Abstract
Context: The molecular mechanism of doxorubicin (DOX) cardiotoxicity involves overproduction of free radicals that leads to intracellular calcium dysregulation and apoptosis. Mangiferin (MGR), a naturally occurring glucosylxanthone, has antioxidant and cardioprotective properties. However, its cardioprotection mechanism has yet to be revealed.
Objective: This study determines whether the cardioprotective effect of MGR is caused by its effect on intracellular calcium regulation.
Materials and methods: Male Sprague–Dawley rats were induced by DOX intraperitoneally with a total dose of 15 mg/kg bw. MGR was given orally at the doses of 30 and 60 mg/kg bw/d for seven consecutive weeks. The parameters examined were mRNA expression levels of proinflammatory cytokine gene (TNF-α), calcium regulatory gene (SERCA2a) and proapoptotic genes (caspase-9 and caspase-12), as well as cytosolic and mitochondrial calcium levels.
Results: Treatment with MGR at 60 mg/kg bw/d significantly decreased the mRNA expression levels of TNF-α by 44.55% and caspase-9 by 52.79%, as well as the cytosolic calcium level by 24.15% (p < 0.05). SERCA2a and caspase-12 expressions were only slightly affected (27.27% increase and 24.85% decrease for SERCA2a and caspase-12, respectively, p > 0.05). Meanwhile, MGR 30 mg/kg bw/d gave insignificant results in all parameters.
Discussion and conclusion: MGR protected against DOX-induced cardiac inflammation and apoptosis via down-regulation of proapoptotic and proinflammatory gene expressions, upregulation of SERCA2a gene expression, and normalization of cytosolic calcium level. Thus, the cardioprotective effect of MGR is at least in part due to the regulation of intracellular calcium homeostasis.
Introduction
Doxorubicin (DOX) has been widely used as an anticancer agent due to its excellent efficacy. This agent is used to treat various types of malignancy, especially solid tumors, and is currently the standard therapy for metastatic sarcoma in the US (Chu & Sartorelli, Citation2009). However, the clinical use of DOX has been limited due to its toxicity on various vital organs, particularly heart, kidney, and brain (US National Cancer Institute, Citation2014).
Cardiotoxicity is one of the most common forms of DOX toxicity, with an incidence rate of 11% and 1.7% for acute and chronic cardiotoxicity, respectively (Takemura & Fujiwara, Citation2007). Oxidative stress has been proved to be the major cause of DOX cardiotoxicity. It has been widely acknowledged that oxidative stress can cause various intracellular disturbances by disrupting the function and structure of essential macromolecules. One of the most studied hypotheses of molecular mechanism of DOX cardiotoxicity is the effect of oxidative stress to intracellular calcium regulation (Octavia et al., Citation2012).
Intracellular calcium homeostasis is tightly controlled by several regulatory proteins in order to maintain proper contractility of heart muscle. Several studies have proved that reactive oxygen species resulted from DOX treatment has either direct or indirect effect to calcium regulatory proteins (Arai et al., Citation1998; Olson et al., Citation2005; Richard et al., Citation2011). Arai et al. (Citation2000) demonstrated the effect of DOX to the expression and activity of SERCA2a, a calcium pump responsible for calcium influx from cytosol to sarcoplasmic reticulum. H2O2 produced by DOX causes phosphorylation of ERK and its translocation to the nucleus, which initiates Egr-1 transcription. The binding of Egr-1 to the negative regulatory region of SERCA2a gene causes inhibition of SERCA2a transcription. The decrease of SERCA2a expression causes a rise in cytosolic calcium level, leading to the activation of intrinsic apoptotic pathway mediated by caspase-12 (Huang et al., Citation2003; Jang et al., Citation2004). Meanwhile, mitochondrial uptake of calcium is also increased. When a certain threshold of mitochondrial calcium level is reached, a series of events involving mitochondrial permeability transition pore (MPTP) opening is triggered, causing the release of cytochrome-c to the cytosol and the formation of apoptosome, which in turn activates caspase-9 (Halestrap & Pasdois, Citation2009). The activation of caspase-12 and caspase-9 leads to apoptotic cell death. The loss of cardiomyocytes due to apoptosis is one of the major causes of severe contractility impairment which is the prominent feature of DOX cardiotoxicity (Wencker et al., Citation2003).
Although cardiotoxicity continues to be a major problem in DOX therapy, there are not many ideal cardioprotective agents available. Dexrazoxane, an iron chelator derived from EDTA, is currently the only approved cardioprotective agent for DOX therapy. However, there are still certain drawbacks, such as the expensive price and concerns about its negative effect on DOX anticancer activity. In short, a better cardioprotective agent as an alternative to dexrazoxane is still urgently needed.
