DCs were treated with non-cytotoxic concentrations of the components and then analyzed for MHC II, CD86, and CD40 expression by flow cytometry. The effects of compounds on mitogenic, as well as allogenic T cell responses in mixed lymphocyte culture (MLR) and the release of cytokines were investigated.
Results: At 0.1 µg/ml, reduced mean fluorescent intensity (MFI) of CD86 for thymol (80.3 ± 0.2% of untreated control) and CD40 for carvacrol (79.5 ± 0.14%) was observed (p < 0.001). Decreased mitogenic T cell proliferation by thymol [proliferation index (PI) from 0.93 ± 0.11 at 1 µg/ml to 0.42 ± 0.16 at 100 µg/ml (p < 0.01)] and carvacrol [PI from 1.08 ± 0.3 at 1 µg/ml to 0.28 ± 0.1 at 100 µg/ml (p < 0.001)] was seen. Ten micrograms/ml thymol (PI, 0.85 ± 0.04) and carvacrol (PI, 0.89 ± 0.03) inhibited allogenic T cell response (p < 0.05). Decreased IFN-γ level in MLR supernatant from 1441 ± 27.7 pg/ml in untreated cells to 944 ± 32.1 at 10 µg/ml of thymol and of carvacrol (886 ± 31.7 pg/ml) (p < 0.01) was found. IL-4 levels were decreased in the presence of both compounds (p < 0.01).
Conclusion: These data showed the suppressive effects of thymol and carvacrol on DCs maturation and function, as well as T cell responses.
Introduction
Dendritic cells (DCs) have the ability to process and present antigens, induce a primary immune response and secrete various cytokines. Secreted cytokines include inflammatory cytokines such as TNF-α, IL-1β, IL-6, and IL-12 which makes DCs a good candidate for immunological modulation (Carreño et al., Citation2011; Gelao et al., Citation2014; Ebadi et al., Citation2014). According to reports, there are various plant-derived compounds that have immunomodulatory effects on murine DCs. For example, curcumin down-regulates the expression of costimulatory molecules and MHC class II antigens in stimulated DCs (Kim et al., Citation2005). This compound significantly blocks LPS-induced expression of IL-12 and inflammatory cytokines which shows its potential anti-inflammatory role for treating immune-related disorders in experimental animal models and certain clinical applications (Rogers et al., Citation2010). Polysaccharides from Ganoderma lucidum (Curtis) P. Karst (Ganodermataceae), a medicinal mushroom, have been shown to induce phenotypic and functional maturation of DCs with significant human lymphocytes proliferation and cytokine production (Meng et al., Citation2011). Sinomenine (SN), a pure compound isolated from the Sinomenium acutum (Thunb.) Rehder & E.H. Wilson (Menispermaceae) plant, decreases the function and proliferation of T and B cells in addition to interfering with differentiation, recruitment and function of DCs (Chen et al., Citation2007).
Previously, we investigated the effects of three medicinal plants from the Labiatae family – Thymus vulgaris L. (thyme), Thymus daenensis Celak, and Zataria multiflora Boiss on DCs (Amirghofran et al., Citation2012). These plants showed immunomodulatory effects on DCs maturation and/or function. T. vulgaris and Z. multiflora extracts modulated CD40 expression on DCs and suppressed allogenic T cell proliferation whereas T. daenesis had no effect on allogenic T cell responses. The effect of these extracts on IFN-γ and IL-4 cytokines secretion showed a non-significant decrease in cytokine levels. The results of this research supported the immunoinhibitory effects of the above-mentioned plants which enabled them to be candidates for further studies on their components and possible usefulness as immune-mediated disease therapies. Various studies have investigated their chemical compositions. According to the results, there exist a variety of phenolic and other chemical compounds in T. vulgaris that include carvacrol, cymol, linalool, thymol, tannin, flavonoids, saponins, borneol, and triterpenic acids (Behnia et al., Citation2008). Thymus daenensis contains thymol, carvacrol, and geraniol (Rustaiee et al., Citation2013) and the main constituents of Z. multiflora are carvacrol, zatrinal, oleanolic acid, thymol, betulic acid, rosmarinic acid, monoterpenoids, sesquiterpenoids, p-cymene, and γ-terpinene (Saei-Dehkordi et al., Citation2010). Similarities exist between the components of these plants. For example, the phenolic compounds, thymol and carvacrol, are found in all three plants. The aim of this study was to investigate the immunomodulatory effects of thymol and carvacrol on DCs maturation and T cell activation.
