Abstract
Context: The flower bud of Tussilago farfara L. (Compositae) (FTF) is one of the traditional Chinese medicinal herbs used to treat cough, phlegm, bronchitic, and asthmatic conditions.
Objective: The objective of this study is to isolate four caffeoylquinic acids from the ethyl acetate extract (EtE) of FTF and to evaluate their antitussive, expectorant, and anti-inflammatory activities.
Materials and methods: The structures of compounds 1–4 isolated from EtE were determined by spectral analysis. Mice were orally treated with these compounds and their mixture (in a ratio of 5:28:41:26 as in EtE) at doses of 10 and 20 mg/kg once daily for 3 d. The antitussive and expectorant activities were evaluated separately with the ammonia liquor-induced model and the phenol red secretion model. The anti-inflammation activity was evaluated using leukocyte count in the bronchoalveolar lavage fluid after ammonia liquor-induced acute airway inflammation.
Results: The four compounds were identified as chlorogenic acid (1), 3,5-dicaffeoylquinic acid (2), 3,4-dicaffeoylquinic acid (3), and 4,5-dicaffeoylquinic acid (4). All compounds, especially compound 4 (58.0% inhibition in cough frequency), showed a significant antitussive effect. However, the mixture was the most effective to inhibit the cough frequency by 61.7%. All compounds also showed a significant expectorant effect, while compound 2 was the most potent to enhance the phenol red secretion by 35.7%. All compounds significantly alleviated inflammation, but compound 4 showed the strongest effect to inhibit the leukocytosis by 49.7%.
Discussion and conclusion: The caffeoylquinic acids and their mixture, exhibiting significant antitussive, expectorant, and anti-inflammatory effects, could be considered as the main effective ingredients of FTF, and they may act in a collective and synergistic way.
Introduction
Cough is one of the most common symptoms that lead patients to seek medical care from doctors. Under normal conditions, the primary function of coughing is to protect the respiratory tract from inhaled irritants and the cough reflex is activated by the stimulation of vagal afferent nerves in the extrapulmonary airways and mediated by brainstem (Young & Smith, Citation2011). Under disease conditions, coughing becomes excessive and patients often self-medicate for acute cough associated with transient upper respiratory tract infections (“colds”). However, some serious respiratory diseases, such as asthma, chronic obstructive pulmonary disease (COPD), and lung cancer, have a common symptom of chronic cough (Mackenzie et al., Citation2004). So, the chronic cough is being one more serious medical problem than the acute or subacute cough.
Current antitussive drugs are generally divided into two categories, i.e., central or peripheral ones, based on their site of action. The antitussives suppressing cough by the central pathway comprise the majority of currently used cough suppressants (Reynolds et al., Citation2004). Although opioids including codeine phosphate are the most effective antitussive, their use is limited by undesirable side effects such as addiction (Kamei, Citation2002). Peripheral acting antitussives such as levodropropizine and guaifenesin generally inhibit the responsiveness of airway nerve subtypes that evoke cough, but these drugs are not used widely (Dicpinigaitis, Citation2006). As a result, effective and well-tolerated cough medicines remain to be searched for clinical requirement.
Tussilago farfara L. (Compositae) is one of the common medicinal herbs used in China, North Africa, Siberia, and Europe (Xue et al., Citation2012). The flower bud of T. farfara (FTF) has been used for more than 2000 years for the treatment of cough, phlegm, bronchitic, and asthmatic conditions. It also has been recorded in all editions of Chinese Pharmacopoeia and applied in many Chinese patent medicines. In Eastern Europe, the leaf of T. farfara (coltsfoot) has been used as an herbal remedy for the same applications (Nanjing University of Chinese Medicine, Citation2006). Modern pharmacological experiments have demonstrated that the water extract of FTF exhibited obvious antitussive and expectorant activities (Li et al., Citation2009b; Zhang et al., Citation2009). However, the effective chemical substances and the mechanisms remain to be elucidated. A chemical profiling of 1H NMR and a multivariate data analysis of FTF revealed that chlorogenic acid, 3,5-dicaffeoylquinic acid, and rutin may be closely related with its antitussive and expectorant activities (Li et al., Citation2013). Our previous work showed that the total phenolic acids of FTF markedly enhanced the latent period of cough and inhibited the cough frequency in mice. A LC-MS analysis of the total phenolic acids indicated that the principal components were chlorogenic acid (CA, monocaffeoylquinic acid) and isochlorogenic acids (dicaffeoylquinic acids, DCQA) (Wang, Citation2013).
