Abstract
Context: Spices and herbs are recognized sources of natural antioxidants that can protect from oxidative stress, thus play an important role in chemoprevention of liver diseases. Ginger is used worldwide primarily as a spicy condiment.
Objective: This study evaluated the ability of ginger extract (GE) to ameliorate oxidative-hepatic toxicity induced by lead acetate (PbAc) in rats.
Materials and methods: Five groups of animals were used: group I kept as control; groups II, IV, and V received PbAc (1 ppm in drinking water daily for 6 weeks, and kept for an additional 2 weeks without PbAc exposure); group III treated orally with GE (350 mg/kg body weight, 4 d per week) for 6 weeks; group IV (protective) received GE for 2 weeks before and simultaneously with PbAc; and group V (treatment) received GE for 2 weeks after PbAc exposure.
Results: GC-MS analysis of GE revealed its content of gingerol (7.09%), quercetin (3.20%), dl-limonene (0.96%), and zingiberene (0.18%). Treatment of PbAc-treated rats with GE has no effect on hepatic Pb concentrations. However, it maintained serum aspartate aminotransferase level, increased hepatic glutathione (157%), glutathione S-transferase (GST) (228%), glutathione peroxidase (GPx) (138%) and catalase (CAT) (112%) levels, and reduced hepatic malondialdehyde (80%). Co-treatment of PbAc group with GE upregulated mRNA expression of antioxidant genes: GST-α1 (1.4-fold), GPx1 (1.8-fold), and CAT (8-fold), while post-treatment with GE upregulated only mRNA expression of GPx1 (1.5-fold).
Conclusion: GE has an antioxidant protective efficacy against PbAc-induced hepatotoxicity, which appears more effective than its therapeutic application. However, the changes in antioxidant gene expression were not reflected at the protein level.
Introduction
Lead (Pb) is one of the most abundant heavy metal pollutants in the environment, and is well documented as a dangerous and insidious poison (White et al., Citation2007). Continuous environmental and/or occupational exposure to Pb may contribute to renal, nervous, hepatic, hematologic, immunologic, and reproductive disorders in humans and animals (Flora et al., Citation2012). Pb-induced oxidative stress or disruption of the pro-oxidant/antioxidant balance in blood and soft tissues is postulated to be the major mechanism of various tissue injuries (Ashry et al., Citation2010; Flora et al., Citation2003). Chronic exposure to Pb results in its accumulation, mainly in the liver, kidneys, and brain, as well as in other organs and tissues, where it causes many metabolic and histological changes, membrane damage, altered gene expression, and apoptosis (Kojima et al., Citation2002; Quintanilla-Vega et al., Citation2000; Sobin et al., Citation2013; Wang et al., Citation2013b). It causes oxidative stress by inducing the generation of reactive oxygen species (ROS), increasing the levels of lipid peroxidation (LPO) and thiobarbituric acid-reactive substances (TBARs), and inhibiting the activity of many antioxidant biomolecules (Alcaraz-Contreras et al., Citation2011; Ashry et al., Citation2010). The biochemical and molecular toxicodynamics of Pb have yet to be fully clarified; there are some studies that note indirect mechanisms of its genotoxicity such as the production of free radicals, and provide measurements of chromosomal aberrations, DNA adducts, DNA strand breaks, and micronuclei (García-Lestón et al., Citation2010; Palus et al., Citation2003).
The use of medicinal plants is not merely a custom of the distant past. The use of herbal remedies is more prevalent in patients with chronic diseases (Egede et al., Citation2002). Spices and herbs are recognized as sources of natural antioxidants that can protect from oxidative stress and thus play an important role in chemoprevention of diseases that have their etiology and pathophysiology in ROS (Ashry et al., Citation2010; El-Ashmawy et al., Citation2005; El-Neweshy et al., Citation2013; El-Sayed et al., Citation2015). Ginger [Zingiber officinale L., (Zingiberaceae)] has been largely used worldwide as a spicy condiment (Grant & Lutz, Citation2000). Its roots and their extracts contain 6-gingerol and its derivatives, which are active phenolic compounds with high antioxidant activities both in vivo and in vitro that could protect against free radical damage as effectively as ascorbic acid (Kim et al., Citation2007). 6-Gingerol has strong anti-inflammatory, anticancer, antithrombotic, and anti-apoptotic properties (Habib et al., Citation2008; Kim et al., Citation2007; Thomson et al., Citation2002; Young et al., Citation2005). A reliable quantification of gene expression with high precision and a large dynamic range is important for measuring immediate molecular responses (Mocellin et al., Citation2003), and has become the method of choice for quantitative gene expression measurements (Stahlberg et al., Citation2005). In view of the above, this study was undertaken to assess biochemical and molecular functional alterations in the liver resulting from subchronic Pb toxicity through the estimation of oxidative stress status and the expression of antioxidant enzyme-related genes, as well as the evaluation of the possible protective and therapeutic effects of ginger extract (GE).
Materials and methods
Chemicals and assay kits
Lead acetate (PbAc; C4H6O4PbċH2O; MW 379.33) in the form of pure crystal was obtained from Riedel-De Haën, AG, Seelze, Hannover, Germany. The assay kits used for the biochemical measurements of alanine aminotransferase (ALT), aspartate aminotransferase (AST), reduced glutathione (GSH), glutathione S-transferase (GST), glutathione peroxidase (GPx), catalase (CAT), superoxide dismutase (SOD), and lipid peroxide (malondialdehyde, MDA) were purchased from Biodiagnostic or Biotechnology Co. (Cairo, Egypt). Complete gene expression assay kits for RNA extraction, GoScript™ Reverse Transcription System for cDNA synthesis, and SYBR® Green qPCR Master Mix were obtained from Promega (Madison, WI). All other chemicals were of reagent grade and were commercially available from local scientific distributors in Egypt.