Materials and methods
Animals
Adult, 8 weeks old Balb/c and C57BL/6 male mice (20–22 g) were purchased from Razes Institute, Shiraz. Animals were acclimatized to standard laboratory condition (temperature, 22 ± 2 °C and lighting period of 12 h) and maintained on a standard mouse chow and water ad libitum. All protocols were approved by the ethical committee of the Shiraz University of Medical Sciences for handling of the animals.
DCs preparation
Spleens were removed from Balb/c mice and DCs prepared as described before (Bordbar et al., Citation2012). Briefly, spleens were cut into small fragments and then digested with 1 mg/ml collagenase D (Roche, Mannheim, Germany) and DNase (0.02 mg/ml, Roche) in RPMI 1640 solution (Sigma, St Louis, CA). After incubation for 30 min at 37 °C under 5% CO2, they were dissociated in RPMI medium containing 5 mM EDTA. The suspension was then passed through a stainless mesh and after washing with phosphate-buffered saline (PBS) and centrifugation, the cell pellet was resuspended in RPMI 1640 culture medium. Then DCs were separated using density gradient centrifugation on Nycodenz (Axis-Shield, Norway). Splenic DCs were washed twice with medium and then cultured in complete medium supplemented with 5% heat-inactivated fetal calf serum, l-glutamine, pyruvate, penicillin, and streptomycin (all from Gibco, Karlsruhe, Germany) in cell culture plates. After 2 h of incubation at 37 °C in a 5% CO2 atmosphere, plates were gently washed with warm culture medium and the non-adherent cells were discarded. The adherent cells were maintained in the complete culture medium in the presence or absence of the components and the incubation was continued for 16 h. After that DCs were collected and cell count was adjusted to 1 × 105/ml of culture medium and used without time delay in flow cytometry analysis. The viability of DCs isolated by this method was 90%. The purity of DC preparations was routinely greater than 90%, as assessed by flow cytometry analysis with anti-CD11c monoclonal Antibody. Collected DCs were used for various experiments in the presence of thymol and carvacrol. In all the experiments, DMSO at the highest concentration used in the test wells was used as the negative control, e.g., 0.5% in viability assay, 1% in cell proliferation assay, and 0.1% in flow cytometry.
Cell viability assay
Effects of thymol and carvacrol (Sigma, St. Louis, MO) on the viability of DCs was determined by 3-(4,5-dimethylthiazol-2-yl)-2,5-diphenyltetrazolium bromide (MTT) assay in which the yellow tetrazolium salt is metabolized by viable cells to purple formazan crystals (Karimi et al., Citation2014). Briefly, after 2 h incubation of isolated DCs and removing non-adherent cells, they were seeded in flat-bottom 96-well plates and treated with 1, 10, 20, 30, and 50 µg/ml of thymol and carvacrol for 16 h at 37 °C in a 5% CO2 atmosphere. About 10 µl of MTT (5 mg/ml, Sigma) was added to each well and the incubation was continued for an additional 4 h. After that 100 µl of dimethyl sulfoxide (DMSO, Sigma) was added to solubilize formazan crystals in the cells. Then absorbance of each well at 570 and 630 nm was determined on a microplate enzyme-linked immunosorbent assay (ELISA) reader (Pharmacia, Uppsala, Sweden). Viability percentage was calculated by the following formula: [(absorbance of treated cells/absorbance of corresponding control) × 100. Control was cells cultured without the compounds (untreated). In all experiments, DMSO as the solvent at the highest concentration used in the test wells (e.g., 0.5%) was added to the untreated (control) wells. The experiments were performed in triplicate wells and repeated at least three times.
Cell proliferation assay
In vitro cell proliferation was measured using 5-bromo-2′-deoxyuridine (BrdU) cell proliferation kit (Roche) according to the protocols of the manufacturer. Briefly, splenocytes were separated from Balb/c mouse spleen and cultivated at 1 × 105 cells/well/100 μl in flat-bottom 96-well culture plates in the presence or absence of different concentrations of the compounds. Con A (1 μg/ml, Sigma) was added to the culture of splenocytes to activate the cells. After 48 h, the cultivated cells were exposed to BrdU by adding 10 µl of BrdU solution to each well and the incubation was continued for 18 h. The medium was then removed, the cells were fixed, and DNA was denatured to improve the accessibility of the incorporated BrdU for detection by antibody. After washing, peroxidase-conjugated anti-BrdU antibody was added to the wells and the plate was incubated at room temperature for 90 min. Then substrate was added and the absorbance of the samples was measured in a microplate reader at 450 nm and 690 nm reference wavelengths. Control was Con A-stimulated cells grown in media containing DMSO (1%) without the compounds (untreated cells). The proliferation index (PI) was calculated by dividing the absorbance of treated cells to that of control.