Mangiferin (MGR) is a C-glucosylxanthone found in many higher plants, including the mango tree. A particular species of mango tree that grows in Indonesia, Mangifera foetida Lour. (Anacardiaceae) (locally called bacang), has been proved to contain higher levels of MGR compared with other mango varieties. Numerous studies have reported the potent antioxidant (Dar et al., Citation2005) and iron-chelating activities (Andreu et al., Citation2005) of MGR. The cardioprotective effect of MGR against DOX-induced cardiotoxicity has been proved in vivo in our previous study (Arozal et al., Citation2014) in which MGR treatment at the dose of 50 mg/kg bw/d was shown to improve biochemical, histological, and electrocardiographic alterations in DOX-induced rats. However, the same study also showed that MGR treatment at 100 mg/kg bw/d had inconsistent positive impact on the heart tissue of test subjects. Therefore, MGR 100 mg/kg bw/d was probably not the optimal dose for rats.
Although MGR cardioprotective effects were well documented, data on its cardioprotection mechanism are scanty. The focus of the present study is to explore the possible molecular mechanism of MGR that involves intracellular calcium regulation. This study is a continuation of our previous study (Arozal et al., Citation2014) which focuses on MGR cardioprotective effect as an effort to enrich the use of evidence-based Indonesian natural herbal medicine.
Materials and methods
Materials
Unless otherwise stated, all reagents were of analytical grade and purchased from Sigma-Aldrich (Nucleos, Singapore) or Merck Millipore (Jakarta, Indonesia). Doxorubicin hydrochloride injection was obtained from Kalbe Pharma (Jakarta, Indonesia). Mangiferin was of analytical grade and obtained from Plamed Science Technology Company (Xi-an, China). RNA isolation, cDNA synthesis, and RT-PCR kits were purchased from Roche (Jakarta, Indonesia).
Animals
This study used male Sprague–Dawley rats aged 12–16 weeks weighing about 180–200 g from BPOM, Jakarta, Indonesia. Rats were kept in a room with constantly controlled temperature (21 °C) and humidity (55%) with a 12 h light/dark cycle. Standard laboratory food and water were given ad libitum. The protocol has been approved by Animal Care Committee from Ethics Committee, Faculty of Medicine, Universitas Indonesia, Jakarta, Indonesia.
DOX and MGR preparation
To prepare DOX, a certain volume of DOX (supplied as 2 mg/mL of DOX in saline 0.9%) was extracted from the vial using syringe in a fume hood. No dilution was needed.
MGR 30 mg/kg bw was prepared by mixing 100 mg of MGR powder with 10 mL of 0.5% sodium CMC using mortar and pestle until MGR was perfectly suspended (Arozal et al., Citation2014). MGR 60 mg/kg bw was prepared with the same procedure, except the amount of MGR powder used was 200 mg.
Experimental design
The dose and the schedule of the study were determined according to our previous study (Arozal et al., Citation2014) with some adjustments. As has been mentioned earlier, the previous higher dose of MGR (100 mg/kg bw/d) was shown giving inconsistent results while the lower dose (50 mg/kg bw/d) gave optimal results. Because of this, for this study, new doses were selected which is slightly lower (30 mg/kg bw/d) and slightly higher (60 mg/kg bw/d) than the previous optimum dose (50 mg/kg bw/d). The dose and the schedule of DOX treatment were selected according to the work of Ibrahim et al. (Citation2009), where the cumulative dose of 15 mg/kg bw of DOX divided in six injections within two weeks was able to induce cardiotoxicity in rats.
After 2 weeks of acclimatization, rats were randomly divided into four groups of five rats each. The groups were as follows: the normal group (normal) which only received carboxymethyl cellulose (CMC) 0.5% and saline (vehicles), the toxic control group (DOX) which only received DOX, and two MGR groups that received both DOX and MGR (the DOX + MGR30 group was given MGR 30 mg/kg bw/d and the DOX + MGR60 group was given MGR 60 mg/kg bw/d, both groups received DOX with the same dose as the DOX group). MGR was given orally every day for seven consecutive weeks in CMC 0.5% as vehicle. DOX were given intraperitoneally with a total dose of 15 mg/kg bw divided in six injections with saline 0.9% as vehicle, starting from the beginning of second week until the end of third week.
Throughout the experiment, rats were monitored closely for signs of toxicity and mortality. Rats were weighed every day. At the end of the seventh week, rats were sacrificed by cervical dislocation method. Hearts were dissected out, washed with saline, weighed, and immediately stored in −80 °C for parameter examination.