Plant material and extraction
Dried roots of Z. officinale (ginger) were obtained, May 2013, from a local Herbs and Medicinal Plants market (Alexandria, Egypt). The plant was authenticated by Prof. Dr. Selim Heneidy and Prof. Dr. Azza Shehata – Botany Department, Faculty of Science, Alexandria University, Alexandria, Egypt. An aqueous extract was prepared by soaking 100 g of the dry ground ginger root in 500 ml of distilled water at 40–50 °C with daily shaking for 5 d; then, the extract was stored at 4 °C. The infusions were filtered through a double-layered gauze, the filtrate was centrifuged at 3000 rpm for 10 min, and the water was evaporated in a hot air oven at 50 °C. The aqueous extracts were formulated as suspensions 20% (w/v) in distilled water containing few drops of Tween® 80 (Shalaby & Hamowieh, Citation2010).
Gas chromatography–mass spectrometry (GC-MS) analysis
The chemical composition of water extract of ginger roots was performed using Trace™ GC Ultra-ISQ mass spectrometer (Thermo-Scientific, Waltham, MA) with a direct capillary column TG–5MS (30 m × 0.25 mm × 0.25 µm film thickness). The column oven temperature was initially held at 40 °C, and then increased by 5 °C/min to 280 °C. The injector and detector (MS transfer line) temperatures were kept at 250 °C. Helium was used as a carrier gas at a constant flow rate of 1 ml/min. Extract derivatization was done using BSTFA/TMCS (80:20, v:v) for 1 h at 70 °C, after evaporation to dryness of dichloromethane/methanol mixture. The resulting solution was dried and then dissolved in hexane. The solvent delay was 2 min and diluted samples of 1 µl were injected automatically using Autosampler AS3000 (Thermo-Scientific, Waltham, MA) coupled with GC in the splitless mode. EI mass spectra were collected at 70 eV ionization voltages over the range of m/z 50–650 in full scan mode. The ion source and quadrupole temperatures were set at 200 °C and 150 °C, respectively. The components were identified by comparison of their retention times (Rt) and mass spectra with those of WILEY 09 (Flavor & Fragrance Natural & Synthetic Compounds) and NIST 11 (National Institute of Standards and Technology, Gaithersburg, MD) Mass Spectral databases.
Animals and treatments
Eight-week-old male albino rats weighing approximately 100–120 g were used for this study. The rats were housed in a room at a controlled temperature (23 ± 1 °C) and humidity (55 ± 5%), with a 12 h dark/light cycle and ad libitum access to food and water. All rats were housed in metal box cages for 1 week prior to the experiment for acclimatization and to ensure normal growth and behavior. After the acclimatization period, the rats were divided into five groups (24 rats per group), as follows:
Group I, normal control animals; Group II, (PbAc group) animals received PbAc (1 ppm) daily for 6 weeks in their drinking water, and were subsequently kept for a further 2 weeks without PbAc exposure; Group III, (GE group) animals were treated orally with GE (350 mg/kg bwt, 4 d weekly) for 6 weeks; Group IV, (protective group) animals were pretreated orally with GE, as in Group III for 2 weeks before and during PbAc exposure for another 6 weeks; and Group V, (treatment group) animals received PbAc as in Group II followed by withdrawal of PbAc and treatment with GE for a further 2 weeks.
Six rats were randomly selected from each group at the 2nd, 4th, 6th, and 8th weeks of the experiment. Blood samples were collected from these rats and centrifuged at 5000 rpm for 10 min, and the resulting serum samples were stored at −20 °C until the biochemical parameters were assessed. The rats were then euthanized by decapitation, and their livers were collected to assess tissue Pb concentration, oxidative status, and antioxidant indices. A portion of the liver samples collected at the 6th and 8th weeks, which used immediately to assess the expression of antioxidant enzymes-related genes. The animal experiments were performed in accordance with the institutional and national guidelines for the care and use of laboratory animals, and were approved by local ethics committee (Ethical Issue: #VM1522/2010). All efforts were made to minimize the number of rats used and their suffering.
Lead concentration
At the 2nd, 6th, and 8th weeks of the experiment, the Pb concentrations in the liver tissue samples were measured by atomic absorption spectrophotometry according to the method described by Jorhem and Engman (Citation2000). Calibration curves were constructed by adding known amounts of a Pb standard (E. Merck, Darmstadt, Germany). Diluted samples of the digested tissue were injected into the atomic absorption spectrophotometer (Perkin-Elmer Model 400, Shelton, CT), and hollow cathode lamps of Pb were used at a wavelength of 283.3 nm. The liver Pb content was expressed as ppm.
Serum biochemical parameters
The hepatic function biomarkers, serum ALT, and AST enzyme activities were measured spectrophotometrically according to instructions of the manufacturer.
