Abstract
Context: Obesity is the main risk factor for type 2 diabetes mellitus. Secondary metabolites with biological activities and pharmacological potential have been identified in species of the Baccharis genus that are specifically distributed in the Americas.
Objective: This study evaluated the effects of methanol extracts from Baccharis dracunculifolia DC. Asteraceae on metabolic parameters, satiety, and growth in monosodium glutamate (MSG) induced-obesity model rats.
Materials and methods: MSG was administered to 32 newborn rats (4 mg/g of body weight) once daily for 5 consecutive days. Four experimental groups (control, control + extract, MSG, and MSG + extract) were treated for 30 consecutive days with 400 mg/kg of B. dracunculifolia extract by gavage. Biochemical parameters, antioxidant activity, total extract phenolic content (methanolic, ethanolic, and acetone extractions), and pancreatic islets were evaluated.
Results: High levels of phenolic compounds were identified in B. dracunculifolia extracts (methanol: 46.2 ± 0.4 mg GAE/L; acetate: 70.5 ± 0.5 mg GAE/L; and ethanol: 30.3 ± 0.21 mg GAE/L); high antioxidant activity was detected in B. dracunculifolia ethanol and methanol extracts. The concentration of serum insulin increased 30% in obese animals treated with extract solutions (1.4–2.0 µU/mL, p < 0.05). Insulin secretion in pancreatic islets was 8.3 mM glucose (58%, p < 0.05) and 16.7 mM (99.5%, p < 0.05) in rats in the MSG + extract and MSG groups, respectively.
Discussion and conclusion: Treatment with B. dracunculifolia extracts protected pancreatic islets and prevented the irreversible cellular damage observed in animals in obesity and diabetes models.
Introduction
The pathogenesis of diabetes mellitus is linked to pro-inflammatory cellular mechanisms, both in pancreatic β-cells and skeletal muscle cells (Akash et al., Citation2013a). Recently, anti-inflammatory and molecular therapies such as interleukin 1 receptor antagonism have been considered innovative because it reduces apoptosis in pancreatic β-cells and improves their function in insulin release (Akash et al., Citation2012). In addition, more sensitive scientific technologies (fluorescent nanosensors, electrochemical biosensors, genetic screening and profiling, and radioimmune assays) and analysis of biomarkers (IL-6, TNF-α, leptin, adiponectin, glutathione peroxidase, thioredoxin, endothelin-1, and intercellular adhesion molecule 1) are emerging with potential to combat this pathology (Akash et al., Citation2013b). In the experimental field, different studies with different models that mimic the clinical signs of diabetes (streptozotocin, alloxan, monosodium glutamate, Zucker diabetic fatty rats, and ob/ob) contribute promoting new-supporting procedures and therapies to the treatment of diabetes. Among these new approaches, the use of natural products such as propolis (Ueda et al., Citation2013), Baccharis (Lemos et al., Citation2007), caffeine (Akash et al., Citation2013c; Silva et al., Citation2014), and specific components of medicinal plants such as artelipin C, flavonoids, phenolic compounds, and coumarins (Marcucci et al., Citation2001) have been promising.
The large Baccharis (Asteraceae) genus includes approximately 350 species distributed specifically in the Americas. Several studies identified secondary metabolites with biological activities and pharmacological potential (Brown et al., Citation1994; Labbe et al., Citation1986; Zdero et al., Citation1989) in plants within this genus. Several biological properties have been described in Baccharis species including anti-rheumatic and antifungal (Rahalison et al., Citation1995), hepatoprotective (Seo et al., Citation2003) and protective action against gastric ulcers (Lemos et al., Citation2007). Baccharis dracunculifolia DC, popularly known as “alecrim-do-campo”, is largely distributed in South America from southeastern Brazil, to Argentina and Uruguay. In Brazil in particular, honeybees (Apis mellifera) utilize flower nectars of B. dracunculifolia to produce green propolis.
Other plants with antidiabetic properties must also be considered. Allium cepa L. (Amaryllidaceae) contains quercetin, an important component that induces hypoglycemia by inhibiting α-glucosidases in the intestine and increases the levels of adiponectin, a hormone in adipose tissue that can prevent the digestion of carbohydrates and reduce insulin resistance (Akash et al., Citation2014). Nigella sativa L. (Ranunculaceae) has an effect on glycemic control due to its insulinotropic and antioxidant activities and capacity to inhibit hepatic gluconeogenesis (Akash et al., Citation2011).
Monosodium glutamate (MSG) intake results in degeneration of the hypothalamus arcade nucleus and leads to obesity, growth deficit [low levels of growth hormone (GH)], and sexual and behavioral dysfunction in rodents. The hypothalamus arcade nucleus is an important region of leptin action that, when injured, causes imbalanced food ingestion leading to loss of appetite and small animals (Lemos et al., Citation2013; Morris et al., Citation1998; Voltera et al., Citation2008).
