abstract
Context The underground edible tuber of Dioscorea alata L. (Dioscoreaceae) is a functional food with high nutritive value and therapeutic potential. The tuber is known to possess anti-inflammatory properties in traditional medicine.
Objective The present study explores the anti-inflammatory activity and standardisation of D. alata tuber hydromethanol extract.
Materials and methods Hydromethanol extract (70%) of D. alata tuber was chemically characterised using HPLC and GC-MS techniques. Murine lymphocytes were cultured for 48 h with six different concentrations (0–80 μg/mL) of the extract. The expression of nitric oxide (NO), TNF-α, COX-1, COX-2, and PGE2 were evaluated using colorimetric and ELISA methods.
Results Dioscorea alata extract inhibited the expression of NO and TNF-α with an IC50 value of 134.51 ± 6.75 and 113.30 ± 7.44 μg/mL, respectively. The IC50 values for inhibition of total COX, COX-1, COX-2 activities and PGE2 level were 41.96 ± 3.07, 141.41 ± 8.99, 32.50 ± 1.69, and 186.34 ± 15.36 μg/mL, respectively. Inhibition of PGE2 level and COX-2 activity was positively correlated (R2 = 0.9393). Gallic acid (GA), 4-hydroxy benzoic acid (4HBA), syringic acid (SYA), p-coumaric acid (PCA), and myricetin (MY) were identified and quantified using HPLC. GC-MS analysis revealed the presence of 13 different phytocompounds such as hexadecanoic acid, methyl stearate, cinnamyl cinnamate, and squalene.
Conclusion The D. alata extract significantly down-regulated the pro-inflammatory signals in a gradual manner compared with control (0 μg/mL). Different bioactive phytocompounds individually possessing anti-inflammatory activities contributed to the overall bioactivity of the D. alata tuber extract.
Introduction
The underground tuber of Dioscorea alata L. (Dioscoreaceae) is a staple food in south-eastern Asia and Africa (Akoruda Citation1984) and also well known in traditional medicine for its anti-inflammatory properties (Khare Citation2007). The tuber is extensively used as diuretic, aphrodisiac, anthelminitic and anti-diabetic in Indian traditional medicine (Maithili et al. Citation2011). Besides, the tubers of different yams such as D. alata are used in the treatment of inflammatory diseases in Chinese traditional medicine (Liu et al. Citation2012). Previous reports suggest that the tuber mucilage of D. alata stimulates antigen production and phagocytic activity; in addition, it enhances the cytotoxic activity of splenocytes (Shang et al. Citation2007). A bioactive hydro-methanol fraction of the tuber demonstrated stimulation of cell adhesion, phagocytic activity, plaque formation, and haemaglutination properties on murine cells (Dey et al. Citation2014). Previously we demonstrated that the hydro-methanol fraction modulates murine Th1/Th2 cytokine balance by up-regulating IFN-γ and IL-2 and down-regulating IL-4 and IL-10 levels on murine lymphocytes besides possessing excellent mitogenic activity (Dey & Chaudhuri Citation2014). Furthermore, another group of researchers demonstrated potent free radical scavenging activity of the same bioactive fraction of D. alata underground tuber (Das et al. Citation2012).
It is noteworthy that other yams of the same genus such as D. bulbifera L. (Mbiantcha et al. Citation2011), D. batatas Decne. (Jin et al. Citation2010), D. villosa (Lima et al. Citation2013), D. japonica Thunb. (Chiu et al. Citation2013) and D. trifida L.f. (Mollica et al. Citation2013) have already demonstrated anti-inflammatory activities. Different bioactive constituents were also identified in the tubers such as gallic acid and vanillic acid in D. japonica (Chiu et al. Citation2013), diosgenin and allantonin in D. trifida (Mollica et al. Citation2013), and diosgenin D. villosa (Lima et al. Citation2013). However, D. alata has never been evaluated for the same in spite of the fact that the tubers are consumed extensively and also has been claimed to possess anti-inflammatory properties in traditional medicine (Khare Citation2007). Moreover, the phytochemical profile of D. alata also remained unexplored. Therefore, we have studied the in vitro anti-inflammatory activity of D. alata underground tuber extract on murine splenic lymphocytes. Furthermore, the extract was chemically characterised by HPLC and GC-MS to reveal the bioactive constituents in the extract.