Estimation of tissue antioxidants and lipoperoxidation markers
To estimate their antioxidant status, liver samples were homogenized individually to make 10% homogenates and to obtain aliquots for the spectrophotometric estimation of tissue GSH levels and GST, GPx, CAT, and SOD enzymes activities. GSH levels were assayed using a method based on the reductive cleavage of 5,5′-dithiobis-(2-nitrobenzoic acid) by a sulfhydryl (–SH) group, yielding a yellow color (Beutler et al., Citation1963). The amount of the reduced chromogen (absorbance measured at 405 nm) is directly proportional to the GSH concentration. GST activity was assayed by measuring the conjugation of 1-chloro-2,4-dinitrobenzene to GSH (Habig et al., Citation1974). CAT activity was measured according to the method described by Aebi (Citation1984). CAT reacts with a known quantity of H2O2, and the reaction was halted after 1 min with a CAT inhibitor. In the presence of peroxidase, the remaining H2O2 reacts with 3,5-dichloro-2-hydroxybenzene sulfonic acid and 4-aminophenazone to form a chromophore, and the color intensity of this reaction is inversely proportional to the amount of CAT in the sample. SOD activity was assayed using the method described by Nishikimi et al. (Citation1972), which relies on the ability of the enzyme to inhibit the phenazine-methosulfate-mediated reduction of nitroblue tetrazolium dye. SOD activity was measured by detecting the change in absorbance at 560 nm over 5 min. The activity of the GPx enzyme in the tissue was determined as an indirect measure of oxidized glutathione (GSSG) that is produced upon the reduction of organic peroxide by GPx (Paglia & Valentine, Citation1967).
Lipid peroxide was measured spectrophotometrically as the concentration of malondialdehyde (MDA) after the reaction with thiobarbituric acid in acidic medium at a temperature of 95 °C for 30 min to form TBARs (Placer et al., Citation1966). The absorbance of the resulting pink product was measured at 534 nm.
Reverse transcription–quantitative PCR (RT-qPCR)
The total RNA was isolated from 30 mg of fresh liver tissue using an RNA extraction kit, following the protocol of the manufacturer. The quality of the extracted RNA was confirmed with 2% agarose electrophoresis. Single-stranded cDNA was synthesized from 2 μg of total RNA using an oligo(dT) primer and M-MLV Reverse Transcriptase (GoScript™ Reverse Transcription System, Promega, Madison, WI), according to the instructions of the manufacturer. The cDNAs were used as the template for RT-qPCR using QPCR SYBR® Green Master Mix and specific primers for the indicated antioxidant enzymes-related genes: GST-α1, GPx1, CAT, and SOD2 (Table S1). The expression values of the target genes were normalized to those of the housekeeping gene, β-actin. Amplification and the detection of SYBR® Green were performed with a real-time Mx3005P detection system (Agilent Technologies, Santa Clara, CA). RT-qPCR data were obtained as a threshold cycle (Ct) value, and were used to calculate ΔCt values for each sample. The fold change for the relative gene expression to the control was calculated by the 2−ΔΔCt method (Livak & Schmittgen, Citation2001). For the control group, ΔΔCt equals zero and 20 equals to one, so that the fold change in gene expression relative to the control equals one, by definition. For the treated groups, assessment of 2−ΔΔCt determined the fold change in gene expression relative to the control. All samples were performed and analyzed in triplicate.
Statistical analysis
All data are expressed as the mean ± standard deviations (SDs), and the levels of significance are cited. SPSS statistical package version 17.0 for Windows (IBM, Armonk, NY) was used for all data analyses. Differences in values were analyzed by one-way analysis of variance, followed by Duncan’s multiple range tests. Differences were deemed significant when p < 0.05.
Results
Chemical composition of GE
The GC-MS analysis of GE revealed that it contains phenolics, alkaloids, flavonoids, tannins, anthraquinones, terpenoid, and steroids. The identified bioactive components of GE are listed in , with their respective RT and percent composition (area %), where the most important substances are gingerol, quercetin, dl-limonene, ar-curcumene, zingiberene, β-sesquiphellandrene, linalool, pyrazine, and 1,8-terpin hydrate. The major identified compound was the gingerol (7.09%), while from total ion chromatogram (TIC), we note that the flavonoid quercetin has been appeared at multiple RTs with total percent composition of 3.2%. The chromatogram of the number of peaks of the compounds detected is shown in Figure S1.
Table 1. Bioactive components of aqueous extract of ginger roots as determined by using gas chromatography–mass spectrometry (GC-MS).
Lead concentration
As shown in , the Pb concentration was significantly (p < 0.05) increased in the livers of PbAc-treated animals at the 2nd and 6th weeks of exposure compared with the control animals. The Pb concentration was decreased by equivalent levels at the 8th week (2 weeks after withdrawal of Pb with or without GE treatment), but remained significantly higher than that of the control group. In addition, these data show that GE supplementation had no observable effect on the Pb concentration in the liver.
Table 2. The protective and therapeutic effects of ginger extract (350 mg/kg bwt orally 4 d per week) on lead concentrations in the livers (ppm, µg/g fresh weight) of rats exposed to Pb (1 ppm) for six consecutive weeks in drinking water.
Liver function biomarkers
In relation to the control group, the PbAc-treated animals exhibited increased serum AST enzyme activity at the 2nd and 4th weeks of exposure; these increased activities were observed to fall within the normal range after pre- and post-treatment with GE. Conversely, AST levels were significantly (p < 0.05) decreased at the 6th week of exposure in PbAc-treated animals, even after Pb withdrawal, and GE supplementation of these rats failed to sustain or restore AST activity to that of controls. There was no significant effect of PbAc and/or GE treatment on serum ALT enzyme activity throughout the entire experimental period ().
Table 3. The protective and therapeutic effects of ginger extract (350 mg/kg bwt orally 4d per week) on serum AST and ALT activities and hepatic lipid peroxidation byproducts in rats exposed to Pb (1 ppm) for six consecutive weeks in drinking water.