The MSG induced-obesity model produces animals that are normo- or hypophagic with accumulated visceral fat that results from sympathetic activity and limited thermogenic responses in the brown adipose tissue through decreased energy use, increased fat storage, and increased adipocyte sizes (Macho et al., Citation2000; Voltera et al., Citation2008).
The damage induced by MSG promote hypothalamic obesity with different neuroendocrine and metabolic alterations that contribute to the development of hyperinsulinemia, insulin resistance, and hyperleptinemia with decreased activity of glucose transporter 4 (GLUT-4) (Brown et al., Citation1994; Hirata et al., Citation1997).
Primary defects of obesity can promote insulin resistance, diabetes inducing β-cell dysfunction, insulin secretion, and insulin action. Therefore, this study evaluated whether chronic supplementation of B. dracunculifolia extract solution could modify satiety, lipid profile, and glucose-stimulated insulin secretion in rat pancreatic islets, and whether the administration of B. dracunculifolia methanol extract to MSG induced-obesity model rats affects metabolic parameters, satiety, and growth.
Materials and methods
Animals
Newborn male Wistar rats weighing between 7 and 8 g were used in the study and were divided into two groups of 16 animals each. One group received 4 g/kg of body weight of intradermal monosodium glutamate (MSG) injections to induce obesity (Olney, Citation1969) during the first 5 days of life. The control group received injections of an equimolar saline solution during the same first 5 days of life. Animals from both groups were weaned at 21 days of age and subsequently divided in four groups: control (normal rats treated with Tween 80 solution in distilled water); control extract (treated with B. dracunculifolia extract solution); MSG (hypothalamic obesity induced by monosodium glutamate, treated with Tween 80 solution in distilled water); and MSG extract (treated with B. dracunculifolia extract solution). These groups were treated for 30 consecutive days. The subchronic treatment with B. dracunculifolia extract solution used the dose of 400 mg/kg by gavage.
All animals were housed under controlled conditions of 12 h light–dark (7 am to 7 pm) and temperature (21 ± 2 °C). Water and standard rodent chow (Nuvital®, Curitiba/PR, Brazil) were supplied ad libitum. All experimental procedures were approved by the Animal Ethics Committee (protocol n. 016/2011) from the Paraná Midwest State University.
Botanical material
Baccharis dracunculifolia was collected in April of 2011 at the Paraná Midwest State University campus (25°32′7.24″S and 50°39′42.39″W). The plant material was identified by Prof. Dr. Paulo Vitor Farago (Ponta Grossa State University – Department of Pharmaceutical Sciences). The voucher specimen was deposited in the Herbarium from the Ponta Grossa State University and registered under number 18173.
The B. dracunculifolia methanol extract solution was prepared from naturally shade-dried leaves; dried leaves were ground and sifted. We conducted a test of residual humidity indicating the solvent concentration to be added. Leaves (4 g) were dried according to the drying method described in the Brazilian Pharmacopoeia (Citation1988); the drying step was performed in triplicate. Samples were heated at 100 °C for 3 days and reweighed. The difference between weights was considered the value of residual moisture content in the sample, and was approximately 10%.
The extraction process was performed through orbital agitation in samples of 50 g of ground leaves in 220 mL of methanol. This mixture remained in the shaker for 1 week totaling 1100 × g of plant spray. The solution was subsequently filtered, and the filtrate evaporated in a rotary evaporator at a controlled temperature, and then in a water bath (for 3 days) with controlled temperature for the complete removal of solvent (methanol). The extract was diluted in Tween 80 (vehicle) and distilled water solution in the ratio of 1/8.
Antioxidant activity
Antioxidant activities were assessed in vitro by the DPPH free radical scavenging, ABTS radical cation decolorization, and ferric reducing antioxidant power (FRAP) methods.
DPPH free radical spectrophotometric assay
The antioxidant activity was assessed by the DPPH radical scavenging capacity performed according to the methodology described by Thetsrimuang et al. (Citation2011), with adaptations and using the 1,1-diphenyl-2-picrilhidrazyl (DPPH) reagent.
Bioactive compounds were extracted with methanol, ethanol, or acetone (5 g of air-dried powered leaves and 20 mL of extraction solvent). The reaction mixture consisted of 0.5 mL of sample (extraction solution from plant or extract solution used in the biological model of obesity), 3 mL of absolute ethanol, and 0.3 mL of 0.5 mM DPPH radical solution in ethanol. The mixture was evaluated at 517 nm after 40 min of reaction time.