The part of total phenolic acids of FTF has been demonstrated to have antitussive and expectorant activities. However, it is not clear whether caffeoylquinic acids contribute to the antitussive activity of FTF. Therefore, we isolated the four major caffeoylquinic acids from the refined ethyl acetate extract (EtE, the antitussive part) of FTF, and evaluated their antitussive, expectorant, and anti-inflammatory effects in mice. Moreover, the corresponding activities of a mixture of four compounds in a ratio as in EtE at the same doses were simultaneously tested so as to learn the way in which the compounds may function, singly or synergistically.
Materials and methods
Chemicals and materials
Pentoxyverine, ammonium chloride, and prednisone were purchased from Sinopharm Rongsheng Pharmaceutical Co. (Henan, China), Xilong Chemicals Co. (Guangdong, China), and Zhejiang Xianju Pharmaceutical Co. (Zhejiang, China). Chlorogenic acid (CA, 3-caffeoylquinic acid) was obtained from Nanjing Zelang plant extract Co. (Jiangsu, China, purity >98%). Phenol red was purchased from Nanjing Chemical Reagent Co. (Nanjing, China), and urethane was obtained from Shanghai Qingxi Chemicals Co. (Shanghai, China). Chromatographic grade methanol and formic acid were supplied by Merck (Darmstadt, Germany), and purified water was obtained from Hangzhou Wahaha Company (Zhejiang, China). Other reagents were of analytical grade.
FTF was collected in Bozhou Chinese Medicinal Herbal Market (Bozhou, Anhui province, China) in 2012 and identified by Prof. M. Zhang, one of the authors. The voucher specimens were deposited in our laboratory of China Pharmaceutical University.
Extraction and isolation
The dried FTF (1.4 kg) was crushed and refluxed with 9 L of 70% ethanol for three times, each for 2 h. After expelling the solvent in vacuum, the combined extract (550 g) was suspended in water (adjusted to pH 4.0 with 4% HCL) and extracted with ethyl acetate for three times. The crude ethyl acetate extract was degreased by petroleum ether to obtain the refined ethyl acetate extract (EtE).
EtE (20 g) was separated on a silica gel column acidified with 1% phosphoric acid and eluted by petroleum ether–ethyl acetate (8:1–1:2) to afford six fractions, FrA–FrF. After removal of pigments by Sephadex column, FrD was further separated by semi-preparative HPLC (Shimadzu LC-20AP, Shimadzu Corp., Tokyo, Japan) on a RP-C18 column (Phenomenex Luna C18, 10 mm × 250 mm, 5 μm, Phenomenex Inc., Torrance, CA) eluted by methanol–water (37:63) in 3 mL/min (detected at 280 nm) to yield compounds 2 (260 mg) and 4 (220 mg). FrE was subjected to ODS glass–column chromatography eluted with methanol–water (80:20) to give compounds 1 (180 mg) and 3 (280 mg).
Quantitative analysis of EtE was performed on an Agilent 1260 series system (Agilent Technologies, Atlanta, GA) equipped with an auto sampler, a vacuum degasser, a quarternary pump, and a diode array detector (DAD). Samples were separated on an Agilent Zorbax SB-C18 column (Agilent Technologies, Atlanta, GA) (4.6 mm × 250 mm, 5 μm, Agilent, Atlanta, GA) operated at 25°C with a flow rate of 1 mL/min. The mobile phase consisted of 0.05% phosphoric acid (A) and methanol (B), and the elution program as follows: 0 min, 15% of B; 8 min, 25% of B; 20 min, 30% of B; 21 min, 39% of B; 30 min, 40% of B; 35 min, 48% of B; 43 min, 48% of B; and 50 min, 60% of B. A detective wavelength was set at 280 nm and the injection volume was 10 μL.