Total RNA isolation
Heart tissue was homogenized using Ultra Turrax electric homogenizer. Total RNA was isolated from heart homogenate using Tripure Isolation Reagent (Roche, Jakarta, Indonesia) according to the protocol of the manufacturer. The isolated total RNA concentration and purity were measured spectrophotometrically at 260 nm using Nanodrop 2000 (Thermo Scientific, Waltham, MA). Only the samples with sufficient purity (A260/280 > 1.8) were subjected to the next treatment.
cDNA synthesis
cDNA synthesis reaction was performed using Transcriptor First Strand cDNA Synthesis Kit (Roche, Jakarta, Indonesia). The resulted cDNA concentration and purity was measured spectrophotometrically at 260 nm using Nanodrop 2000 (Thermo Scientific, Waltham, MA).
qRT-PCR
Target genes were amplified with quantitative real-time polymerase chain reaction (qRT-PCR) method using FastStart Essential DNA Green Master (Roche, Jakarta, Indonesia). Primers used for amplification are presented in . Amplification was performed in 45 cycles followed by melting curve analysis. The amplification conditions for each gene were as follows: β-actin (denaturation: 95 °C for 10 s, annealing: 57 °C for 10 s, elongation: 72 °C for 23 s), TNF-α (denaturation: 95 °C for 10 s, annealing: 60 °C for 30 s, elongation: 72 °C for 1 s), SERCA2a (denaturation: 95 °C for 30 s, annealing: 60 °C for 30 s, elongation: 72 °C for 90 s), caspase-12 (denaturation: 95 °C for 10 s, annealing: 60 °C for 10 s, elongation: 72 °C for 30 s), and caspase-9 (denaturation: 95 °C for 10 s, annealing: 57 °C for 10 s, elongation: 72 °C for 13 s).
Table 1. Gene-specific primers used in RT-PCR.
After RT-PCR, the amplification product underwent electrophoresis on 2% agarose gel stained with SYBR Green for band analysis. Only the amplification products that showed one band with the intended product length were quantified. Level of mRNA expression was quantified based on 2 −ΔΔCT method (Livak & Schmittgen, Citation2001).
Isolation of mitochondria
Heart tissue (100–200 mg) was homogenized in buffer A (containing sucrose 0.25 M, Tris-HCl 5 mM, potassium dihydrogen phosphate/KH2PO4 3 mM, magnesium chloride/MgCl2 5 mM and bovine serum albumin/BSA 0.5 mg/mL) using Dounce homogenizer (Thermo Scientific, Waltham, MA). Isolation of mitochondria was performed with the differential centrifugation method (Johnson & Lardy, Citation1967). In brief, homogenate was centrifuged at the speed of 600 × g for 10 min to remove cell debris. Supernatant was then centrifuged at 15 000 × g for 5 min. Supernatant was kept as cytosolic fraction, and pellet was washed with buffer B (containing all components of buffer A except BSA) and centrifuged at 15 000 × g for 5 min. The washing procedure was repeated twice to obtain pure mitochondrial fraction. The temperature was kept at 4 °C throughout the entire process.
Measurement of mitochondrial fraction purity
The purity of isolated mitochondria was determined spectrophotometrically by succinate dehydrogenase (SDH) activity assay (Evans, Citation1978). The reaction mixtures in the cuvette were 652 µL of demineralized water, 120 µL of 800 mM Na phosphate buffer (pH 7.6), 120 µL of 10 mM potassium cyanide (KCN), 240 µL of 100 mM Na succinate, 48 µL of 1 mM 2,6-dichlorophenol indophenol (DCPIP), and 40 µL of sample. The reaction mixture was measured at 600 nm for 3 min with 15 min intervals at 37 °C. SDH activity was expressed as the amount of reduced DCPIP (nmol) per mg protein per minute and presented in . Total protein content was estimated by the method of Bradford (Citation1976).
Table 3. SDH relative specific activity (RSA) of mitochondrial fraction.
Measurement of cytosolic and mitochondrial calcium levels
Cytosolic and mitochondrial calcium levels were measured spectrophotometrically using the o-cresolphthalein complexone (o-CPC) method (Gitelman, Citation1967). Briefly, 500 µL of color reagent (containing 10 mg of o-CPC and 100 mg of 8-hydroxyquinoline in 100 mL of demineralized water), 500 µL of 2-amino-2-methylpropan-1-ol (2-AMP) buffer (pH 10.6), and 25 µL of sample was incubated at room temperature for 15 min and then measured at 540 nm.
Statistical analysis
The results were analyzed using statistical program SPSS Version 19 (SPSS Inc., Chicago, IL). One way ANOVA was employed for comparison among groups, followed by post hoc test using LSD. p < 0.05 was considered to be significant.
Results
Stable growth of body weight was observed in all groups at the first week. However, in all groups receiving DOX (DOX, DOX + MGR30, DOX + MGR60), there was a considerable decrease of body weight which occurred along with DOX treatment (data not shown). DOX, DOX + MGR30, and DOX + MGR60 also showed signs of general acute toxicity, including diarrhea, nose and mouth bleeding, and paleness. Body weight was increased and toxicity signs were gradually improved after DOX treatment ended. A comparison of heart weight (HW) to body weight (BW) data (HW/BW) showed that DOX treatment increased HW/BW significantly in DOX only-treated group compared with the normal group. In groups receiving MGR, HW/BW was mildly decreased compared to DOX only-treated group. Mortality rates were 0% in all groups ().