Liver tissue lipid peroxidation
As shown in , the hepatic level of MDA, a bio-indicator of LPO, was significantly increased (p < 0.05) in rats exposed to PbAc compared with control rats. Conversely, it was significantly (p < 0.05) diminished in the GE-treated group at the 2nd week and was comparable to controls at the 4th and 6th weeks of exposure. PbAc withdrawal, with or without GE treatment, gradually restored MDA concentrations to levels similar to those of controls.
Liver tissue antioxidants
GSH levels
Hepatic GSH levels were significantly increased in the PbAc-intoxicated group at the 2nd week of exposure and then decreased towards the 6th week. Conversely, GSH levels were significantly improved at the 6th week of exposure in the protective group compared with the control group. After PbAc withdrawal, GSH levels were similar to that of the control group (), with or without GE treatment (Groups II and V).
Table 4. The protective and therapeutic effects of ginger extract (350 mg/kg bwt orally 4 d per week) on hepatic non-enzymatic and enzymatic antioxidant molecules in rats exposed to Pb (1 ppm) for six consecutive weeks in drinking water.
GST activity
GST activity was significantly (p < 0.05) enhanced in the liver tissues of PbAc-treated rats and in rats that were pretreated with GE, particularly at the 4th and 6th weeks of exposure. After PbAc withdrawal, GST activity in the livers of these animals was decreased with or without GE treatment (Groups II and V) but was still comparable with that of PbAc-exposed rats ().
GPx activity
There were no obvious effects on hepatic GPx activity after PbAc or GE treatment throughout the experimental period, but it was slightly decreased in a time-dependent manner in the PbAc-treated group and a tendency to increase upon pre-treatment with GE compared with the control group ().
CAT activity
Compared with the control group, CAT enzyme activity was reduced in the liver tissues of the PbAc-treated group, with a significant (p < 0.05) reduction in the 6th week of exposure. GE co-treatment maintained CAT activity within normal values in the protective group. In addition, after PbAc withdrawal (particularly with GE treatment), CAT activity returned to that of controls ().
SOD activity
As shown in , SOD enzyme activity in the liver tissue was not affected by PbAc and/or GE treatment throughout the entire period of the experiment.
Quantitative analysis of hepatic mRNA expression
To clarify the observed changes in the activities of antioxidant enzymes, their relative gene expression profiles in the liver were examined by RT-qPCR. As shown in , exposure to PbAc for 6 weeks downregulated the relative mRNA expression of antioxidant enzymes-related genes; GST-α1, GPx1, CAT, and SOD2 that were upregulated 2 weeks after PbAc withdrawal (at 8th week). Similarly, GE supplementation downregulated the relative mRNA expression of target genes except GPx1 that was upregulated. Pretreatment of PbAc-treated group with GE (the protective group) induced upregulation of relative mRNA expression of GST-α1, GPx1, and CAT antioxidant genes. However, treatment of the PbAc-intoxicated group with GE (Treatment group) for 2 weeks after PbAc withdrawal (8th week) only upregulated the relative mRNA expression of GPx1 gene, and did not cause any changes in other downregulated antioxidants gene transcripts. As from and , the changes in the antioxidant enzymes activity and relative expression of their genes are not usually in a positive correlation.
Figure 1. Relative mRNA expression of the hepatic antioxidant enzymes-related genes GST-α1, GPx1, CAT, and SOD2 in rats exposed to PbAc and/or GE for six consecutive weeks and after PbAc withdrawal with or without GE for a further 2 weeks. The mRNA transcript levels are normalized relative to that of β-actin, housekeeping gene and represented as fold induction over control. No threshold cycle (Ct) was detected for CAT expression in the treatment group at the 8th week of exposure. Triplicate samples were analyzed to obtain an average concentration for each treatment.
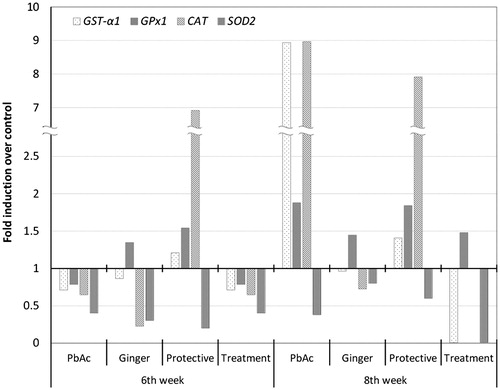
Discussion
Pb is a ubiquitous environmental and industrial pollutant that has been detected in nearly all phases of environmental and biological systems (White et al., Citation2007). Its persistence in human and animal tissues has been frequently associated with considerable health risks (El-Neweshy & El-Sayed, 2011; El-Sayed & El-Neweshy, Citation2010; Pareja-Carrera et al., Citation2014). It is believed that dietary plants can protect tissues against the damaging effect of oxidative stress (Ashry et al., Citation2010; El-Ashmawy et al., Citation2005; El-Neweshy et al., Citation2013; El-Sayed et al., Citation2015). Our results revealed that the tissue concentrations of Pb correlated with the duration of exposure. Under continuous exposure, there is a tendency for the total internal body concentrations to increase while the animal is exposed to the metal, as Pb accumulates and is not degradable. Several studies have reported an initial dramatic increase in Pb, which then decrease even though the animals are constantly exposed to the Pb (Descamps et al., Citation1996; Lagisz et al., Citation2005). Pb levels were reduced by the same amount after PbAc withdrawal with or without GE treatment, suggesting that GE does not exhibit metal-chelating ability and does not provide hepatoprotection against Pb accumulation. Additionally, the markers of liver function remained persistently high, particularly AST, at early exposure periods in rats treated with PbAc, which may be indicative of hepatic damage (Omobowale et al., Citation2014). GE initially improved transaminases activities (Farag et al., Citation2010) but failed to restore their activity to normal towards the end of the exposure period. Thus, GE could protect against the early Pb-induced hepatotoxicity.