Animals and drug administration
ICR mice (18–22 g) were purchased from the Comparative Medicine Center of Yangzhou University (Jiangsu, China), and the animal license was SCXK 2014-0001. All mice were housed at temperature 25 ± 2 °C and relative humidity 50 ± 10% under a standard light-dark cycle. Animal welfare and experimental procedures were carried out in accordance with the Principles of Laboratory Animals and the related ethical regulations of China Pharmaceutical University.
After 3–5 d of adaptation, the animals were randomly divided into groups (10 in each group). All drugs were suspended with 0.5% sodium carboxymethyl cellulose (CMC-Na) solution, and 0.5% CMC–Na solution was taken as control. All mice were treated with test drugs or 0.5% CMC-Na at 0.2 mL/20 g body weight.
Evaluation of antitussive activity
Antitussive effects were investigated with the mouse cough model induced by ammonia liquor (Chen, Citation1994; Wang et al., Citation2012). The mice were exposed to a 500 mL glass jar sprayed with 13% ammonium hydroxide (0.2 mL) to record the latent period and cough frequency. Mice with latent period less than 1 min and the cough frequency more than three times in 1 min were chosen to be eligible animals for the experiments. After 24 h of recovery, the eligible mice were divided into groups randomly and orally given the test drugs once daily for 3 d, using pentoxyverine (17 mg/kg) as the positive control. After 1 h of the last administration, each mouse was placed in a 500 mL glass jar saturated with 0.2 mL 13% ammonia liquor. The latent period and the frequency of cough in 2 min were observed and recorded by a skilled observer.
Evaluation of expectorant activity
Expectorant activity was tested with the model of phenol red secretion in mice (Han et al., Citation2010; Wei et al., Citation2010). Briefly, mice were orally administrated with the test drugs once a day for 3 d, taking ammonium chloride as the positive control. After 30 min of the last administration, the mice were intraperitoneally injected with 2.5% phenol red solution. Thirty minutes later, the mice were anesthetized with 30% ethyl carbamate and killed. After being dissected free from adjacent organs and peeled off connective tissue, the trachea and primary bronchi were taken out and put into 1 mL of 5% sodium bicarbonate solution immediately, ultrasonicated for 15 min, and centrifuged at 3000 rpm for 10 min. The absorbance of the centrifuged supernatant was measured at the wavelength of 546 nm by a microplate reader (BioTek Epoch, Winooski, VT). The standard curve of phenol red was performed as described in literatures (Wei et al., Citation2010).
Evaluation of anti-inflammatory activity
Anti-inflammatory activity was evaluated by the acute airway inflammation induced by ammonia liquor in mice (Li et al., Citation2009a). Mice were orally administered with the test drugs once a day for 3 d. Thirty minutes before the last administration, mice were exposed to ammonia for 2 min except for the control group. Six hours later, the mice were killed by anesthesia with 30% urethane, and bronchoalveolar lavage fluid (BALF) was obtained by injecting 2.1 mL of ice-cold physiological saline (three times, each for 0.7 mL) via a tracheal cannula. The collected BALF (recovery rate of about 80%) was shaken well and the total leukocytes were counted with a cell-count board.
Statistical analysis
Data were expressed as mean ± SE (standard error) and analyzed by one-way analysis of variance (ANOVA) followed by Student’s t-test and/or the Bonferroni correction. Values of p < 0.05 were considered to be statistically significant.