Table 2. Survival rate, body weight, and heart weight data.
TNF-α mRNA expression level
TNF-α mRNA expression analysis results as depicted in shows that DOX treatment increased TNF-α mRNA expression level significantly (p < 0.05) in the DOX-only treated group compared with the normal group (52.6%), while both groups receiving MGR (DOX + MGR30 and DOX + MGR60) showed decreased TNF-α mRNA expression level compared with the DOX only-treated group (21.33% for DOX + MGR30 and 44.55% for DOX + MGR60). However, the change was only statistically significant at group receiving MGR 60 mg/kg bw (p < 0.05).
Figure 1. Effect of MGR on DOX-induced alteration of TNF-α mRNA expression level. mRNA expression levels are normalized to β-actin as housekeeping gene. Values are presented as mean ± SD (n = 5), ap < 0.05 versus the normal group; bp < 0.05 versus the DOX group; normal = normal group, DOX = doxorubicin 15 mg/kg bw, DOX + MGR30 = DOX 15 mg/kg bw and MGR 30 mg/kg bw/d, DOX + MGR60 = DOX 15 mg/kg bw, and MGR 60 mg/kg bw/d.
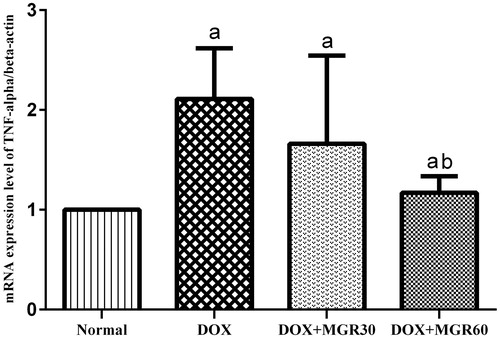
SDH assay
The result of SDH assay presented in showed that the isolated mitochondrial fraction of all samples showed high purity, indicated by the high value of SDH relative specific activity of mitochondrial fraction.
Cytosolic calcium level
shows that in the DOX-only treated group, DOX treatment increased cytosolic calcium level significantly (p < 0.05) compared with the normal group (39.13%). Both doses of MGR decreased cytosolic calcium level (21.01 and 24.15% for DOX + MGR30 and DOX + MGR60, respectively), although the statistically significant decrease was only given by the group receiving 60 mg/kg bw of MGR (p < 0.05).
Figure 2. Effects of MGR on DOX-induced alteration of mitochondrial calcium level (mg/dl). Values are presented as mean ± SD (n = 5), ap < 0.05 versus the normal group; normal = normal group, DOX = doxorubicin 15 mg/kg bw, DOX + MGR30 = DOX 15 mg/kg bw and MGR 30 mg/kg bw/d, DOX + MGR60 = DOX 15 mg/kg bw, and MGR 60 mg/kg bw/d.
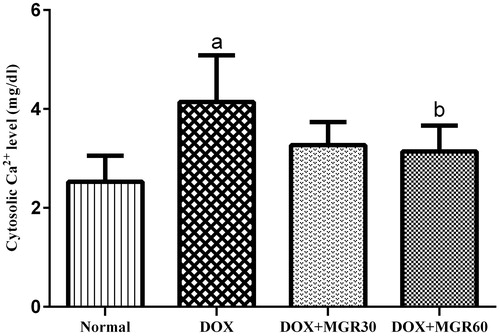
Mitochondrial calcium level
Although DOX decreased calcium level significantly (p < 0.05) compared with the normal group in the DOX only-treated group (52.77%), both groups receiving MGR showed insignificant increase (p > 0.05) compared with the DOX only-treated group, which are 9.23% for DOX + MGR30 and 18.39% for DOX + MGR60 ().
Figure 3. Effects of MGR on DOX-induced alteration of cytosolic calcium level (mg/dl). Values are presented as mean ± SD (n = 5), ap < 0.05 versus the normal group; normal = normal group, DOX = doxorubicin 15 mg/kg bw, DOX + MGR30 = DOX 15 mg/kg bw and MGR 30 mg/kg bw/d, DOX + MGR60 = DOX 15 mg/kg bw, and MGR 60 mg/kg bw/d.