The mixture of ethanol (3.3 mL) and sample (0.5 mL) was used as a blank. The control solution was prepared by mixing ethanol (3.5 mL) and DPPH radical solution (0.3 mL). Tests were carried out in triplicate; the total antioxidant activity was calculated in relation to the reactivity to Trolox (a water soluble analogue of vitamin E). Results were expressed as mmol Trolox/g of sample (Trolox-equivalent antioxidant capacity – TEAC).
ABTS radical cation decolorization assay
The ABTS radical scavenging capacity test was performed according to the methodology described by Huang et al. (Citation2011) with some modifications. ABTS radical cations (ABTS•+) were generated by mixing 7 mmol/L ABTS (2,2′-azinobis-(3-ethylbenzothiazoline-6-sulfonic acid) stock solution with 2.45 mmol/L potassium persulfate solution. The mixture was kept in the dark and at room temperature for 16 h. The ABTS•+ solution was diluted with ethanol to an absorbance of 0.70 ± 0.02 at 734 nm. An aliquot (30 µL) of the sample was added to 3 mL of the diluted ABTS + solution. After 6 min, the absorbance was assessed at 734 nm. Tests were carried out in triplicate; the total antioxidant activity was calculated in relation to the reactivity to Trolox. Results were expressed as mM Trolox/g of sample (TEAC).
Ferric reducing antioxidant power
The ferric reducing antioxidant power (FRAP) assay was executed according to Benzie and Strain (Citation1996), with some modifications. The FRAP reagent solution was prepared by mixing 25 mL of 300 mM acetate buffer, 2.5 mL of 10 mM TPTZ (2, 4, 6-tripyridyl-s-triazine) solution, and 2.5 mL of 20 mM ferric chloride hexahydrate. An aliquot (90 µL) of the sample was allowed to react with 2.7 mL of the FRAP solution and 270 µL of distilled water for 30 min at 37 °C. Absorbance was read in the reaction mixture at 593 nm. The antioxidant potential of the sample was determined against a standard curve of ferrous sulfate (100, 500, 1000, 1500, and 2000 µM).
All measurements were carried out in triplicate and results were expressed as µM FeSO4·7H2O/g of sample.
Total phenolics assay
The total phenolic content was determined through the Folin–Ciocalteu assay and according to the procedure described by Singleton and Rossi (Citation1965). Absorbance was read at 750 nm (UV–VIS lambda 40, Perkin Elmer, Shelton, CT) and results were calculated using a pre-prepared gallic acid calibration curve (0–100 mg/L). All measurements were carried out in triplicates. The phenolics content was expressed as gallic acid equivalents (mg of GAE/L of plant extract). The total polyphenol content were quantified in B. dracunculifolia plant extracts (methanolic, ethanolic, and acetone extractions) and the extract solution employed in the biological model.
Characterization of obesity
Adipose tissue depots were removed immediately after euthanasia and weighed to evaluate obesity. The visceral depots evaluated were mesenteric, retroperitoneal, and epididymal fat pads. Inguinal fat content was measured as subcutaneous depots. All data were expressed in g/100 g of body weight. In addition, obesity was also evaluated by the Lee Index, calculated using body weight (g)1/3/naso-anal length (cm), and used as a predictor of obesity in rodents (Bernardis & Patterson, Citation1968).
Insulin resistance
Insulin sensitivity was evaluated using the homeostasis model assessment index (HOMA-IR) as a surrogate marker of insulin resistance. The HOMA-IR was obtained using fasting glucose (mM) × fasting insulin (ng/mL)/22.5 (Matthews et al., Citation1985).
Biochemical analysis
After 12 h of fasting, rats from all groups were anaesthetized with ketamine and xylazine (55 and 8 mg/kg body weight, respectively) and euthanized by cervical dislocation; samples of total blood were collected to measure insulin levels by radioimmunoassay (RIA) (Scott et al., Citation1981). Fasting plasma glucose levels were determined by the glucose and glucose-oxidase techniques (Trinder, Citation1969). The determinations of lipid, serum cholesterol, HDL cholesterol, LDL cholesterol, VLDL cholesterol, and triglycerides, were performed by Labtest Diagnostic SA®.
Glucose oral tolerance test
Blood was collected from the tail vein in animals that fasted for 12 h. These animals subsequently received one single dose of glucose (1 mg/kg of body weight) by gavage, and new blood samples were collected at times 15, 30, and 60 min. Blood glucose levels were determined in a glucometer (Accu-chek Advantage®).