Pb cause oxidative damage in the liver by enhancing LPO, which inactivates cell constituents during radical chain reactions that lead to the loss of membrane integrity (Liu et al., Citation2010; Patra et al., Citation2001). The significant increase in MDA suggests that ROS are associated with Pb metabolism, leading to the peroxidation of membrane lipids (Haleagrahara et al., Citation2010b). This indirectly causes ROS to accumulate in hepatic tissues, thereby increasing oxidation. Increased LPO could also be attributed to Pb-induced disturbances of free radical scavenging molecules, such as GSH, GST, GPx, CAT, and SOD (Ashry et al., Citation2010; Liu et al., Citation2010), which protecting the cell against oxidative damage. The GSH redox cycle consists of GSH, GPx, GR, and GST, which are the major components of the antioxidant defense system. GSH plays a role in the detoxification of a variety of electrophilic compounds and peroxides via catalysis by GST and GPx (Chatterjee, Citation2013). In this context, increased tissue GSH following PbAc exposure at the 2nd week and decreasing towards the end of the exposure period were observed. The increased GSH may be due to the binding of Pb to GSH to overcome the toxicity. Additionally, most of the radicals generated by metal-mediated reactions can be neutralized by GSH, which acts as the first line of cellular defense against metals by chelating and detoxifying them (Sies, Citation1999), which is consistent with the greater accumulation of Pb in the liver tissues. However, the decreased levels of tissue GSH at the 6th week may be because Pb has been shown to alter antioxidant activities by inhibiting functional –SH groups in several enzymes and antioxidant molecules, including GSH in exposed organisms (Patrick, Citation2006), rendering them non-functional and thus further impairing oxidative balance (Ashry et al., Citation2010). After exposure, Pb withdrawal resulted in normalized hepatic GSH concentrations, which supports our suggestion that GE has no direct effect on Pb toxicity.
Furthermore, there was an increase in the GST at the 4th and 6th weeks, and a reduction in GPx and CAT at the 6th week in the PbAc-treated group. Alghazal et al. (Citation2008) reported an increase in GST following exposure to inorganic Pb. Consequently, increased levels of GST and GSH, the substrate for GST, may result from Pb-generated injuries. These protective adaptation reactions protect cells from the damage caused by oxidative stress. The observation regarding GPx is consistent with the research of Jackie et al. (Citation2011), who found that GPx was reduced in the liver of rats after the oral administration of PbAc. GPx is a selenium-containing metalloenzyme that is partially located within cellular membranes and can remove H2O2 by converting GSH into GSSG (Cohen, Citation2011). Therefore, the reduced hepatic GPx following PbAc exposure may result from the involvement of this enzyme in the dismutation of peroxides generated by the Pb-induced LPO (Flora et al., Citation2003) or by the interference of Pb with some essential metals (e.g., selenium) needed for GPx activity (Wang et al., Citation2013a). The reduced hepatic CAT in the PbAc-treated rats observed in this study is consistent with the results of previous studies (Liu et al., Citation2010, Citation2012). The reduced CAT, which is predominantly located in cellular peroxisomes (Davies, Citation2000), indicates that it was overwhelmed by the ROS generated by subchronic PbAc exposure. The activity of antioxidant enzymes is exhausted by excessive ROS, which is utilized in response to ROS during the conversion of H2O2 to water (Davies, Citation2000). In contrast to the observations presented here, a reduction in hepatic SOD was reported after chronic PbAc exposure in rats (Jackie et al., Citation2011; Sujatha et al., Citation2011), which is not considered as a discrepancy point because of the compensatory mechanism between antioxidant molecules.
The antioxidant properties of ginger and its components have been explored in various in vitro (Habsah et al., Citation2000; Miean & Mohamed, Citation2001) and in vivo model systems (El-Sharaky et al., Citation2009). Herein, GE displays antioxidant activity against Pb-induced oxidative stress, as evidenced by improved changes in hepatic MDA, GSH, GST, GPx, and CAT following supplementation in PbAc-intoxicated rats. These could be attributed to its antioxidants constituents of gingerol and zingiberene, as well as dl-limonene, curcumene, linalool, β-sesquiphellandrene, and quercetin (Miean & Mohamed, Citation2001; Zachariah, Citation2008; Zancan et al., Citation2002). Haleagrahara et al. (Citation2010a) indicated that ginger has powerful antioxidant activity against Pb-induced oxidative stress in rat livers. Moreover, after Pb withdrawal, particularly with GE treatment, MDA and GSH, and antioxidant enzymes returned to baseline, indicating recovery of these animals. This finding suggests that GE has constructive effects against PbAc-induced toxicity and the protective effect of GE is more pronounced than its therapeutic effect.