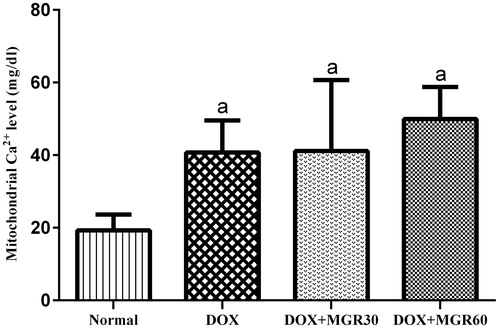
SERCA2a mRNA expression level
DOX treatment caused a significant decrease (p < 0.05) of SERCA2a mRNA expression level in the DOX only-treated group when compared with the normal group (44.0%). Both MGR-treated groups showed elevated SERCA2a mRNA expression level as evidenced in (15.15 and 27.27% for DOX + MGR30 and DOX + MGR60, respectively). Although the change was not statistically significant (p > 0.05) compared with the DOX only-treated group, the group receiving MGR 60 mg/kg bw the mRNA expression almost reached normal level.
Figure 4. Effect of MGR on DOX-induced alteration of SERCA2a mRNA expression level. mRNA expression levels are normalized to β-actin as housekeeping gene. Values are presented as mean ± SD (n = 5), ap < 0.05 versus the normal group; normal = normal group, DOX = doxorubicin 15 mg/kg bw, DOX + MGR30 = DOX 15 mg/kg bw and MGR 30 mg/kg bw/d, DOX + MGR60 = DOX 15 mg/kg bw, and MGR 60 mg/kg bw/d.
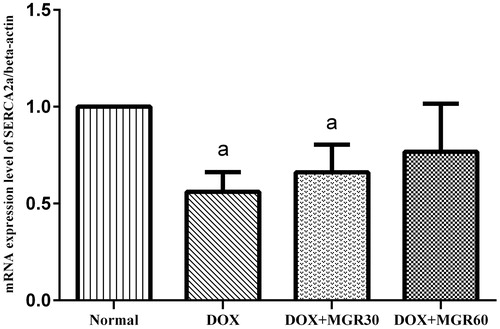
Caspase-12 mRNA expression level
shows that DOX treatment significantly increased (p < 0.05) caspase-12 mRNA expression level in the DOX only-treated group (40.83%). Although MGR treatment of both doses showed insignificant decrease (p > 0.05) of caspase-12 mRNA expression level compared with the DOX only-treated group (15.38 and 24.85% for DOX + MGR30 and DOX + MGR60, respectively), at MGR 60 mg/kg bw the mRNA expression almost reached normal level.
Figure 5. Effect of MGR on DOX-induced alteration of caspase-12 mRNA expression level. mRNA expression levels are normalized to β-actin as housekeeping gene. Values are presented as mean ± SD (n = 5), ap < 0.05 versus the normal group; normal = normal group, DOX = doxorubicin 15 mg/kg bw, DOX + MGR30 = DOX 15 mg/kg bw and MGR 30 mg/kg bw/d, DOX + MGR60 = DOX 15 mg/kg bw, and MGR 60 mg/kg bw/d.
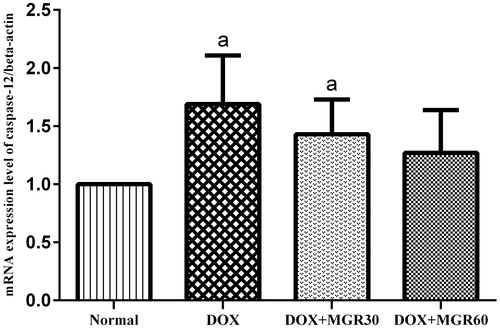
Caspase-9 mRNA expression level
shows that DOX treatment cause a significant increase (p < 0.05) of caspase-9 mRNA expression level in the DOX only-treated group (49.24%), while both doses of MGR decreased it (13.19 and 52.79% for DOX + MGR30 and DOX + MGR60, respectively). A statistically significant decrease was shown with the group receiving MGR 60 mg/kg bw (p < 0.05).
Figure 6. Effect of MGR on DOX-induced alteration of caspase-9 mRNA expression level. mRNA expression levels are normalized to β-actin as housekeeping gene. Values are presented as mean ± SD (n = 5), ap < 0.05 versus the normal group; bp < 0.05 versus the DOX group; normal = normal group, DOX = doxorubicin 15 mg/kg bw, DOX + MGR30 = DOX 15 mg/kg bw and MGR 30 mg/kg bw/d, DOX + MGR60 = DOX 15 mg/kg bw, and MGR 60 mg/kg bw/d.