Isolation of pancreatic islets
The isolation of pancreatic islets was performed by the collagenase technique (Lacy & Kostianovsky, Citation1967) with adaptations. Rats from all groups were anesthetized, and their abdominal wall was cut and opened. Hank’s buffered saline solution (HBSS, 10 mL) containing collagenase type V (1 mg/mL, Sigma Chemical Co., St. Louis, MO) was injected into the common bile duct. The pancreas, swollen with the collagenase solution, was quickly excised and incubated in a plastic culture bottle for 15 min at 37 °C. The suspension obtained was filtered over a 0.5 mm metal mesh and washed five times with HBSS including 0.12% bovine serum albumin fraction V (BSA). The islets were collected with the aid of a microscope. At least four rats were used to obtain a pool of pancreatic islets from each animal group.
Islet incubation
Batches of four islets were pre-incubated for 60 min in 1 mL of normal Krebs solution containing 120 mM NaCl, 4.8 mM KCl, 2.5 mM CaCl2, 1.2 mM MgCl2, 24 mM NaHCO3, and 5.6 mM glucose. This solution was gassed with O2/CO2 (95/5%) to maintain pH 7.4, supplemented with BSA (0.12%), and used in the next experimental protocols in the study (Lacy & Kostianovsky, Citation1967). The pre-incubated islets then underwent another 60 min incubation in the different glucose concentrations of 5.6, 8.3, and 16.7 mM in Krebs solution. Samples from the incubation media were taken and stored frozen for further measurement of secreted insulin by RIA.
Statistical analysis
Data were expressed as mean and standard error of mean (SEM) using the statistical program Graphpad Prism version 5.0 (Graphpad Software Inc., San Diego, CA) for Windows. Analyses were performed using analysis of variance (ANOVA) followed by the Bonferroni post-test. The acceptable level of significance was p < 0.05.
Results
Biometrical and Biochemical parameters
Animals treated with MSG showed a significant increase in the Lee index (8% higher) compared to the control animals. The Lee index for the MSG-treated animals is characteristic of the induction of obesity. Treatments with the B. dracunculifolia methanol extract solution did not promote changes in this parameter (). Moreover, the obesity model was efficient, and animals showed an increase in mesenteric fat (200%), periepididymal fat (100%), retroperitoneal fat (55%), and subcutaneous-inguinal fat (190%) compared to animals in the Control group (). Triglycerides were 62.3 and 67% higher in MSG group than the control and control extract groups, respectively.
Table 1. Effect of chronic administration of B. dracunculifolia extract in biochemical and biometric parameters in rats from the induced-obesity model.
Glucose oral tolerance test
No significant difference was observed in glycemic levels between obese rats and rats in the control group. The maximum glycemic peak occurred at 15 min in the oral glucose tolerance test; the MSG group showed a significant increase of 5.5% in the area under the glucose concentration curve when compared to the control group. Similarly, animals in the MSG Extract group showed a significant increase of 7.64% in the area under the glucose concentration curve compared to the control group, and 8.2% compared to the control extract group. Therefore, treatment with B. dracunculifolia extract solution did not attenuate the increase in glycemia in obese animals when compared to animals in the control groups ().
Hormonal alterations
The concentration of serum insulin increased significantly (30%) only in obese animals treated with the solution of extract (). A significant increase of 33% in secreted insulin was observed in animals in the MSG Extract group compared to animals in the MSG group.
Figure 2. Plasma (A) insulin, and (B) HOMAir score [insulin (μU/mL) × glycemia (mmol/L)/22.5] in the studied groups. The values are presented as mean ± EPM (n = 8). Different letters indicate statistically significant difference between groups (p < 0.05; Bonferroni test after one-way ANOVA).
![Figure 2. Plasma (A) insulin, and (B) HOMAir score [insulin (μU/mL) × glycemia (mmol/L)/22.5] in the studied groups. The values are presented as mean ± EPM (n = 8). Different letters indicate statistically significant difference between groups (p < 0.05; Bonferroni test after one-way ANOVA).](/cms/asset/23a89580-d5ed-479d-8a34-dc4094cc963b/iphb_a_1067232_f0002_b.jpg)
The results presented in showed that the MSG group secreted more insulin than the control group and indicated significant differences between the glucose concentrations of 5.6 mM (63%) and 8.3 mM (107%).
Figure 3. Glucose-induced insulin secretion (A) MSG treatment; (B) 30 days of oral administration of B. dracunculifolia extract solution; (C) MSG-obese rats treated with B. dracunculifolia extract solution. The values are presented as mean ± EPM. Symbols above bars represent statistical difference only for *p < 0.05 in the analysis of variance.