Flow cytometry analysis
The expression of DC markers was analyzed by flow cytometry. Cells after 16 h of exposure to the compounds were transferred to flow cytometry tubes. After washing, they were incubated with 1 μl of monoclonal antibodies for 1 h at 4 °C. The antibodies used were phycoerythrin (PE)-conjugated anti CD11c, fluorescence isothiocyanate (FITC)-conjugated major histocompatibility complex (MHC) class II molecule, FITC-conjugated CD86, FITC-conjugated CD40 and FITC- and PE-conjugated isotype controls, all from Becton Dickinson (BD, San Jose, CA). After incubation, they were washed with PBS and fixed with 1% paraformaldehyde. The expression of markers was examined on a BD FACSCalibur flow cytometer using Cell Quest software (BD). Analysis of data was performed by WinMDI software (Scripps, LaHoya, CA). Forward- and side-scatter parameters were used to gate live cells and exclude dead and contaminating cells. The mean fluorescence intensity (MFI) obtained for each marker was compared with that of the untreated cells (containing 0.1% DMSO). DCs stimulated with 50 ng/ml tumor necrosis factor (TNF)-α were used as a positive control.
Allogenic response
Allogenic-mixed lymphocyte reaction (MLR) was performed in the presence or absence of the compounds as previously described (Bordbar et al., Citation2014). Briefly, DCs were isolated from Balb/c mice and after 2 h of incubation at 37 °C and 5% CO2 were treated with mitomycin (25 µg/ml, Sigma) at 37 °C for 20 min. After washing, they were co-cultured in a ratio of 1:10 with responder T cells that had been isolated from spleen of C57BL/6 mouse by a nylon wool-enrichment technique. These cells were prepared at concentration of 1 × 105 in RPMI medium supplemented with 5% human AB serum. The purity of T cells was more than 90% as determined by flow cytometry staining with anti-CD3 monoclonal antibody. About 100 µl of both DC and T cell suspensions were co-cultured in 96-well round-bottomed plates in the presence or absence of the compounds. Cells were incubated at 37 °C and 5% CO2 for 3 d and then BrdU was added into the wells. After 24 h, cell proliferation was determined by BrdU proliferation assay kit as mentioned before. Control was triplicate wells containing T cells and DCs without adding the compounds, containing DMSO (0.5%). The PI was calculated by dividing the absorbance of treated co-cultures to that of control. In a parallel experiment, the supernatant of cells were collected after 2 d and quantified for cytokine levels.
Cytokine assay
The concentration of IL-4 and IFN-γ in culture supernatants of MLR was determined using corresponding ELISA kits obtained from eBioscience (San Diego, CA) according to the instructions provided by the manufacturer. All samples were tested at least in duplicate. The sensitivity of the kits for IL-4 and IFN-γ was 2 pg/ml.
Statistical analysis
Data were presented as mean ± standard error and the differences between groups were assessed using SPSS software (SPSS Inc. Chicago, IL) and Student’s t-test and one-way ANOVA. p Values less than 0.05 were considered as significant.
Results
Effects of thymol and carvacrol on DCs viability
To determine the non-cytotoxic concentrations of thymol and carvacrol on DCs, we used the MTT assay to perform viability assays on DCs treated with different concentrations of these compounds. Both compounds did not decrease DCs viability at concentrations up to 10 µg/ml (). Therefore, we chose the 0.1, 1, and 10 µg/ml concentrations for the subsequent experiments on DCs.
Figure 1. Effects of thymol and carvacrol on the viability of dendritic cells (DCs). DCs were isolated from Balb/c mice and were cultured in the presence of various concentrations of the compounds for 16 h and then viability was assessed by MTT colorimetric assay. Negative control (C−) was untreated cells growing in media containing 0.5% DMSO as the solvent. The bars indicate mean ± standard error of at least three independent experiments. *p < 0.05, **p < 0.01, and ***p < 0.01 show significant differences with negative control.