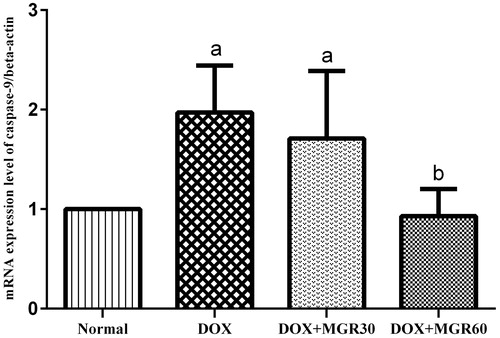
Discussion
Our results showed that a total dose of 15 mg/kg bw of DOX was able to cause cardiac toxicity as evidenced by elevated cardiac pro-inflammatory gene expression. Furthermore, there were evidences of disturbed intracellular calcium homeostasis and apoptotic cardiomyocyte death as shown by elevated mitochondrial and cytosolic calcium levels, decreased calcium regulatory gene expression, and elevated caspases expression. Treatment with MGR at both 30 and 60 mg/kg bw/d was able to decrease inflammation, hence exhibiting a cardiac protective effect. This effect is likely associated with MGR-induced intracellular calcium regulation since MGR treatment exhibited lower level of intracellular calcium, higher expression of calcium regulatory gene, and lower expression of caspases. To the best of our knowledge, this is the first study that revealed intracellular calcium regulation as a molecular mechanism of MGR cardioprotective effect.
In this study, signs of toxicity and body weight decrease were observed in all groups receiving DOX (DOX, DOX + MGR30, and DOX + MGR60) after DOX treatment. However, the gradual improvement of body weight that occurred after DOX treatment ended did not differ among groups receiving MGR (DOX + MGR30 and DOX + MGR60) and not receiving MGR (DOX). There were no deaths observed in all groups. Considering body weight and mortality rate data, it can be concluded that MGR seemed to have no effect on general acute toxicity.
Inflammation marker examination revealed that MGR indeed showed protective effect against DOX-induced cardiac inflammation as indicated by the significant decrease of TNF-α mRNA expression level in all groups receiving MGR compared to group only receiving DOX. Instead of cardiac biochemical markers, TNF-α expression was used to assess cardiac damage because our previous work has already established that 15 mg/kg bw of DOX was able to cause significant increase of serum CK and LDH levels (Arozal et al., Citation2014). TNF-α has been widely accepted as an appropriate surrogate marker to assess heart damage because of strong positive correlation between its level and the degree of heart damage (Torre-Amione et al., Citation1996a,Citationb). In this study, MGR-induced decrease of TNF-α mRNA expression level confirmed the ability of MGR to ameliorate heart damage caused by DOX. This result was in line with our previous study that showed significant improvement in biochemical and histological parameters with MGR treatment in DOX-treated rats (Arozal et al., Citation2014).
Mitochondrial and cytosolic calcium levels were analyzed to assess the effect of MGR on intracellular calcium homeostasis. As has been confirmed by many studies, DOX causes marked increase on cytosolic calcium level as a direct effect of disturbed calcium regulation, which in turn causes mitochondrial calcium overload (Kapelko et al., Citation1996; Zhou et al., Citation2001). In this study, it was found that MGR treatment was able to ameliorate DOX-induced increase on cytosolic calcium level. However, mitochondrial calcium level was somehow inversely affected. There are several possible explanations for this contradictive result. First, MGR probably has direct effect on mitochondrial calcium uptake transporter activity, as has been reported for several other flavonoids (Montero et al., Citation2004). Second, MGR might affect mitochondrial calcium transporter expression, since evidence showed that MGR has the capability to modulate certain gene expressions (Leiro et al., Citation2004). These hypotheses require further investigation. However, since MGR-induced mitochondrial calcium increase was hardly significant (p > 0.05), it can be assumed that it is not harmful for mitochondria. It is entirely possible that the mild increase of mitochondrial calcium level might be beneficial for respiration enzymes activity (Denton, Citation2009).
SERCA2a was suspected as a potential target for MGR since this protein is the central player in intracellular calcium regulation. DOX has been consistently found to decrease SERCA2a mRNA and protein levels (Arai et al., Citation1998; Olson et al., Citation2005; Richard et al., Citation2011), and SERCA2a impairment is closely associated with decreased heart contractility in DOX cardiotoxicity (Frank et al., Citation2003; Periasamy & Huke, Citation2001). In this study, DOX treatment caused significant decrease (p < 0.05) of SERCA2a mRNA expression level in the DOX only-treated group which can partially explain the rise of cytosolic and mitochondrial calcium levels. MGR treatment was shown to increase SERCA2a mRNA expression level, albeit not statistically significant (p > 0.05). Nevertheless, this study revealed for the first time that MGR can indeed restore SERCA2a expression level. Considering the subsequent decrease on cytosolic calcium level, it can be concluded that this mechanism is at least partly responsible for the cardioprotective effect of MGR. However, it is still uncertain whether this is due to direct effect of MGR or indirect effect of its antioxidant activity. Again, further study is required to prove this hypothesis.