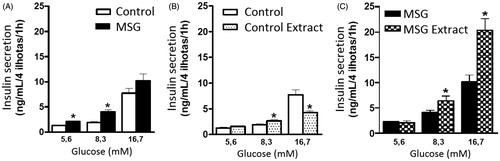
The concentration of secreted insulin increased gradually in the control extract group in the three glucose concentrations compared to the control group () with increased significant difference in the two highest concentrations (8.3 and 16.7 mM). However, the control group showed the lowest concentration of insulin secreted by the pancreatic islets compared to the control extract group in the lowest glucose concentrations (5.6 and 8.3 mM). The control group also showed the highest insulin concentration secreted by islets in 16.7 mM glucose compared to the control extract group.
The higher concentration of insulin secreted by islets in obese animals was observed in the MSG extract group compared to the MSG group (); significant differences in insulin secreted concentrations were observed between the concentrations of 8.3 mM (58%) and 16.7 mM (99.5%) of glucose.
shows a significant reduction in the pancreatic islets area in the MSG-treated group compared to the control group. Interestingly, the protective effect of the B. dracunculifolia methanol extract solution can be observed, quantified by the largest islets compared to result in the MSG group.
Figure 4. Effects of oral administration of B. dracunculifolia extract solution on the morphology of pancreatic islets from MSG-obese rats. Photomicrograph representative of pancreatic islets (left) stained with hematoxylin–eosin (H&E). Morphometric evaluations of the area with islets in all groups were analyzed and their respective values presented as mean ± SEM in the graphic (right). Symbols above bars represent statistical difference of p < 0.05 in the Student’s t-test. *CON versus MSG; •MSG versus MSG Extract.
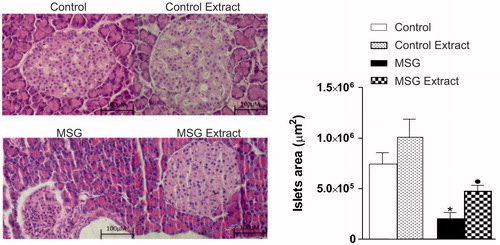
Antioxidant activity and total phenolics content
The B. dracunculifolia extract solution showed ability to scavenge DPPH and ABTS+ radicals and ferric reducing power. The antioxidant activities detected in B. dracunculifolia (plant extract) and the extract employed in the biological model (extract solution) are shown in .
Figure 5. Extraction of bioactive from B. dracunculifolia plant extract with: *ethanol, **methanol, ***acetone.
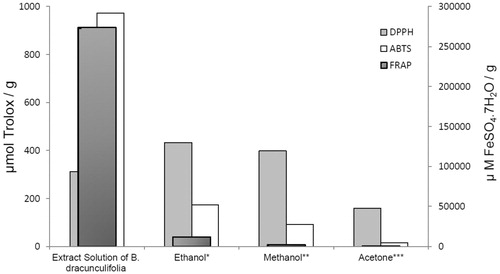
shows that the antioxidant activity varied according to the solvent used (ethanol, methanol, or acetone). The ethanol and methanol plant extracts showed higher antioxidant activities than the acetone plant extract in both DPPH and ABTS assay methods. Similarly, the ethanol plant extract showed higher ferric reducing power than the methanol and acetone plant extracts.
The standard gallic acid curve equation () was determined as y = 0.010 X − 0.0109, R2 = 0.9968. The results showed that B. dracunculifolia is a rich source of phenolics compounds (). Ethanol and methanol were the best extracting solvents (46.2 and 70.5 mg GAE/L, respectively). The phenolics content in the extract solution (128.6 mg/GAE/L) was markedly higher than that measured in the plant extract (methanolic, ethanolic, or acetone extractions).
Table 2. Total phenolics content in extract from Baccharis dracunculifolia.
Discussion
Obesity, which is associated with an accumulation of visceral fat and is the main risk factor for type 2 diabetes mellitus, is responsible for triggering changes in lipid profiles. This fat accumulation can lead to a state of insulin resistance, characterized by reduction in insulin levels that are relevant to inhibit glucose production by the liver and stimulate glucose uptake by the muscle and adipose tissues (Leahy, Citation2005; Weiss et al., Citation2007).
The characterization of obesity was confirmed using the Lee index and the visceral fat deposits and subcutaneously (inguinal) contents. The Lee Index was higher in animals in the MSG group compared to animals in the Control group, which characterized induction of obesity in these animals (Lemos et al., Citation2013; Scomparin et al., Citation2009).
Monosodium glutamate (MSG) animals showed alterations in the metabolism of plasma lipoproteins, with meaningful content found in elevated triglycerides, compared to control animals. This result indicate that the extract solution failed to restore the lipid parameters in MSG animals.