The RT-qPCR gene expression data showed that mRNA levels of the hepatic antioxidant enzymes-related genes GST-α1, GPx1, CAT, and SOD2 were downregulated after PbAc exposure. The hepatic mRNA levels of GST (Daggett et al., Citation1997), GPx, CAT, and SOD transcripts (Liu et al., Citation2010) are downregulated in rats exposed to Pb. These changes may result from oxidative DNA damage induced by Pb in the rat liver (Liu et al., Citation2012). Pb-induced ROS might also act as subcellular messengers in redox-sensitive gene-regulatory and signal-transduction pathways to alter gene expression (Allen & Tresini, Citation2000). Our results revealed that GE inhibited the mRNA expression of hepatic GST-α1, CAT, and SOD2, but stimulated the mRNA levels of hepatic GPx1. Oh et al. (Citation2012) have previously reported that GE downregulated CAT and GPx mRNA levels in mouse primary hepatic cells. The inhibitory effect of GE on the gene expression of some antioxidant enzymes-related genes may be related to a feedback mechanism, as dietary supplementation of antioxidants leads to downregulation of the antioxidant response element (ARE). In addition, when provided with external antioxidant, animals will reduce the effort expended to build their own internal defenses; the chronic consumption of exogenous antioxidants reduces the “pressure” on the ARE system to gear up its own endogenous antioxidant defense system (Todd, Citation2011). Biologically, taking antioxidants leads to homeostatic downregulation of the ARE.
In the present study, there were differences in antioxidant enzymes activity and the expression of the corresponding mRNAs, which may be due to variations in protein and mRNA turnover. In addition, the efficiency of protein translation may be increased, and once the protein is translated, it may be stabilized from degradation (Hebbar et al., Citation2005). Gene expression is often interpreted in terms of protein levels, both for peptide hormones and receptors, when both are measured; the correlations are not very strong and can be as little as 40% depending on the system (Vogel & Marcotte, Citation2012). Several processes occur between transcription and translation, and protein stability plays an important role in enzymatic activity. The half-life of different proteins can vary from minutes to days, whereas the degradation rate of mRNAs has a much smaller range (2–7 h for mammalian mRNAs). Other factors that might account for these discrepancies include a lower rate of mRNA transcription compared with protein translation in mammalian cells, because on average, two mRNAs are transcribed per hour, compared with dozens of proteins translated per mRNA per hour (Vogel & Marcotte, Citation2012). They suggested that changes in gene expression level are frequently not reflected at the protein level. Moreover, a correlation among transcripts, proteins, and their activity is generally hard to find because of the presence of various checkpoints in the transcription, translation, and the post-translational modification of proteins to form a functionally active protein. The redox status and pH of reaction mixture are also key regulators of various enzyme activities (Holcik & Sonenberg, Citation2005; Schwanhausser et al., Citation2011). Some proteins and enzymes are denatured very easily after extraction, so their activities may frequently be overlooked.
Conclusion
The increase in tissue Pb levels resulted in subchronic Pb hepatic toxicity, which in turn resulted an increase in tissue LPO, perturbation of antioxidant pathways, and downregulation of the mRNA expression levels of antioxidant enzymes-related genes. Remarkably, pre- and post-treatments of PbAc-challenged animals with GE appear to be highly effective in improving the toxic effects of Pb, without a reduction in Pb concentrations, and the protective efficacy of GE appears to be more pronounced than its therapeutic effect. The activities of the antioxidant enzymes, however, did not correlate well with the levels of expression of their genes following Pb and/or GE treatment. Further studies will be required to qualify, understand, and predict the magnitude of the correlation, which will have important implications for studying the underlying mechanisms of molecular hepatotoxicity.
Supplementary material available online
Acknowledgements
The authors wish to thank Dr. Mamoun Abd El-Kareem, Atomic and Molecular Physics Unit, Experimental Nuclear Physics Department, Nuclear Research Centre, Egyptian Atomic Energy Authority, Inshas, Cairo, Egypt, for his kind helpful for GC-MS analysis and interpretations.
Declaration of interest
The authors report no conflicts of interest. The authors alone are responsible for the content and writing of this article.
References
- Aebi H. (1984). Catalase in vitro. Methods Enzymol 105:121–6
- Alcaraz-Contreras Y, Garza-Ocanas L, Carcano-Diaz K, Ramirez-Gomez XS. (2011). Effect of glycine on lead mobilization, lead-induced oxidative stress, and hepatic toxicity in rats. J Toxicol 2011:430539. doi:10.1155/2011/430539
- Alghazal MA, Lenártová V, Holovská K, et al. (2008). Activities of antioxidant and detoxifying enzymes in rats after lead exposure. Acta Vet Brno 77:347–54
- Allen RG, Tresini M. (2000). Oxidative stress and gene regulation. Free Radic Biol Med 28:463–99
- Ashry KM, El-Sayed YS, Khamiss RM, El-Ashmawy IM. (2010). Oxidative stress and immunotoxic effects of lead and their amelioration with myrrh (Commiphora molmol) emulsion. Food Chem Toxicol 48:236–41
- Beutler E, Duron O, Kelly BM. (1963). Improved method for the determination of blood glutathione. J Lab Clin Med 61:882–8
- Chatterjee A. (2013). Reduced glutathione: A radioprotector or a modulator of DNA-repair activity? Nutrient 5:525–42.