Results
Isolation and structure identification
EtE was obtained from the 70% ethanol extract of FTF and further separated by column chromatography and semi-preparative HPLC leading to the isolation of compounds 1–4, four caffeoylquinic acids (). By comparing the HPLC-DAD chromatogram with that of the authentic compound, the structure of compound 1 was determined as CA. By comparing the 1H and 13C NMR data () with those in literatures (Chen et al., Citation2014), the structures of compounds 2–4 were identified as 3,5-DCQA (isochlorogenic acid A), 3,4-DCQA (isochlorogenic acid B), and 4,5-DCQA (isochlorogenic acid C). A quantitative HPLC determination on EtE showed that the contents of compounds 1–4, being the main constituents in EtE, were 3.7, 21.3, 31.0, and 19.4% (in a ratio of 5:28:41:26), totaled as 75.4% ().
Figure 1. (A) Chemical structures of compounds 1–4 and (B) HPLC chromatogram of EtE portion of the flower bud of Tussilago farfara, (1) chlorogenic acid, (2) 3,5-dicaffeoylqunic acid (isochlorogenic acid A), (3) 3,4-dicaffeoylqunic acid (isochlorogenic acid B), and (4) 4,5-dicaffeoylqunic acid (isochlorogenic acid C).
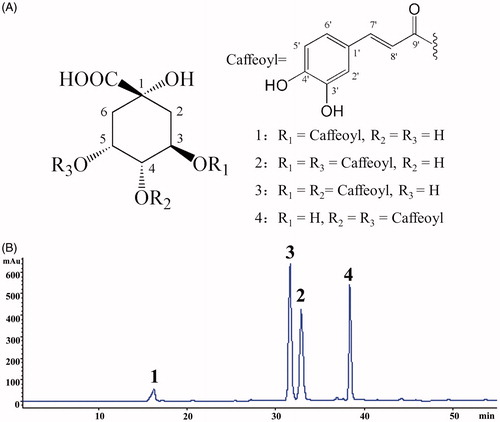
Table 1. 1H NMR (500 MHz, MeOH-d4) and 13C NMR (125 MHz, MeOH-d4) data of compounds 2–4.
Antitussive effects of EtE and a mixture of compounds 1–4
In order to know whether EtE and its main ingredients (compounds 1–4) devote to the antitussive activity of FTF, both EtE and a mixture of compounds 1–4 in the ratio as in EtE (5:28:41:26) were tested for their effects on mouse cough model induced by ammonia liquor. The results are shown in . Compared with the control group, treatment with EtE decreased the cough frequency at both doses of 100 and 200 mg/kg (p < 0.05), but significantly enhanced the latent period of cough only at the dose of 100 mg/kg. Treatment with the mixture of four compounds at doses of 10, 20, and 40 mg/kg decreased the number of cough by 73.7, 79.0, and 50.3% and enhanced the latent period of cough by 35.2, 80.3, and 54.9%, respectively. The results also revealed that the best dosage of the mixture for antitussive effects was 20 mg/kg among the three tested doses, which was further confirmed by the Bonferroni correction, a much stricter statistical method for post hoc tests ().
Figure 2. Effects of EtE and the mixture of compounds 1–4 on the ammonia-induced (A) latent period (s) and (B) frequency of cough (in 2 min) in mice. EtE was administered at 100 (L) and 200 (H) mg/kg, and the mixture of compounds 1–4 in the ratio as in EtE was given at 10 (L), 20 (M), and 40 (H) mg/kg. Pentoxyverine (17 mg/kg) was taken as a positive control, and the vehicle was administered with 0.5% CMC-Na. Values were expressed as mean ± SE (n = 9–10). Statistical significance was determined by Student's t-test, *p < 0.05, **p < 0.01, and ***p < 0.001, or by the Bonferroni correction (in red), #p < 0.05, ###p < 0.001, comparing with the vehicle.