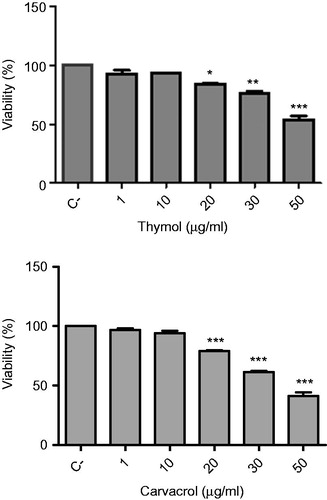
Effects of thymol and carvacrol on lymphocyte proliferation
We investigated the general effects of thymol and carvacrol on lymphocytes using Con A activated-mouse spleen cells. Both compounds decreased lymphocyte proliferation. As shown in , thymol dose dependently reduced the PI from 0.93 ± 0.11 at 1 µg/ml to 0.42 ± 0.16 at 100 µg/ml (p < 0.01). At 50 µg/ml, carvacrol reduced the PI to 0.41 ± 0.09 and at 100 µg/ml this compound decreased the PI to 0.28 ± 0.1 (p < 0.001).
Figure 2. Effects of thymol and carvacrol on lymphocyte proliferation. Mice spleen cells were stimulated with concanavalin (Con) A and then treated with different concentrations of the compounds for 48 h. Cell proliferation was measured by BrdU cell proliferation assay. Control was untreated Con A-stimulated cells growing in media containing the solvent (DMSO) [at the highest concentration used in the test wells (e.g., 1%)]. The proliferation index (PI) was calculated by dividing the optical density (OD) of treated cells to OD of control. Data represent mean ± standard error of PI resulted from three independent experiments each in triplicate. *p < 0.05, **p < 0.01, and ***p < 0.001 show significant results.
![Figure 2. Effects of thymol and carvacrol on lymphocyte proliferation. Mice spleen cells were stimulated with concanavalin (Con) A and then treated with different concentrations of the compounds for 48 h. Cell proliferation was measured by BrdU cell proliferation assay. Control was untreated Con A-stimulated cells growing in media containing the solvent (DMSO) [at the highest concentration used in the test wells (e.g., 1%)]. The proliferation index (PI) was calculated by dividing the optical density (OD) of treated cells to OD of control. Data represent mean ± standard error of PI resulted from three independent experiments each in triplicate. *p < 0.05, **p < 0.01, and ***p < 0.001 show significant results.](/cms/asset/91bfc6cc-d04b-4f03-90ef-cb072bf75354/iphb_a_1055579_f0002_b.jpg)
Effects of thymol and carvacrol on DCs phenotype
We examined various concentrations of thymol and carvacrol for their effects on DCs phenotype. DCs were isolated from the spleen of Balb/c mice. Following exposure to thymol and carvacrol, we used flow cytometry to analyze these cells for expression of MHC II, CD86, and CD40. Both DCs treated with TNF-α and untreated DCs were used as controls. Stimulation of DCs with TNF-α induced a mature DC phenotype characterized by significant up-regulation of MHC II, CD86, and CD40 expression compared with the negative control (p < 0.05, ). With respect to thymol, this compound mildly decreased the MFI of CD86 at 0.1 µg/ml (80.7 ± 0.2% of the untreated control) and 10 µg/ml (80.3 ± 0.4% of the untreated control). Thymol showed no significant effect on CD40 or MHC II molecule expression. Carvacrol significantly decreased CD40 MFI at 0.1–10 µg/ml. The MFI of CD40 at 0.1 µg/ml of this compound was 79.5 ± 0.14% of the negative control (p < 0.001). We observed no significant effect on the expression of CD86 and MHC II. These data indicated that thymol and carvacrol down-regulated the expression of DCs maturation markers. shows flow cytometry histograms that represent the MFI of CD40 and CD86 molecules in DCs treated with 10 µg/ml of thymol and carvacrol.
Figure 3. Flow cytometry analysis of the expression of CD40, CD86, and MHC II molecules on dendritic cells (DCs) after exposure to different concentrations of thymol (top) and carvacrol (bottom) for 16 h. Control (negative) was untreated cells growing in media containing 0.1% DMSO. TNF-α (50 ng/ml) was used as positive control. The cells were stained with monoclonal antibodies to CD86, MHC II, CD40, and CDllc molecules and then the expression of surface markers was analyzed by flow cytometry. DCs were gated on CD11c+ cells. The mean fluorescence intensity (MFI) ± standard error of markers compared to the control is shown in each panel. *p < 0.05, **p < 0.01, and ***p < 0.001.