Materials and methods
Chemicals
Solvents and materials were obtained from HiMedia Laboratories Pvt. Ltd (Mumbai, India). Concanavalin A (Con A) was obtained from Sigma-Aldrich (St. Louis, MO). TNF-α ELISA kit was procured from RayBio (Norcross, GA), COX activity assay kit and PGE2 EIA kit were purchased from Cayman Chemical Company (An Arbor, MI). HPLC standards of gallic acid, p-coumaric acid (PCA), syringic acid, 4-hydroxybenzoic acid, 3,4-xylenol, p-cresol, o-cresol and jasmonic acid were procured form Sigma-Aldrich (St. Louis, MO) and rutin, quercetin, myricetin and ferulic acid were obtained from ChromaDex (Irvine, CA). Milli-Q® ultrapure water (Waters Copr, Milford, MA) from the departmental facility was used for all the experiments.
Plant material
Dioscorea alata tuber was collected during the month of April 2014, from the garden of Department of Zoology, University of North Bengal. The plant was identified by plant taxonomist Prof. A. P. Das of Department of Botany, University of North Bengal. A voucher specimen was stored at the herbarium of Department of Botany, University of North Bengal, with an accession number of 09719.
Extract preparation
The tuber was washed with water to remove dirt, then it was cut into pieces and shade-dried at room temperature for 20 d. Dry pieces were grinded to powder using a blender. The powder (100 g) was then mixed with 70% methanol (1000 mL) and kept at 37 °C in a shaking incubator (160 rpm) for 18 h. The mixture was centrifuged at 5000 rpm for 15 min. The supernatant liquid was separately stored. The remaining pellet was again mixed with 70% methanol (1000 mL) and kept in a shaking incubator as previously described and centrifuged. The supernatants from both the extraction phases were mixed and filtered using a vacuum filter. The filtrate was concentrated under reduced pressure in a rotary evaporator. The resultant was lyophilised and stored at −20 °C until further use.
Animals
Swiss albino mouse (30 ± 2 g) of 6–8 weeks of both sex were maintained under standard conditions in the animal house of the Department of Zoology, University of North Bengal, with food and water ad libitum. The experiments were approved by the ethical committee of University of North Bengal (No. 840/ac/04/CPCSEA) and performed in accordance with the legislation for the protection of animals used for scientific purposes.
Preparation of splenic lymphocytes
Swiss albino mice were sacrificed under proper anaesthesia and spleen was removed aseptically. The spleen was washed with water to remove any visual trace of blood. The cells were passed through the mesh of a tissue grinder and the cell suspension was prepared in RPMI-1640 medium. The cells were thrice washed with RPMI-1640 (1000 rpm, 10 min) and subjected to 1 mol/L NH4Cl to lyse the remaining red blood cells. After 5 min, ice cold RPMI-1640 was added to stop the reaction and centrifuged at 1000 rpm for 10 min. The cells were once again washed and resuspended in RPMI-1640.
Culture of lymphocytes
The resultant splenic lymphocytes were adjusted to 2 × 106 cells/mL with RPMI-1640 and seeded into 96 well plate (supplemented with 50 U/mL penicillin, 50 U/mL streptomycin, 50 U/mL nystatin and 10% FBS). Con A was added to get a final concentration of 5 μg/mL. Different concentrations (0–80 μg/mL) of D. alata extract were added and the plate was incubated at 37 °C for 48 h under 5% CO2 and humidified atmosphere of 90% air.
After incubation, culture supernatants from each well were centrifuged at 2850 × g for 5 min. The supernatants were used to assay NO, TNF-α and PGE2 levels and the cell pellets were used to determine COX activities.