Despite the slightly higher serum insulin and glucose levels in the MSG group compared to the other groups, hyperglycemia or exacerbated insulinemia were not observed, indicating that the obesity model did not induce a significant increase in insulin resistance. However, treatment with MSG promoted a significant reduction in the area under the pancreatic islets in both control groups (). This result indicates that the treatment with MSG induced damage in pancreatic islets, which probably compromised signaling mechanisms for insulin secretion. Interestingly, treatment with B. dracunculifolia extract solution promoted a protective effect in the loss of the area over pancreatic islets. Moreover, this effect could be confirmed in an in vitro experiment where islets from obese rats treated with B. dracunculifolia extract solution had a remarkable amplified response (99.5%) in insulin secretion when treated with increasing doses of glucose (). Therefore, the B. dracunculifolia extract solution could be triggering a protective effect, probably via an antioxidant system, to damages caused by the MSG induced-obesity model. Other studies have characterized the B. dracunculifolia extract solution and reported that the most important components are aglycone flavonoids, phenolic compounds, derivatives of coumaric acid, and other compounds (Marcucci et al., Citation2001) with antioxidant action and properties to prevent exacerbated oxidative stress that correlates with increased insulin resistance (Ueda et al., Citation2013) and damages in pancreatic islets (Zhu et al., Citation2011).
The direct correlation observed between the polarity in the solvent and the antioxidant compounds shows that the solvent used in the extraction influences the antioxidant activity.
The ethanolic and methanolic plant extracts of B. dracunculifolia exhibited significant DPPH and ABTS•+ free radical scavenging ability and ferric reducing power, demonstrating the high antioxidant capacity of this plant. The ethanolic and methanolic plant extracts presented higher DPPH radical scavenging activity than the acetone plant extract (433, 398, and 158.7 mmol Trolox/g, respectively). This result indicates that stronger polar solvents are more suitable for the extraction of bioactives from plant samples.
Martinez-Correa et al. (Citation2012) describe the DPPH free radical scavenging activity in extracts from B. dracunculifolia leaves obtained by a combination of extraction processes with supercritical CO2, ethanol, and water. The authors obtained the highest DPPH free radical scavenging activity (EC50 <200 g/mL) when using water as the extracting solvent and suggested that the antioxidant activity in extracts is strongly dependent on the solvent used, which extracts substances with different DPPH radical scavenging activity.
The ABTS assay also showed that the highest antioxidant activity (173.4 mmol Trolox/g) was obtained with ethanol as extracting solvent, followed by methanol (92.1 mmol Trolox/g) and acetone (15.9 mmol Trolox/g).
The ethanolic plant extract showed the highest ferric reducing power (FRAP) in (11 681.0 µM FeSO4·7H2O/g), followed by the methanolic (2054.0 µM FeSO4·7H2O/g) and acetone plant extract (300.98 µM FeSO4·7H2O/g).
Likewise, the solution formulated from the concentrate B. dracunculifolia extract that was applied in the biological model demonstrated excellent antioxidant capacity.
The ABTS free radical scavenging capacity (972.8 mmol Trolox/g) and ferric reducing power (273 669.0 µM FeSO4·7H2O/g) detected in the solution of extract were significantly higher than those detected in the plant extract (methanolic, ethanolic, or acetone extractions). Conversely, the DPPH radical scavenging activity (311.4 mmol Trolox/g) in the solution of extract was slightly lower than that detected in the plant ethanol or methanol extracts. It is possible that the methanol solvent used to obtain the concentrated B. dracunculifolia extract solution contributed to an inferior extraction of compounds with DPPH radical scavenging activity.
The higher total phenolic content observed in the solution of extract compared to plant extracts shows a correlation between phenolic content and free radical scavenging activity and ferric reducing power.
Phenolic compounds are antioxidant agents, and their bioactivities may be related to their capacity to scavenge free radicals, chelate metals, and inhibit lipoxygenase (Sahu & Saxena, Citation2013). The high content of phenolic compounds observed in the solution of extract used in the biological model suggest that these compounds contribute to a high antioxidant activity.
Although the MSG obesity model used in this study showed a significant increase in the anthropometric variables (Lee index, Mesenteric fat, Periepididymal fat, and Retroperitoneal fat) and blood levels of triglycerides, the model was not effective in triggering insulin resistance according to the HOMA-IR results presented in . However, a better glucose dose dependent response, considering insulin secretion (8.3 and 16.7 mM glucose), was only observed in vitro, in isolated islets, in the obese group treated with B. dracunculifolia. This result is particularly interesting because it shows that isolated islets in obese animals only produce more insulin when treated with the extract solution. The extract used in this study showed high levels of total phenolic content () and high antioxidant activity, demonstrated by three different methods (). Phenolic compounds are related to high antioxidant and chelating activities (Sahu & Saxena, Citation2013). Antidiabetic and β-pancreatic cells regenerative properties have been assigned to these compounds because they lead to increased insulin secretion, significant α-amylase and α-glucosidase inactivation in the digestive system, and increased glucose uptake in the skeletal muscle (Mohan & Nandhakumar, Citation2014). Hence, the B. dracunculifolia extract can be considered as a promising antioxidant bioproduct with high potential use in drug formulation.