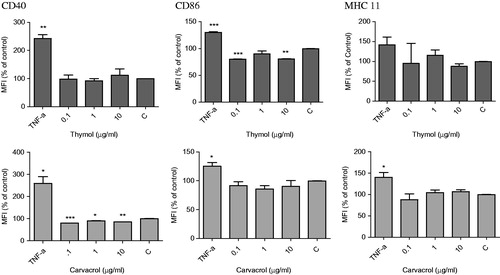
Figure 4. Histograms for the expression of CD40 and CD86 on DCs treated with 10 µg/ml thymol and carvacrol by flow cytometry as an example. Negative control was untreated cells containing 0.1% DMSO in medium and positive control was cells treated with TNF-α. The cells were stained with monoclonal antibodies to CD86, MHC II, CD40, and CDllc molecules and then the expression of surface markers was analyzed. DCs were gated on CD11c+ cells. Numbers written on M2 marker are the mean fluorescence intensity of molecules in cells treated with the compounds, TNF-α or DMSO. M1 is the relevant isotype control. The result shown is one representative experiment out of three independent experiments.
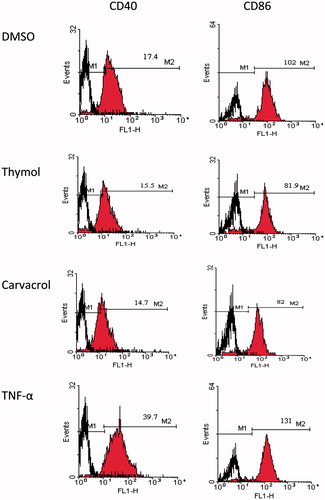
Effects of thymol and carvacrol on allogenic MLR
The effects of the compounds on the allogenic response of T cells were determined by analysis of cell proliferation. To achieve this, we co-cultured C57BL/6 mouse T cells with DCs isolated from a Balb/c mouse. As shown in , thymol significantly decreased T cell proliferation at 10 µg/ml (PI; 0.85 ± 0.04, p < 0.05). Carvacrol also inhibited T cell proliferation in allogenic MLR at the same concentration (PI; 0.89 ± 0.03, p < 0.05).
Figure 5. Effects of thymol and carvacrol on the mixed lymphocyte reaction. DCs were co-cultured with allogenic T cells at 1:10 ratio in the presence or absence of the compounds for 48 h. Negative control was untreated cells growing in media containing 0.5% DMSO. Cell proliferation was measured using BrdU assay. The PI was calculated by dividing the absorbance of treated co-cultures to that of control. The bars indicate mean ± standard error of at least three independent experiments. *p < 0.05, **p < 0.01, and ***p < 0.001 show significant differences with negative control.
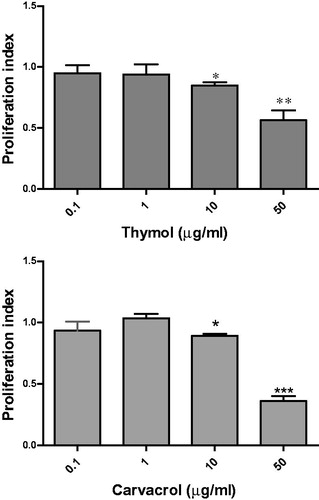
Effects of thymol and carvacrol on cytokine production
We examined for production of IFN-γ and IL-4 cytokines in the supernatant of the mixed lymphocyte culture. In this analysis, thymol decreased IFN-γ secretion compared with the control. As shown in , the level of IFN-γ at the 1 µg/ml was 837 ± 9 pg/ml and at 10 µg/ml, it was 944 ± 32.1 pg/ml – both were less than observed in untreated cells (1441 ± 27.7 pg/ml, p < 0.01). This compound also reduced IL-4 secretion at 10 µg/ml (21.5 ± 8.5 pg/ml) compared with the control (93.1 ± 6.7 pg/ml, p < 0.01).
Carvacrol also decreased IFN-γ at various concentrations that ranged from 1221 ± 8.5 pg/ml (p < 0.05) at 0.1 µg/ml to 886 ± 31.7 pg/ml (p < 0.01) at 10 µg/ml compared with the untreated control (1441 ± 27.7 pg/ml). As shown in , this compound also slightly decreased IL-4 release at 1 µg/ml (53 ± 17 pg/ml) compared with the control (93.1 ± 6.7 pg/ml; p < 0.05).