Proapoptotic genes expression analysis was performed to assess the effect of MGR on downstream apoptotic pathway of intracellular calcium regulation. In this study, it was found that in the DOX-only treated group, DOX treatment caused a significantly higher expression of caspase-12, and even more so for caspase-9. The higher increase of caspase-9 is probably due to the fact that caspase-9 can be activated by nearly every pathway that cause MPTP opening, while caspase-12 is specifically activated by intracellular calcium dysregulation (Morishima et al., Citation2002). The same reason can probably be applied to explain the lower effect of MGR on caspase-12 compared with caspase-9 in this study. MGR has been proved to protect mitochondria from ultrastructure modification, oxygen metabolism impairment, and lipid peroxidation (Prabhu et al., Citation2006). The broad effects of MGR on DOX-induced mitochondrial damage supported the notion that intracellular calcium regulation is only partially responsible for its cardioprotective effect. However, in this study, MGR-induced significant decrease of caspase-9 expression seemed contradictory to the increased mitochondrial calcium level. This is probably because mitochondrial ROS level can significantly impact the calcium-induced MPTP opening threshold. At high mitochondrial ROS level which was associated with DOX treatment, MPTP opening threshold is decreased, making mitochondria more prone to calcium-induced intrinsic apoptotic pathway activation mediated by caspase-9 (Connern & Halestrap, Citation1994). On the contrary, it is highly possible that MGR-induced decrease of mitochondrial ROS level has increased the threshold, confirming a significant decrease of caspase-9 mRNA expression level.
Conclusions
Based on the overall results, it can be concluded that MGR causes normalization of intracellular calcium homeostasis, which is partly due to the positive effect of MGR on SERCA2a mRNA expression level. As a result, DOX-induced intrinsic apoptotic pathways activation and the subsequent heart damage is reduced. Hence the study showed that the cardioprotective effect of MGR is partly due to intracellular calcium regulation.
Authors’ contributions
F. D. A. designed the study and performed the experimental work and data analysis. W. A. and M. L. supervised the experimental work. S. S. performed the experiments. V. S., N. N., and F. D. S. served as scientific advisors and helped edited the manuscript. All authors read and approved the final manuscript.
Declaration of interest
The authors report that they have no conflicts of interest. This study was partially funded by a grant from DRPM UI, Depok, Indonesia. The authors express deep gratitude to all technicians in Animal Housing Facility and researchers in Pharmacokinetics Laboratory, Department of Pharmacology and Therapeutics, Universitas Indonesia, for their assistance in this study.
References
- Andreu GP, Delgado R, Velho JA, et al.. (2005). Iron complexing activity of mangiferin, a naturally occurring glucosylxanthone, inhibits mitochondrial lipid peroxidation induced by Fe2+-citrate. Eur J Pharmacol 13:47–55.
- Arai M, Tomaru K, Takizawa T, et al.. (1998). Sarcoplasmic reticulum genes are selectively down-regulated in cardiomyopathy produced by doxorubicin in rabbits. J Mol Cell Cardiol 30:243–54.
- Arai M, Yoguchi A, Takizawa T, et al.. (2000). Mechanism of doxorubicin-induced inhibition of sarcoplasmic reticulum Ca2+-ATPase gene transcription. Circ Res 86:8–14.
- Arozal W, Suyatna FD, Juniantito V, et al.. (2014). The effects of mangiferin (Mangifera indica L.) in doxorubicin-induced cardiotoxicity in rats. Drug Res 64:1–7.
- Bradford MM. (1976). A rapid and sensitive method for the quantification of microgram quantities of protein utilizing the principle of protein-dye binding. Anal Biochem 72:248–54.
- Chu E, Sartorelli AC. (2009). Cancer Chemotherapy. In: Katzung BG, Masters SB, Trevor AJ, eds. Katzung’s Basic and Clinical Pharmacology, 11th ed. New York: McGraw-Hill, 935–63.
- Connern CP, Halestrap AP. (1994). Recruitment of mitochondrial cyclophilin to the mitochondrial inner membrane under conditions of oxidative stress that enhance the opening of a calcium-sensitive non-specific channel. Biochem J 302:321–4.
- Dar A, Faizi S, Naqvi S, et al.. (2005). Analgesic and antioxidant activity of mangiferin and its derivatives: The structure activity relationship. Biol Pharm Bull 28:596–600.
- Denton RM. (2009). Regulation of mitochondrial dehydrogenases by calcium ions. Biochim Biophys Acta 1787:109–16.
- Evans HW. (1978). Preparation and characterization of mammalian plasma membranes. In: Work TS, Work E, eds. Laboratory Techniques in Biochemistry and Molecular Biology. North Holland: Elsevier, 93–176.
- Frank KF, Bolck B, Erdmann E, et al.. (2003). Sarcoplasmic reticulum Ca -ATPase modulates cardiac contraction and relaxation. Cardiovasc Res 57:20–7.
- Gitelman H. (1967). Clinical chemistry: Calcium o-cresol pthtalein complex one method. Anal Biochem 20:521–3.