Inhibition of nitric oxide
NO inhibitory capacity of D. alata was measured from the culture supernatant using the Griess reagent method (Hibbs et al. Citation1988) with slight modifications. In brief, culture supernatant (50 μL) was mixed with 200 μL of Griess reagent (1% sulphanilamide and 0.1% N-(1-naphthyl) ethylenediamine hydrochloride in 2.5% H3PO4) and incubated at 37 °C for 20 min. After the incubation, the absorbance was read at 540 nm. The percentage inhibition of NO was calculated using the following formula:
where A0 is the absorbance of the control and A1 is the absorbance in the presence of the sample.
Estimation of TNF-α
The levels of TNF-α in the culture supernatant were measured using RayBio ELISA kit (RayBio, Norcross, GA) according to the instructions of the manufacturer. Briefly, 100 μL culture supernatant from different experimental groups were added to the wells of the 96-well ELISA plate which was pre-coated with anti-mouse TNF-α. After 2.5 h of incubation at room temperature, the solutions were discarded and the wells were washed four times with assay buffer (300 μL). Next, 100 μL biotinylated anti-mouse TNF-α antibody was added to respective wells and the plate was incubated at room temperature with continuous but gentle shaking. After 1 h, the wells were washed with assay buffer as above. Then 100 μL of horseradish peroxidase (HRP)–streptavidin conjugate solution was added and the plate was incubated for 45 min at room temperature with gentle shaking. Next, the wells were washed as above and 100 μL 3,3′,5,5′-tetramethylbenzidine (TMB) one-step substrate reagent was added followed by 30 min incubation at room temperature in dark. Then 50 μL of 0.2 M sulphuric acid was added to each well to stop the reaction. The absorbance was immediately measured at 450 nm using Bio-Rad iMark™ microplate absorbance reader (Bio-Rad Laboratories, Hercules, CA). Standard was run in parallel to the samples.
Measurement of PGE2 level
PGE2 was measured by competition between PGE2 and PGE2-acetylcholineesterase (PGE2-AChE) for restricted amount of PGE2 monoclonal antibody using PGE2 EIA kit (Cayman, Ann Arbor, MI) according to the manufacturer’s instructions. In brief, 50 μL cell culture supernatant was added to the respective wells followed by 50 μL of PGE2-AChE tracer and 50 μL PGE2 monoclonal antibody. The plate was incubated 18 h at 4 °C and then washed five times with wash buffer. Ellman’s reagent (200 μL) was added and incubated in dark for 60 min with constant mild shaking. Then, the absorbance was read at 415 nm. Standard curve of PGE2 was prepared in parallel to the samples.
Measurement of COX activities
COX-1, COX-2, and total COX activities were measured using COX activity assay kit (Cayman Chemicals Company, Ann Arbor, MI) by measuring the peroxidase activity of COX through monitoring oxidised N,N,N′,N′-tetramethyl-p-phenylenediamine (TMPD) levels. The cell pellets after 48 h culture were homogenised in cold buffer consisting of 0.1 M Tris-HCL in 1 mM EDTA (pH 7.8). The homogenate was centrifuged (10 000 × g for 15 min) and the resultant supernatants were used for the assay. In brief, assay buffer, heme reagent, test samples, and background samples were added to their respective wells in the 96-well plate according to the instructions of manufacturer. DuP-697 (COX-2 inhibitor) and SC-560 (COX-1 inhibitor) were added (10 μL) to the respective inhibitor wells. The plate was incubated at 25 °C for 5 min and then, 20 μL TMPD was added to each well. Arachidonic acid (20 μL) was added to each well to start the reaction. The plate was incubated at 25 °C for 5 min and the absorbance was read at 590 nm. Ovine COX-1 was run as standard in parallel to the samples.