Figure 6. Cytokine production in mixed lymphocyte reaction. DCs were cultured with allogenic T cells in the presence or absence of the compounds and then IFN-γ and IL-4 cytokines were measured in the culture supernatants by enzyme-linked immunosorbent assay (ELISA) assay. Control was untreated wells containing 0.5% DMSO. The bars indicate mean ± standard error of two independent experiments. *p < 0.05 and **p < 0.01 show significant differences with negative control.
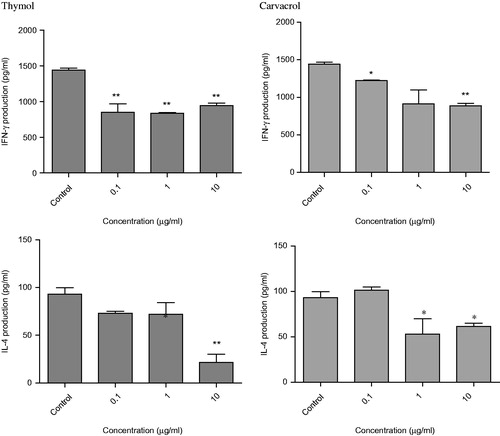
Discussion
In traditional medicine, thyme and thyme-like plants have been used for centuries to cure various diseases. The use of these plants continues as they are widely applied for their anti-septic, expectorant, anti-broncholitic, anti-spasmodic, anesthetic, carminative, and diuretic properties (Behnia et al., Citation2008; Nakhai et al., Citation2007; Zargari, Citation1990). In addition, antimicrobial, antioxidant, and anti-inflammatory activities are among their beneficial effects (Braga et al., Citation2006; Sajed et al., Citation2013; Ulukanli et al., Citation2011). Many studies have been performed to determine the chemical constituents of these plants grown in different areas of the world. Although variations exist in the type and quantity, both thymol and carvacrol are reported to be among the major components. A number of evidences show that thymol and carvacrol may possess certain abilities to modulate immune responses. The anti-inflammatory activity of thymol has been previously demonstrated by its inhibitory effect on the release of human neutrophil elastase (Braga et al., Citation2006), on cytokine responses by macrophages (Ocaña-Fuentes et al., Citation2010) and on inflammatory edema and leukocyte migration (Fachini-Queiroz et al., Citation2012). Carvacrol and thymol also reduce nitric oxide and hydrogen peroxide production in lipopolysaccharide stimulated cells (Kavoosi et al., Citation2012).
Because lymphocyte proliferation is a key event leading to the initiation and development of inflammation, the effect of thymol on the growth and activity of lymphocytes as the main cells of the immune response was investigated in our earlier study (Amirghofran et al., Citation2011). This compound inhibited proliferation of the human lymphocytes as well as the Jurkat T cell line in a concentration-dependent manner. The same result was obtained in the present study on mice lymphocytes as both thymol and carvacrol decreased mice lymphocyte proliferation, which indicated their immunosuppressive effects on lymphocytes. Given the critical role of DCs in initiation and regulation of immune responses and the presence of immunomodulatory effects of thyme and thyme-like plants on DCs (Amirghofran et al., Citation2012), we intended to study the effects of thymol and carvacrol on DCs maturation and function. In the current study, we have performed flow cytometry analyses on treated DCs for the expressions of DC maturation markers that included CD40, CD86, and MHC II co-stimulatory molecules. These molecules are involved in the interaction of DCs with naïve T cells and are important for priming T cells. DCs present antigens to T cells with the help of MHC class II molecules and stimulate them to develop an effective immune response. CD40 and CD86 provide a lower activation threshold to render T cells more sensitive to antigen stimulation (Jia et al., Citation2013). CD40 is also involved in up-regulation of other stimulatory and co-stimulatory molecules; its expression is critical for DC maturation and function. The results of our study have shown that both compounds significantly down-regulated the expression of co-stimulatory molecules. Thymol decreased CD86 expression and carvacrol decreased CD40 expression compared to the untreated control which indicated the inhibitory effects of these compounds on phenotypic maturation of DCs.
Origin, phenotype, maturation status, and the presence of cytokines are among factors that affect the function of DCs (Dalod et al., Citation2014). These cells participate in both innate and adaptive immune systems and play a central role in the initiation and regulation of immune responses (Mildner & Jung, Citation2014). We have tested the effect of thymol and carvacrol on DCs co-cultured with T cells in order to determine their impact on DCs function and T-cell responses. Both compounds at 10 µg/ml significantly reduced T cell proliferation in MLR. To determine whether thymol and carvacrol modulated the production of cytokines by T cells, we measured the levels of IFN-γ and IL-4 as the main T helper (h)1 and Th2 cytokines in the supernatant of the mixed lymphocyte culture. Our data showed that both compounds had the ability to decrease IFN-γ and IL-4 production in MLR which indicated their suppressive effects on T cell proliferation and cytokine production.