- Cohen GN. (2011). Selenocysteine and selenoproteins. In: Cohen GN, ed. Microbial Biochemistry. 2nd ed. Netherlands: Springer, 363–71
- Daggett DA, Nuwaysir EF, Nelson SA, et al. (1997). Effects of triethyl lead administration on the expression of glutathione S-transferase isoenzymes and quinone reductase in rat kidney and liver. Toxicology 117:61–71
- Davies KJ. (2000). Oxidative stress, antioxidant defenses, and damage removal, repair, and replacement systems. IUBMB Life 50:279–89
- Descamps M, Fabre MC, Grelle C, Gerard S. (1996). Cadmium and lead kinetics during experimental contamination and decontamination of the centipede Lithobius forficatus L. Arch Environ Contam Toxicol 31:350–3
- Egede LE, Ye X, Zheng D, Silverstein MD. (2002). The prevalence and pattern of complementary and alternative medicine use in individuals with diabetes. Diabetes Care 25:324–9
- El-Ashmawy IM, El-Nahas AF, Salama OM. (2005). Protective effect of volatile oil, alcoholic and aqueous extracts of Origanum majorana on lead acetate toxicity in mice. Basic Clin Pharmacol Toxicol 97:238–43
- El-Neweshy MS, El-Maddawy ZK, El-Sayed YS. (2013). Therapeutic effects of date palm (Phoenix dactylifera L.) pollen extract on cadmium-induced testicular toxicity. Andrologia 45:369–78
- El-Sayed YS, El-Neweshy MS. (2010). Impact of lead toxicity on male rat reproduction at “hormonal and histopathological levels”. Toxicol Envirovn Chem 92:765–74
- El-Sayed YS, Lebda MA, Hassinin M, Neoman SA. (2015). Chicory (Cichorium intybus L.) root extract regulates the oxidative status and antioxidant gene transcripts in CCl4-induced hepatotoxicity. PLoS One 10:e0121549
- El-Sharaky AS, Newairy AA, Kamel MA, Eweda SM. (2009). Protective effect of ginger extract against bromobenzene-induced hepatotoxicity in male rats. Food Chem Toxicol 47:1584–90
- Farag AGA, Elhalwagy MEA, Farid HEA. (2010). Effect of ginger supplementation on developmental toxicity induced by fenitrothion insecticide and/or lead in albino rats. Pestic Biochem Physiol 97:267–74
- Flora G, Gupta D, Tiwari A. (2012). Toxicity of lead: A review with recent updates. Interdiscip Toxicol 5:47–58
- Flora SJ, Pande M, Mehta A. (2003). Beneficial effect of combined administration of some naturally occurring antioxidants (vitamins) and thiol chelators in the treatment of chronic lead intoxication. Chem Biol Interact 145:267–80
- García-Lestón J, Méndez J, Pásaro E, Laffon B. (2010). Genotoxic effects of lead: An updated review. Environ Int 36:623–36
- Grant KL, Lutz RB. (2000). Ginger. Am J Health Syst Pharm 57:945–7
- Habib SH, Makpol S, Abdul Hamid NA, et al. (2008). Ginger extract (Zingiber officinale) has anti-cancer and anti-inflammatory effects on ethionine-induced hepatoma rats. Clinics (Sao Paulo) 63:807–13
- Habig WH, Pabst MJ, Jakoby WB. (1974). Glutathione S-transferases. The first enzymatic step in mercapturic acid formation. J Biol Chem 249:7130–9
- Habsah M, Amran M, Mackeen MM, et al. (2000). Screening of Zingiberaceae extracts for antimicrobial and antioxidant activities. J Ethnopharmacol 72:403–10
- Haleagrahara N, Jackie T, Chakravarthi S, et al. (2010a). Protective effect of Etlingera elatior (torch ginger) extract on lead acetate-induced hepatotoxicity in rats. J Toxicol Sci 35:663–71
- Haleagrahara N, Jackie T, Chakravarthi S, et al. (2010b). Protective effects of Etlingera elatior extract on lead acetate-induced changes in oxidative biomarkers in bone marrow of rats. Food Chem Toxicol 48:2688–94
- Hebbar V, Shen G, Hu R, et al. (2005). Toxicogenomics of resveratrol in rat liver. Life Sci 76:2299–314
- Holcik M, Sonenberg N. (2005). Translational control in stress and apoptosis. Nat Rev Mol Cell Biol 6:318–27
- Jackie T, Haleagrahara N, Chakravarthi S. (2011). Antioxidant effects of Etlingera elatior flower extract against lead acetate-induced perturbations in free radical scavenging enzymes and lipid peroxidation in rats. BMC Res Notes 4:67
- Jorhem L, Engman J. (2000). Determination of lead, cadmium, zinc, copper, and iron in foods by atomic absorption spectrometry after microwave digestion: NMKL Collaborative Study. J AOAC Int 83:1189–203
- Kim JK, Kim Y, Na KM, et al. (2007). [6]-Gingerol prevents UVB-induced ROS production and COX-2 expression in vitro and in vivo. Free Radic Res 41:603–614
- Kojima M, Nemoto K, Murai U, et al. (2002). Altered gene expression of hepatic lanosterol 14α-demethylase (CYP51) in lead nitrate-treated rats. Arch Toxicol 76:398–403
- Lagisz M, Kramarz P, Niklinska M. (2005). Metal kinetics and respiration rates in F1 generation of carabid beetles (Pterostichus oblongopunctatus F.) originating from metal-contaminated and reference areas. Arch Environ Contam Toxicol 48:484–9
- Liu CM, Ma JQ, Sun YZ. (2012). Puerarin protects the rat liver against oxidative stress-mediated DNA damage and apoptosis induced by lead. Exp Toxicol Pathol 64:575–82
- Liu CM, Zheng YL, Lu J, et al. (2010). Quercetin protects rat liver against lead-induced oxidative stress and apoptosis. Environ Toxicol Pharmacol 29:158–66