It has been argued recently that the compound Artepillin C from B. dracunculifolia can have a specific antidiabetic action increasing the expression of glucose transporter proteins (GLUT 1 and 4) and adiponectines in cell culture of adipocytes from 3T3-L1 mice (genetically pre-disposed to insulin resistance), and reducing the deleterious action of TNF-α (Choi et al., Citation2011). Other compounds present in B. dracunculifolia such as flavonoids, phenolic compounds, and coumarins have shown antidiabetic effects as reported by Abad and Bermejo (Citation2007).
Conclusions
Results demonstrate that the treatment with B. dracunculifolia extract solution can lead to protection of pancreatic islets and prevention of irreversible cellular damages observed in obesity and diabetes models, thus attenuating obesity clinical consequences such as hyperglycemia and efficiency in mechanisms involved in insulin secretion, which are clinical signs related with the clinical evolution of chronic degenerative diseases as obesity and diabetes. Future studies could contribute to establishing the control of preventive and protective effects of B. dracunculifolia extracts in obesity and diabetes models and could lead to applications in future clinical studies including relevant potential to improve the life and treatment of patients affected by these diseases.
Declaration of interest
The authors report no declarations of interest. This manuscript has been reviewed by a professional science editor and a native English-speaking science editor. The authors are thankful to CAPES (Brazil) and Paraná Araucaria Foundation (Brazil) for the financial support to this study.
References
- Abad MJ, Bermejo P. (2007). Baccharis (Compositae): A review update. ARKIVOC 7:76–96
- Akash MS, Rehman K, Chen S. (2013a). Role of inflammatory mechanisms in pathogenesis of type 2 diabetes mellitus. J Cell Biochem 114:525–31
- Akash MS, Rehman K, Chen S. (2013b). An overview of valuable scientific models for diabetes mellitus. Curr Diabetes Rev 9:286–93
- Akash MS, Rehman K, Chen S. (2013c). Effects of coffee on type 2 diabetes mellitus. Nutrition 30:755–63
- Akash MS, Rehman K, Chen S. (2014). Spice plant Allium cepa: Dietary supplement for treatment of type 2 diabetes mellitus. Nutrition 30:1128–37
- Akash MS, Rehman K, Rasool F, et al. (2011). Alternate therapy of type 2 diabetes mellitus (T2DM) with Nigella (Ranunculaceae). J Med Plants Res 5:6885–9
- Akash MS, Shen Q, Rehman K, Chen S. (2012). Interleukin-1 receptor antagonist: A new therapy for type 2 diabetes mellitus. J Pharm Sci 101:1647–58
- Benzie IFF, Strain JJ. (1996). The ferric reducing ability of plasma (FRAP) as a measure of antioxidant power: The FRAP assay. Anal Biochem 239:70–6
- Bernardis LL, Patterson BD. (1968). Correlation between ‘Lee index' and carcass fat content in weanling and adult female rats with hypothalamic lesions. J Endocrinol 40:527–8
- Brazilian Pharmacopoeia. (1988). 4th ed. Ateneu: São Paulo
- Brown SL, Chaney RL, Angle JS, Baker AJM. (1994). Phytoextraction potential of Thlaspi caerulescens and Bladder Campion for zinc and cadmium-contaminated soil. J Environ Q 23:1151–7
- Choi SS, Cha BY, Iida K, et al. (2011). Artepillin C, as a PPARγ ligand, enhances adipocyte differentiation and glucose uptake in 3T3-L1 cells. Biochem Pharmacol 81:925–33
- Hirata AE, Andrade IS, Vaskevicius P, Dolnikoff MS. (1997). Monosodium glutamate (MSG) – Obese rats develop glucose intolerance and insulin resistance to peripheral glucose uptake. Braz J Med Biol Res 30:671–4
- Huang MH, Huang SS, Wang BS, et al. (2011). Antioxidant and anti-inflammatory properties of Cardiospermum halicacabum and its reference compounds ex vivo and in vivo. J Ethnopharmacol 133:743–50
- Labbe C, Rovirosa J, Faini F, et al. (1986). Secondary metabolites from Chilean Baccharis species. J Nat Prod 49:517–18
- Lacy PE, Kostianovsky M. (1967). Method of the isolation of intact islets of Langerhans from rat pancreas. Diabetes 16:35–9