DCs have the unique ability to promote polarization of T cells toward Th1, induce IFN-γ production or Th2, and induce IL-4 secretion. In addition, they can target naive T cells to differentiate into other T cell subsets such as T regulatory (Treg) cells (Cook et al., Citation2012). It is generally believed that immature DCs are tolerogenic whereas mature DCs are immunogenic and promote immune responses (Hung et al., Citation2013). The tolerogenic features of immature DCs may be attributed to the low level of co-stimulatory molecules and an anti-inflammatory cytokine profile. Compatible with our data, in a study by Spiering et al. (Citation2012), the combination of carvacrol with a physiological form of stress led to the induction of a tolerogenic state in DCs. These cells can induce T cell anergy and Treg cells in vitro and in vivo (Kleinewietfeld & Hafler, Citation2014; Mahnke et al., Citation2007). The decreased T cell allogenic response along with down-regulation of Th1 and Th2 cytokines in MLR may show a general immunosuppressive effect of thymol and carvacrol on T cell response and the possible involvement of immunoregulatory cells. Further studies on production of various cytokines [e.g., tumor growth factor (TGF)-β] or mediators such as prostaglandin (PG) E-2, nitric oxide, and indoleamine 2,3-dioxygenase (IDO) as well as a study on Treg cells and related inhibitory molecules may clarify the mechanism underlying the inhibitory effects of the compounds on DCs function and T cell responses.
In conclusion, thymol and carvacrol showed inhibitory effects on DC maturation, and had the ability to inhibit mitogenic as well as allogenic T cell responses and secretion of IFN- γ and IL-4 cytokines. These data, collectively, have shown that the immunomodulatory effects of thyme and thyme-like plants on DCs and T cell responses might be attributed to the effects of thymol and carvacrol although the possible role of other constituents of these plants should be considered. The results obtained may partly explain the traditional use of these medicinal plants for their anti-inflammatory effects.
Acknowledgements
The present article was extracted from the thesis written by one of the authors (H. Ahmadi).
Declaration of interest
The authors declare no conflicts of interest. The study was supported by Grant no. 4927 from Shiraz University of Medical Sciences.
References
- Amirghofran Z, Ahmadi H, Karimi MH. (2012). Immunomodulatory activity of the water extract of Thymus vulgaris, Thymus daenensis, and Zataria multiflora on dendritic cells and T cells responses. J Immunoassay Immunochem 33:388–402
- Amirghofran Z, Hashemzadeh R, Javidnia K, et al. (2011). In vitro immunomodulatory effects of extracts from three plants of the Labiatae family and isolation of the active compound(s). J Immunotoxicol 8:265–73
- Behnia M, Haghighi A, Komeylizadeh H, et al. (2008). Inhibitory effects of Iranian Thymus vulgaris extracts on in vitro growth of Entamoeba histolytica. Korean J Parasitol 46:153–6
- Bordbar N, Karimi MH, Amirghofran Z. (2012). The effect of glycyrrhizin on maturation and T cell stimulating activity of dendritic cells. Cell Immunol 280:44–9
- Bordbar N, Karimi MH, Amirghofran Z. (2014). Phenotypic and functional maturation of murine dendritic cells induced by 18 alpha-and beta-glycyrrhetinic acid. Immunopharmacol Immunotoxicol 36:52–60
- Braga PC, Dal Sasso M, Culici M, et al. (2006). Anti-inflammatory activity of thymol: Inhibitory effect on the release of human neutrophil elastase. Pharmacology 77:130–6
- Carreño LJ, González PA, Bueno SM, et al. (2011). Modulation of the dendritic cell-T-cell synapse to promote pathogen immunity and prevent autoimmunity. Immunotherapy 3:6–11
- Chen Y, Yang C, Jin N, et al. (2007). Sinomenine promotes differentiation but impedes maturation and co-stimulatory molecule expression of human monocyte-derived dendritic cells. Int Immunopharmacol 7:1102–10