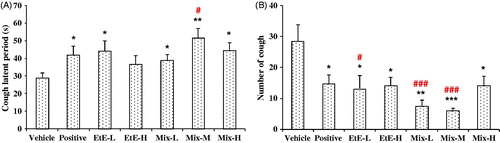
Antitussive effects of compounds 1–4
The antitussive effects of compounds 1–4, together with the mixture of compounds 1–4 in the ratio of 5:28:41:26, were evaluated with the model of ammonia liquor induced cough in mice and the results are shown in . Compared with the control, the mixture showed significantly activities of suppressing coughs by reducing cough frequency by 60.7% (10 mg/kg) and 61.7% (20 mg/kg) and increasing the latent period of cough by 203.6% (10 mg/kg) and 41.0% (20 mg/kg). Treatment with compounds 1–4 greatly inhibited the number of cough by 44.2% (1), 40.3% (2), 45.5% (3), and 58.0% (4) at 10 mg/kg and by 49.9% (1), 38.5% (2), 50.4% (3), and 52.0% (4) at 20 mg/kg. Treatment with compounds 1–4 also significantly enhanced the latent period of cough by 143.6% (1), 174.5% (2), 194.5% (3), and 152.7% (4) at 10 mg/kg and by 80.6% (1), 78.9% (2), 38.4% (3), and 49.6% (4) at 20 mg/kg. Although compound 4 (4,5-DCQA) showed the best antitussive activity, no significant difference was found among the four compounds. The results indicated that compounds 1–4 were responsible, at least partly, for the antitussive activities of FTF.
Figure 3. Effects of compounds 1–4 on the ammonia-induced (A) latent period (s) and (B) number of cough (in 2 min) in mice. Compounds 1–4 and their mixture in the ratio as in EtE were administered at 10 and 20 mg/kg, respectively. Pentoxyverine (17 mg/kg) was taken as a positive control, and the vehicle was administered with 0.5% CMC-Na. Values were expressed as mean ± SE (n = 10). Statistical significance was determined by Student's t-test, *p < 0.05, **p < 0.01, and ***p < 0.001, or by the Bonferroni correction (in red), #p < 0.05, ##p < 0.01, comparing with the corresponding vehicle.
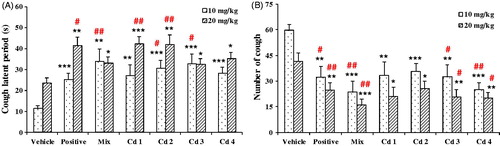
Expectorant effects of compounds 1–4
The expectorant activity of compounds 1–4, together with the mixture of compounds 1–4 in the ratio of 5:28:41:26, were tested with the model of phenol red secretion in mice and the results are shown in . Compared with the control, the mixture brought significant increase in phenol red secretion by 18.0 and 19.3% at doses of 10 and 20 mg/kg, respectively. All compounds could enhance the secretion of phenol red in mice in different degrees. Compound 2 (3,5-DCQA) expressed the most potent increase in the phenol red secretion by 29.7 and 35.7% at 10 and 20 mg/kg, respectively. Compound 3 (3,4-DCQA) showed moderate expectorant activities with the increase of 25.0 and 19.5% at doses of 10 and 20 mg/kg. Although compound 1 (CA) and compound 4 (4,5-DCQA) markedly increased phenol red secretion by 33.7 and 17.2% at 10 mg/kg, they had no effect at the dose of 20 mg/kg. Moreover, a multiple comparison by the Bonferroni correction revealed that the expectorant effect of compound 2 was significantly better than that of compound 4, at the dose of 20 mg/kg.
Figure 4. Effects of compounds 1–4 on the amount of phenol red secretion in mice. Compounds 1–4 and their mixture in the ratio as in EtE were administered at 10 and 20 mg/kg, respectively. Ammonium chloride (500 and 1000 mg/kg) was taken as a positive control, and the vehicle was administered with 0.5% CMC-Na. Values were expressed as mean ± SE (n = 9). Statistical significance was determined with Student's t-test, *p < 0.05, **p < 0.01, and ***p < 0.001, or by the Bonferroni correction (in red), #p < 0.05, ##p < 0.01, comparing with the corresponding vehicle.