- Leahy JL. (2005). Pathogenesis of Type 2 diabetes mellitus. Arch Med Res 36:197–209
- Lemos LC, Pochapski JA, Raczenski A, et al. (2013). Effect of treatment with MSG on growth, satiety and epididymal adiposity in neonatal rats. J Appl Pharm Sci 3:021–5
- Lemos M, Barros MP, Sousa JPB, et al. (2007). Baccharis dracunculifolia, the main botanical source of Brazilian green propolis, displays antiulcer activity. J Pharm Pharmacol 59:603–8
- Macho L, Ficková M, Jezová D, Zórad S. (2000). Late effects of postnatal administration of monosodium glutamate on insulin action in adult rats. Physiol Res 49:79–85
- Marcucci MC, Ferreres F, García-Viguera C, et al. (2001). Phenolic compounds from Brazilian propolis with pharmacological activities. J Ethnopharmacol 74:105–12
- Martinez-Correa HA, Cabral FA, Magalhães PM, et al. (2012). Extracts from the leaves of Baccharis dracunculifolia obtained by a combination of extraction processes with supercritical CO2, ethanol and water. J Supercritical Fluids 63:31–9
- Matthews DR, Hosker JP, Rudenski AS, et al. (1985). Homeostasis model assessment: Insulin resistance and beta-cell function from fasting plasma glucose and insulin concentrations in man. Diabetologia 28:412–19
- Mohan S, Nandhakumar L. (2014). Role of various flavonoids: Hypotheses on novel approach to treat diabetes. J Med Hypoth Idea 8:1–6
- Morris MJ, Tortelli CF, Filippis A, Proietto J. (1998). Reduced BAT function as a mechanism for obesity in the hypophagic, neuropeptide Y deficient monosodium glutamate treated rats. Regul Pept 25:441–7
- Olney JW. (1969). Brain lesions, obesity, and other disturbances in mice treated with monosodium glutamate. Science 164:719–21
- Rahalison L, Benathan M, Monod M, et al. (1995). Antifungal principles of Baccharis pedunculata. Planta Med 61:360–2
- Sahu R, Saxena J. (2013). Screening of total phenolic and flavonoid content in conventional and non-conventional species of Curcuma. J Pharmacogen Phytochemist 2:176–9
- Scomparin DX, Gomes RM, Grassiolli S, et al. (2009). Monosodium glutamate (MSG)-induced obesity provokes autonomic changes. Endocrinol Diabetes Clin Exp 9:962–4
- Scott RA, Cornelius SG, Mersmann HJ. (1981). Effects of age on lipogenesis and lipolysis in lean and obese swine. J Anim Sci 52:505–11
- Seo EK, Lee D, Shin YG, et al. (2003). Bioactive prenylated flavonoids from the stem bark of Artocarpus kemando. Arch Pharm Res 26:124–7
- Silva LA, Pereira R, Túrmina J, et al. (2014). Sulfonylurea induction of caffeine-enhanced insulin secretion and reduction of glycemic levels in diabetic rats. Pharm Biol 1:1–5
- Singleton VL, Rossi JA. (1965). Colorimetry of total phenolics with phosphomolybdic–phosphotungstic acid reagents. Am J Enol Viticult 16:144–58
- Thetsrimuang C, Khammuang S, Chiablaem K, et al. (2011). Antioxidant properties and cytotoxicity of crude polysaccharides from Lentinus polychrous Lév. Food Chem 128:634–9
- Trinder P. (1969). Determination of blood glucose using an oxidase-peroxidase system with a non-carcinogenic chromogen. Ann Clin Biochem 6:24–7
- Ueda M, Hayashibara K, Ashida H. (2013). Propolis extract promotes translocation of glucose transporter 4 and glucose uptake through both PI3K- and AMPK-dependent pathways in skeletal muscle. Biofactors 39:457–66
- Voltera AF, Mário LR, Cesaretti MG, Kohlmann Jr O. (2008). Effects of neuroendocrine obesity induction on systemic hemodynamics and left ventricular function of normotensive rats. Braz Arch Endocrinol Metab 52:47–54
- Weiss EP, Brandauer J, Kulaputana O, et al. (2007). FABP2 Ala54Thr genotype is associated with glucoregulatory function and lipid oxidation after a high-fat meal in sedentary nondiabetic men and women. Am J Clin Nutr 85:102–8
- Zdero C, Bohlmann F, Solomon JC, et al. (1989). ent-Clerodanes and other constituents from Bolivian Baccharis species. Phytochemistry 28:531–42
- Zhu W, Chen M, Shou Q, et al. (2011). Biological activities of Chinese propolis and Brazilian propolis on streptozotocin-induced type 1 diabetes mellitus in rats. Evid Based Complement Alternat Med 2011:468529