- Halestrap AP, Pasdois P. (2009). The role of the mitochondrial permeability transition pore in heart disease. Biochim Biophys Acta 1787:1402–15.
- Huang XM, Zhu WH, Kang ML. (2003). Study on the effect of doxorubicin on expressions of genes encoding myocardial sarcoplasmic reticulum Ca2+ transport proteins and the effect of taurine on myocardial protection in rabbits. J Zhejiang Univ Sci 4:114–20.
- Ibrahim MA, Ashour OM, Ibrahim YF, et al.. (2009). Angiotensin-converting enzyme inhibition and angiotensin AT(1)-receptor antagonism equally improve doxorubicin-induced cardiotoxicity and nephrotoxicity. Pharmacol Res 60:373–81.
- Johnson D, Lardy H. (1967). Isolation of liver and kidney mitochondria. In: Estabrook RW, Pullman ME, eds. Methods in Enzymology. New York: Academic Press, 94–6.
- Jang YM, Kendaiah S, Drew B, et al.. (2004). Doxorubicin treatment in vivo activates caspase-12 mediated cardiac apoptosis in both male and female rats. FEBS Lett 577:483–90.
- Kapelko VI, Williams CP, Gutstein DE, et al.. (1996). Abnormal myocardial calcium handling in the early stage of adriamycin cardiomyopathy. Arch Physiol Biochem 104:185–91.
- Leiro J, Arranza JA, Yáñezb M, et al.. (2004). Expression profiles of genes involved in the mouse nuclear factor-kappa B signal transduction pathway are modulated by mangiferin. Int Immunopharmacol 4:763–78.
- Livak KJ, Schmittgen TD. (2001). Analysis of relative gene expression data using real-time quantitative PCR and the 2-ΔΔCt method. Methods 25:402–8.
- Montero M, Lobat’on CD, Hernandez-Sanmiguel E, et al.. (2004). Direct activation of the mitochondrial calcium uniporter by natural plant flavonoids. Biochem J 384:19–24.
- Morishima N, Nakanishi K, Takenouchi H, et al.. (2002). An endoplasmic reticulum stress-specific caspase cascade in apoptosis: Cytochrome c-independent activation of caspase-9 by caspase-12. J Biol Chem 277:34287–94.
- Octavia Y, Tocchetti CG, Gabrielson KL, et al.. (2012). Doxorubicin-induced cardiomyopathy: From molecular mechanisms to therapeutic strategies. J Mol Cell Cardiol 52:1213–25.
- Olson RD, Gambliel HA, Vestal RE, et al.. (2005). Doxorubicin cardiac dysfunction: Effects on calcium regulatory proteins, sarcoplasmic reticulum, and triiodothyronine. Cardiovasc Toxicol 5:269–83.
- Periasamy M, Huke S. (2001). SERCA pump level is a critical determinant of Ca2+ homeostasis and cardiac contractility. J Mol Cell Cardiol 33:1053–63.
- Prabhu S, Mallika J, Sabitha KE, et al.. (2006). Cardioprotective effect of mangiferin on isoproterenol induced myocardial infarction in rats. Ind J Exp Biol 44:209–15.
- Richard C, Ghibu S, Delemasure-Chalumeau S, et al.. (2011). Oxidative stress and myocardial gene alterations associated with doxorubicin-induced cardiotoxicity in rats persist for 2 months after treatment cessation. JEPT 339:807–14.
- Takemura G, Fujiwara H. (2007). Doxorubicin-induced cardiomyopathy from the cardiotoxic mechanism to management. Prog Cardiovasc Disc 4:330–52.
- Torre-Amione G, Kapadia S, Benedict C, et al.. (1996a). Proinflammatory cytokine levels in patients with depressed left ventricular ejection fraction: A report from the studies of left ventricular dysfunction (SOLVD). J Am Coll Cardiol 27:1201–6.
- Torre-Amione G, Kapadia S, Lee J, et al.. (1996b). Tumor necrosis factor-alpha and tumor necrosis factor receptors in the failing human heart. Circulation 93:704–11.
- US National Cancer Institute. (2014). Adult soft tissue sarcoma treatment (PDQ®): Stage IV adult tissue sarcoma.[Online]. Available from: http://www.cancer.gov/cancertopics/pdq/treatment/adult-soft-tissue-sarcoma/HealthProfessional/page8 [last accessed 1 Jun 2014].
- Wencker D, Chandra M, Nguyen K, et al.. (2003). A mechanistic role for cardiac myocyte apoptosis in heart failure. J Clin Invest 111:1497–504.
- Zhou S, Starkov A, Froberg MK, et al.. (2001). Cumulative and irreversible cardiac mitochondrial dysfunction induced by doxorubicin. Cancer Res 61:771–7.