- Cook PC, Jones LH, Jenkins SJ, et al. (2012). Alternatively activated dendritic cells regulate CD4+ T-cell polarization in vitro and in vivo. Proc Natl Acad Sci USA 19:9977–82
- Dalod M, Chelbi R, Malissen B, et al. (2014). Dendritic cell maturation: Functional specialization through signaling specificity and transcriptional programming. EMBO J 16:1104–16
- Ebadi P, Karimi MH, Amirghofran Z. (2014). Plant components for immune modulation targeting dendritic cells: Implication for therapy. Immunotherapy 6:1037–53
- Fachini-Queiroz FC, Kummer R, Estevão-Silva CF, et al. (2012). Effects of thymol and carvacrol, constituents of Thymus vulgaris L. essential oil, on the inflammatory response. Evid Based Complement Alternat Med 2012:657026
- Gelao L, Criscitiello C, Esposito A, et al. (2014). Dendritic cell-based vaccines: Clinical applications in breast cancer. Immunotherapy 6:349–60
- Hung CY, Ysebaert D, Berneman ZN, et al. (2013). Dendritic cells: Cellular mediators for immunological tolerance. Clin Dev Immunol 2013:972865
- Jia R, Li X, Yu C, et al. (2013). The splicing factor hnRNP C regulates expression of co-stimulatory molecules CD80 and CD40 in dendritic cells. Immunol Lett 153:27–32
- Karimi MH, Ebrahimnezhad S, Namayandeh M, et al. (2014). The effects of Cichorium intybus extract on the maturation and activity of dendritic cells. Daru 24:28
- Kavoosi G, Teixeira da Silva JA, Saharkhiz MJ. (2012). Inhibitory effects of Zataria multiflora essential oil and its main components on nitric oxide and hydrogen peroxide production in lipopolysaccharide-stimulated macrophages. J Pharm Pharmacol 64:1491–500
- Kim GY, Kim KH, Lee SH, et al. (2005). Curcumin inhibits immunostimulatory function of dendritic cells: MAPKs and translocation of NF-kappa B as potential targets. J Immunol 15:8116–24
- Kleinewietfeld M, Hafler DA. (2014). Regulatory T cells in autoimmune neuroinflammation. Immunol Rev 259:231–44
- Mahnke K, Johnson TS, Ring S, et al. (2007). Tolerogenic dendritic cells and regulatory T cells: A two-way relationship. J Dermatol Sci 46:159–67
- Meng J, Hu X, Shan F, et al. (2011). Analysis of maturation of murine dendritic cells (DCs) induced by purified Ganoderma lucidum polysaccharides (GLPs). Int J Biol Macromol 1:693–9
- Mildner A, Jung S. (2014). Development and function of dendritic cell subsets. Immunity 15:642–56
- Nakhai LA, Mohammadirad A, Yasa N, et al. (2007). Benefits of Zataria multiflora Boiss in experimental model of mouse inflammatory bowel disease. Evid Based Complement Alternat Med 4:43–50
- Ocaña-Fuentes A, Arranz-Gutiérrez E, Señorans FJ, et al. (2010). Supercritical fluid extraction of oregano (Origanum vulgare) essentials oils: Anti-inflammatory properties based on cytokine response on THP-1 macrophages. Food Chem Toxicol 48:1568–75
- Rogers NM, Kireta S, Coates PT. (2010). Curcumin induces maturation-arrested dendritic cells that expand regulatory T cells in vitro and in vivo. Clin Exp Immunol 162:460–73
- Rustaiee AR, Yavari A, Nazeri V, et al. (2013). Genetic diversity and chemical polymorphism of some thymus species. Chem Biodivers 10:1088–98
- Saei-Dehkordi SS, Tajik H, Moradi M, et al. (2010). Chemical composition of essential oils in Zataria multiflora Boiss. from different parts of Iran and their radical scavenging and antimicrobial activity. Food Chem Toxicol 48:1562–7
- Sajed H, Sahebkar A, Iranshahi M. (2013). Zataria multiflora Boiss. (Shirazi thyme) – An ancient condiment with modern pharmaceutical uses. J Ethnopharmacol 13:686–98
- Spiering R, van der Zee R, Wagenaar J, et al. (2012). Tolerogenic dendritic cells that inhibit autoimmune arthritis can be induced by a combination of carvacrol and thermal stress. PLoS One 7:e46336
- Ulukanli Z, Cigremis Y, Ilcim A. (2011). In vitro antimicrobial and antioxidant activity of acetone and methanol extracts from Thymus leucotrichius (Lamiaceae). Eur Rev Med Pharmacol Sci 15:649–57
- Zargari A. (1990). Medicinal Plants. Vol. 4. Tehran: Tehran University Press, 1–57