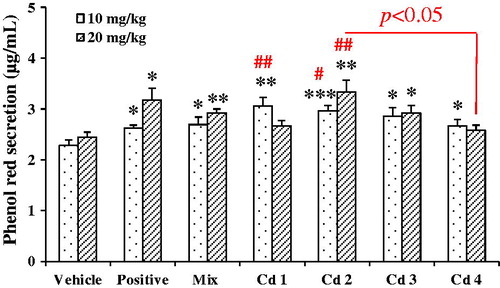
Anti-inflammation effects of compounds 1–4
Airway inflammation is an important feature of chronic cough, irrespective of its cause (Niimi, Citation2013). Therefore, the anti-inflammation effects of compounds 1–4, together with the mixture of compounds 1–4 in the ratio of 5:28:41:26, were evaluated with the model of acute airway inflammation induced by ammonia liquor, taking the number of leukocytes in BALF as the index. The results are shown in . Compared with the control, the leukocytes in BALF of the model mice were markedly increased, indicating the model was successful. Compared with the model, treatment with the mixture at 20 mg/kg observably decreased the number of leukocytes (p < 0.01). Similarly, treatment with compounds 1–4 at 20 mg/kg inhibited the leukocytosis by 20.5, 25.3, 39.0, and 49.7%. A multiple comparison by the Bonferroni correction exhibited that compound 4 (4,5-DCQA) was the most potent anti-inflammation substance. The BALF leukocytes in mice treated with compound 4 were significantly lower than those treated with compounds 1 (p < 0.001), 2 (p < 0.01), and even the vehicle (p < 0.05). It was indicated that compound 4 could strongly reduce leukocytes and may be somewhat harmful if leukocytes were lowered too much.
Figure 5. Effects of compounds 1–4 on the acute airway inflammation induced by ammonia liquor in mice, taking the number of leukocytes in BALF as an indicator. Compounds 1–4 and their mixture in the ratio as in EtE were administrated at 20 mg/kg, respectively. Prednisone acetate (10 mg/kg) was taken as a positive control, and the vehicle was administered with 0.5% CMC-Na. Values were expressed as mean ± SE (n = 9–10). Statistical significance was determined with Student's t-test, *p < 0.05, **p < 0.01, and ***p < 0.001, or by the Bonferroni correction (in red), #p < 0.05, ##p < 0.01, and ###p < 0.001, comparing with the model.
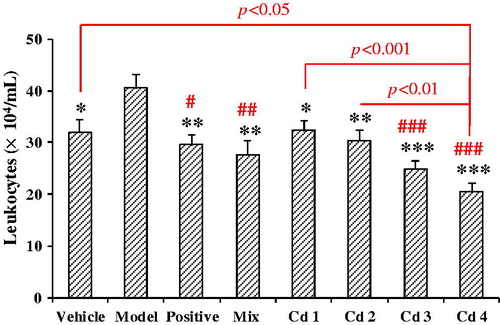
Discussion
Phytochemical researches revealed that FTF mainly contains sesquiterpenoids (tussilagones), phenolic acids (chlorogenic acids), flavonoids, and alkaloids (Liu et al., Citation2007), but the active constituents were still remained unknown. Our previous works showed that the total phenolic acids of FTF were the antitussive fraction (Wang, Citation2013). This study was to separate the main compounds from the active part, and to evaluate whether these compounds contribute to the anti-cough activity of FTF. As a result, four caffeoylquinic acids were isolated from EtE (the effective part) of FTF, and their antitussive, expectorant, and anti-inflammatory effects were tested for the first time. In order to control the false negative errors of the experiments, the Bonferroni correction, a more conservative method for multiple testing, was also employed for the statistical significance test (Genovese, Citation2015).
The antitussive experiments showed that the mixture of four caffeoylquinic acids at the given doses was stronger than anyone of compounds 1–4. After Bonferroni correction, compounds 3 and 4 showed obvious effects on the inhibition of cough frequency while compounds 1 and 2 showed obvious effects on the enhancement of cough latent period. However, the mixture of four compounds exhibited the largest inhibition in cough frequency. It suggested that caffeoylquinic acids in FTF may act in a synergistic way to relieve cough. To the best of our knowledge, the antitussive activities of caffeoylquinic acids have not been reported previously.