- Livak KJ, Schmittgen TD. (2001). Analysis of relative gene expression data using real-time quantitative PCR and the 2(-Delta Delta C(T)) method. Methods 25:402–8
- Miean KH, Mohamed S. (2001). Flavonoid (myricetin, quercetin, kaempferol, luteolin, and apigenin) content of edible tropical plants. J Agric Food Chem 49:3106–12
- Mocellin S, Rossi CR, Pilati P, et al. (2003). Quantitative real-time PCR: A powerful ally in cancer research. Trends Mol Med 9:189–95
- Nishikimi M, Appaji N, Yagi K. (1972). The occurrence of superoxide anion in the reaction of reduced phenazine methosulfate and molecular oxygen. Biochem Biophys Res Commun 46:849–54
- Oh S, Lee J-h, Lee J, et al. (2012). An aqueous extract of Zingiber officinale Roscoe protects mouse primary hepatic cells against hydrogen peroxide-induced oxidative stress. Biotechnol Bioproc E 17:168–75
- Omobowale TO, Oyagbemi AA, Akinrinde AS, et al. (2014). Failure of recovery from lead induced hepatoxicity and disruption of erythrocyte antioxidant defence system in Wistar rats. Environ Toxicol Pharmacol 37:1202–211
- Paglia DE, Valentine WN. (1967). Studies on the quantitative and qualitative characterization of erythrocyte glutathione peroxidase. J Lab Clin Med 70:158–69
- Palus J, Rydzynski K, Dziubaltowska E, et al. (2003). Genotoxic effects of occupational exposure to lead and cadmium. Mutat Res Genet Toxicol Environ Mutagen 540:19–28
- Pareja-Carrera J, Mateo R, Rodríguez-Estival J. (2014). Lead (Pb) in sheep exposed to mining pollution: Implications for animal and human health. Ecotoxicol Environ Saf 108:210–16
- Patra RC, Swarup D, Dwivedi SK. (2001). Antioxidant effects of alpha tocopherol, ascorbic acid and l-methionine on lead induced oxidative stress to the liver, kidney and brain in rats. Toxicology 162:81–8
- Patrick L. (2006). Lead toxicity part II: The role of free radical damage and the use of antioxidants in the pathology and treatment of lead toxicity. Altern Med Rev 11:114–27
- Placer ZA, Cushman LL, Johnson BC. (1966). Estimation of product of lipid peroxidation (malonyl dialdehyde) in biochemical systems. Anal Biochem 16:359–64
- Quintanilla-Vega B, Hoover DJ, Bal W, et al. (2000). Lead interaction with human protamine (HP2) as a mechanism of male reproductive toxicity. Chem Res Toxicol 13:594–600
- Schwanhausser B, Busse D, Li N, et al. (2011). Global quantification of mammalian gene expression control. Nature 473:337–42
- El-Neweshy MS, El-Sayed YS. (2011). Influence of vitamin C supplementation on lead-induced histopathological alterations in male rats. Exp Toxicol Pathol 63:221–7
- Shalaby MA, Hamowieh AR. (2010). Safety and efficacy of Zingiber officinale roots on fertility of male diabetic rats. Food Chem Toxicol 48:2920–24
- Sies H. (1999). Glutathione and its role in cellular functions. Free Radic Biol Med 27:916–21
- Sobin C, Montoya MG, Parisi N, et al. (2013). Microglial disruption in young mice with early chronic lead exposure. Toxicol Lett 220:44–52
- Stahlberg A, Zoric N, Aman P, Kubista M. (2005). Quantitative real-time PCR for cancer detection: The lymphoma case. Expert Rev Mol Diagn 5:221–30
- Sujatha K, Srilatha CH, Anjaneyulu Y, Amaravathi P. (2011). Lead acetate induced nephrotoxicity in Wister albino rats. A pathological, immunohistochemical and ulterasturactural studies. Int J Pharma Bio Sci 2:B.459–69
- Thomson M, Al-Qattan KK, Al-Sawan SM, et al. (2002). The use of ginger (Zingiber officinale Rosc.) as a potential anti-inflammatory and antithrombotic agent. Prostaglandins Leukot Essent Fatty Acids 67:475–8
- Todd AC. (2011). The case against antioxidants. In: Train yourself to thrive on stress. Getting Stronger. Available from: http://gettingstronger.org/2011/03/the-case-against-antioxidants/ [last accessed 17 Mar 2015]
- Vogel C, Marcotte EM. (2012). Insights into the regulation of protein abundance from proteomic and transcriptomic analyses. Nat Rev Genet 13:227–32
- Wang J, Zhu H, Yang Z, Liu Z. (2013a). Antioxidative effects of hesperetin against lead acetate-induced oxidative stress in rats. Indian J Pharmacol 45:395–8
- Wang L, Lin S, Li Z, et al. (2013b). Protective effects of puerarin on experimental chronic lead nephrotoxicity in immature female rats. Hum Exp Toxicol 32:172–85
- White LD, Cory-Slechta DA, Gilbert ME, et al. (2007). New and evolving concepts in the neurotoxicology of lead. Toxicol Appl Pharmacol 225:1–27
- Young HY, Luo YL, Cheng HY, et al. (2005). Analgesic and anti-inflammatory activities of [6]-gingerol. J Ethnopharmacol 96:207–10
- Zachariah TJ. (2008). Ginger. In: Parthasarathy VA, Chempakam B, Zachariah TJ, eds. Chemistry of Spices. King's Linn: CABI, UK by Biddles Ltd, 70–96
- Zancan KC, Marques MOM, Petenate AJ, Meireles MAA. (2002). Extraction of ginger (Zingiber officinale Roscoe) oleoresin with CO2 and co-solvents: A study of the antioxidant action of the extracts. J Supercrit Fluids 24:57–76