HPLC analysis
Dioscorea alata tuber extract was subjected to the Bligh and Dyer (Citation1959) method for removing the lipid contents. The methanol layer was separated and mixed with four volumes of chilled acetone and incubated for 60 min at −20 °C. The solution was then centrifuged at 15 000 × g for 15 min at 4 °C. The pellet containing protein was discarded and the supernatant was subjected to thin-layer chromatography (TLC) on a silica gel plate using 10% acetic acid in chloroform as a solvent. The corresponding bands of secondary metabolites were eluted by acetonitrile after detection with 20% w/v Na2CO3 and diluted Folin–Ciocaltaeu reagent (1:3). The solution was then analysed using HPLC (Agilent Technologies, Roseville, CA) having Zorbax SB-C18 column (4.6 × 150 mm, 3.5 μm) and equipped with Diod Array Detector. Gradient concentration of mobile phase A – methanol (M) and B – water (W) with 0.02% H3PO4 was 25% A + 75% B for 5 min, 30% A + 70% for 10 min, 45% A + 55% for 30 min, and 80% A + 20% B for 45 min. Injection volume was 20 μL and the flow rate was kept at 0.4 mL/min. Analytes were scanned in different wave lengths (254, 275, 280, and 320 nm). The peaks were identified by comparing the relative retention time (RRT) and spectral patterns with the standards of phenolic acids, flavonoids, and alcohols with a concentration of 0.05 mg/mL.
GC-MS analysis
GC-MS analysis of D. alata extract was performed using Agilent 5975C GC-MS system (Agilent Technologies, Roseville, CA) attached with HP-5 ms capillary column (30 m × 0.25 mm i.d. × 0.25 μm film thickness) and equipped with inert MSD triple axis mass detector conditioned at ion trap 200 °C, transfer line 280 °C, electron energy 70 eV (vacuum pressure – 2.21e – 0.5 Torr) was used for analysis. The flow rate of carrier gas (helium) was 1 mL/min. The sample (2 μL) was injected in a splitless mode. The column temperature was set at 60 °C for 1 min. followed by 5 °C/min up to 250 °C. The major and essential compounds in the extract were identified by their retention times and mass fragmentation patterns using Agilent Chem Station (Agilent Technologies, Roseville, CA) integrator and the database of National Institute Standard and Technology (NIST) with a MS library version 2010.
Data analysis and statistics
All experiments were performed in six replicates (n = 6) and data are reported as the mean ± SEM of six measurements. Comparisons between the control group and test groups were performed by one-way analysis of variance (ANOVA) test using KyPlot version 2.0 beta 15 (32 bit) for windows. p Value <0.05 was considered significant. Half-maximal inhibitory concentration (IC50) values were calculated using the formulae
where A1 = IC50; Y = response (Y = 100% when X = 0); X = inhibitory concentration.
The secondary metabolites in HPLC analysis were measured using the following formulae: response factor = and amount of the analyte =
. The concentration of the analytes was expressed in μg/mg as calculated from the dry weight (dw) of the extract initially measured for the extraction process.
Results
Inhibition of nitric oxide
demonstrated dose-dependent inhibition of NO by D. alata tuber extract. At 80 μg/mL, the extent of NO inhibition was 31.85 ± 0.77% compared with 0 μg/mL. The IC50 value was 134.51 ± 6.75 μg/mL.
Estimation of TNF-α
Significant (p < 0.001) down-regulation of TNF-α level (IC50 value = 113.30 ± 7.44 μg/mL) was evident in . At 0 μg/mL, the level of TNF-α was 399.66 ± 9.52 pg/mL, which was lowered to 251.66 ± 12.25 pg/mL at the highest dose. At 0 μg/mL, the amount of inhibition of TNF-α was 37.03% compared with control.
Measurement of PGE2 level
At 80 μg/mL the PGE2 level was significantly (p < 0.001) lowered to 497.13 ± 8.85 pg/mL from 649.3 ± 25.75 at 0 pg/mL (). This resulted in 23.43% lowering of PGE2 level at 80 μg/mL (IC50 value = 186.34 ± 15.36 μg/mL).