Chronic inflammation in the respiratory tract is one of the main reasons for causing cough (Jiang et al., Citation2014), and cysteinyl-leukotrienes secreted by leukocytes are crucial pro-inflammatory mediators involved in asthma and asthmatic cough (Niimi, Citation2013). The anti-inflammatory experiments showed that compounds 3 and 4 could significantly inhibited the ammonia liquid-induced leukocytosis, and the inhibition of compound 4 was significantly stronger than that of compounds 1 and 2 after the Bonferroni correction. Both anti-inflammatory and antitussive effects of compounds 3 and 4 were much better than those of compounds 1 and 2. It is suggested that the antitussive effects of caffeoylquinic acids may be mainly owing to their anti-inflammatory activity to inhibit leukocytosis, resulting in a down-regulation on the levels of cysteinyl-leukotrienes.
To date, no report has been found on the expectorant activity of caffeoylquinic acids. The expectorant experiments indicated that compounds 1 (only 10 mg/kg) and 2 (10 and 20 mg/kg) could increase the phenol red secretion while compounds 3 and 4 had no effects after the Bonferroni correction. These results were consistent with the effects of four compounds on the extension of the cough latent period, hinting that expectorant effects of caffeoylquinic acids may well help to prolong the cough latent period.
In a word, caffeoylquinic acids which are contained in FTF may be the main active ingredients acting in a synergistic way to relieve cough by suppressing airway inflammation and expelling sputum. However, the antitussive and anti-inflammatory efficacies were much stronger than the expectorant one. On one hand, such results were consistent with the traditional use of FTF being better at relieving cough than expelling sputum. On the other hand, such results also revealed that the anti-inflammatory effects of caffeoylquinic acids may play a crucial role for the antitussive activity of FTF. It is known that caffeoylquinic acids are of naturally occurring phenolic substances with antibacterial, antioxidant, antiviral, and hepatoprotective activities (Danino et al., Citation2009; Han et al., Citation2014; Kim et al., Citation2007; Li et al., Citation2005). But the antitussive mechanism of caffeoylquinic acids is elusive. A vitro study showed that CA (compound 1) significantly decreased LPS-induced up-regulation of COX-2 at protein and mRNA levels, thereby inhibiting PGE2 release in RAW 264.7 cells (Shan et al., Citation2009). Therefore, the antitussive activity of caffeoylquinic acids may be involved in inhibiting the release of inflammatory cytokines to mediate cough response, which remains to be further studied.
However, caffeoylquinic acids, especially dicaffeoylquinic acids, are difficult to separate and purify. Due to the limited amounts of compounds, this study adopted only one model and one species of animal to evaluate the antitussive, expectorant, and anti-inflammatory activities. Therefore, further experiments are necessary to confirm the anti-cough effects of these compounds with different models and different animals such as guinea pig.
Conclusion
It is concluded that caffeoylqunic acids are the major contributors for the antitussive effect of FTF. Moreover, this effect is a collective and synergistic response of compounds 1–4 which exert their action by inhibiting the airway inflammation, reducing sputum, or directly relieving cough. However, the mechanism of the action needs further investigation.
Declaration of interest
The authors report that they have no conflicts of interest.
Reference
- Chen J, Mangelinckx S, Ma L, et al. (2014). Caffeoylquinic acid derivatives isolated from the aerial parts of Gynura divaricata and their yeast α-glucosidase and PTP1B inhibitory activity. Fitoterapia 99:1–6.
- Chen Q. (1994). Methodology on Chinese Medicinal Pharmacology. Beijing: People's Medical Publishing House.
- Danino O, Gottlieb HE, Grossman S, et al. (2009). Antioxidant activity of 1,3-dicaffeoylquinic acid isolated from Inula viscosa. Food Res Int 42:1273–80.
- Dicpinigaitis PV. (2006). Current and future peripherally-acting antitussives. Respir Physiol Neurobiol 152:356–62.
- Genovese CR. (2015). False discovery rate control. Brain Mapp 1:501–7.
- Han J, Lv QY, Jin SY, et al. (2014). Comparison of anti-bacterial activity of three types of di-O-caffeoylquinic acids in Lonicera japonica flowers based on microcalorimetry. Chin J Nat Med 12:0108–13.
- Han N, Chang CL, Wang YC, et al. (2010). The in vivo expectorant and antitussive activity of extract and fractions from Reineckia carnea. J Ethnopharmacol 131:220–3.
- Jiang K, Song Q, Wang L, et al. (2014). Antitussive, expectorant and anti-inflammatory activities of different extracts from exocarpium Citri grandis. J Ethnopharmacol 156:97–101.
- Kamei J. (2002). Delta-opioid receptor antagonists as a new concept for central acting antitussive drugs. Pulm Pharmacol Ther 15:235–40.
- Kim KH, Kim YH, Lee RK. (2007). Isolation of quinic acid derivatives and flavonoids from the aerial parts of Lactuca indica L. and their hepatoprotective activity in vitro. Bioorg Med Chem Lett 17:6739–43.
- Li C, Huang F, Dou CG, et al. (2009a). Anti-inflammatory activity of Radix Asteris and Flos Farfarae in drug combination. Chin J Clin Pharmacol Ther 14:155–8.
- Li N, Ma SP, Huang F, et al. (2009b). Research on combination of the Radix Asteris with Flos Farfarae and the effect of Radix Asteris for expectorant. Chin J Clin Pharmacol Ther 14:159–62.
- Li Y, But PP, Ooi VE. (2005). Antiviral activity and mode of action of caffeoylquinic acids from Schefflera heptaphylla (L.) Frodin. Antivir Res 68:1–9.
- Li ZY, Zhi HJ, Zhang FS, et al. (2013). Metabolomic profiling of the antitussive and expectorant plant Tussilago farfara L. by nuclear magnetic resonance spectroscopy and multivariate data analysis. J Pharm Biomed 75:158–64.
- Liu YF, Yang XW, Wu B. (2007). Chemical constituents of the flower buds of Tussilago farfara. J Chin Pharm Sci 16:288–93.
- Mackenzie AJ, Spina D, Page CP. (2004). Models used in the development of antitussive drugs. Drug Discov Today 1:297–302.
- Nanjing University of Chinese Medicine. (2006). The Dictionary of Medicinal Plant (2nd ed). Shanghai: Shanghai Scientific and Technical Publishers.
- Niimi A. (2013). Cough, asthma, and cysteinyl-leukotrienes. Pulm Pharmacol Ther 26:514–19.
- Reynolds SM, Mackenzie AJ, Spina D, et al. (2004). The pharmacology of cough. Trends Pharmacol Sci 25:569–76.
- Shan JH, Fu J, Zhao ZH, et al. (2009). Chlorogenic acid inhibits lipopolysaccharide-induced cyclooxygenase-2 expression in RAW264.7 cells through suppressing NF-κB and JNK/AP-1 activation. Int Immunopharmacol 9:1042–8.
- Wang DD, Wang S, Chen X, et al. (2012). Antitussive, expectorant and anti-inflammatory activities of four alkaloids isolated from bulbus of Fritillaria wabuensis. J Ethnopharmacol 139:189–93.
- Wang L. (2013). Studies on the antitussive part of the flower buds of Tussilago farfara [Master’s thesis]. China Pharmaceutical University.
- Wei W, Wu XM, Li YJ. (2010). Methodology of Pharmacological Experiment (4th ed). Beijing: People's Medical Publishing House.
- Xue SY, Li ZY, Zhi HJ, et al. (2012). Metabolic fingerprinting investigation of Tussilago farfara L. by GC-MS and multivariate data analysis. Biochem Syst Ecol 41:6–12.
- Young EC, Smith JA. (2011). Pharmacologic therapy for cough. Curr Opin Pharmacol 11:224–30.
- Zhang QZ, Zhang Y, Zhang M, et al. (2009). Antitussive part of Flos Farfarae and potentiating part of Radix Asteris in pair-drug of FTF and RA. Lishizhen Med Mater Med Res 20:1042–4.