Measurement of COX activities
demonstrates the effect of D. alata extract on COX activities. At 0 μg/mL, the total COX, COX-1, and COX-2 activities were 23.47 ± 0.92, 10.46 ± 1.52, and 12.74 ± 0.57 U/mL, respectively, which were down-regulated to 9.95 ± 1.16, 6.57 ± 0.63, and 4.88 ± 0.42 U/mL, respectively, at 80 μg/mL. The IC50 values for total COX, COX-1, and COX-2 activities were 41.96 ± 3.07, 141.41 ± 8.99, and 32.50 ± 1.69 μg/mL. The amount of decrease in total COX, COX-1, and COX-2 activities were 57.60, 37.18, and 61.69%, respectively, compared with the 0 μg/mL group.
HPLC and GC-MS analysis
The HPLC chromatogram is demonstrated at . The presence of five phenolic acids namely GA (29.34 μg/g dw), 4HBA (6.48 μg/g dw), SA (2.94 μg/g dw), PCA (2.53 μg/g dw), and MY (42.39 μg/g dw) were detected in the tuber extract. GC-MS analysis () revealed the presence of 13 different metabolites which are enlisted in .
Figure 3. Demonstrates dose-dependent linear correlation of (A) TNF-α versus inhibition of nitric oxide; (B) TNF-α versus COX-2; (C) TNF-α versus PGE2; (D) COX-2 versus PGE2. Axes ‘x’ and ‘y’ denotes correlation points of respective parameters measured at different dose of D. alata extract (0–80 μg/mL). Where R2 = coefficient of determination.
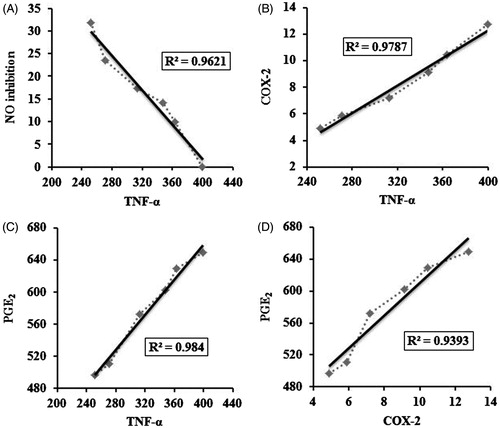
Figure 4. HPLC analysis of D. alata underground tuber extract. (1) gallic acid, (2) 4-hydroxy benzoic acid, (3) syringic acid, (4) p-coumaric acid, and (5) myricetin.
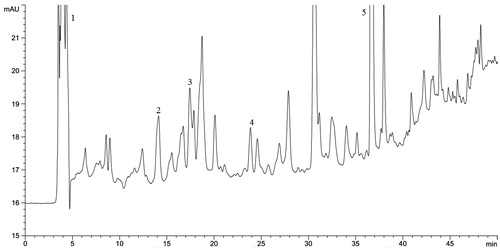
Figure 5. GC-MS analysis of D. alata underground tuber extract corresponding to . *Unidentified compound/repeat compound/column component.
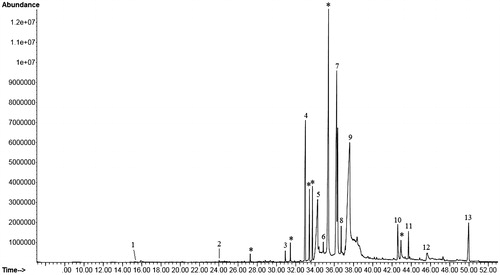
Table 1. Phytochemicals identified in D. alata underground tuber extract by GC-MS analysis corresponding to .
Discussion
Elimination of invading pathogens from the blood stream, as well as MHC expression and antigen presentation is mediated by splenic lymphocytes through a complex inter-connecting network of cytokines and chemokines (Mebius & Kraal Citation2005). Splenic lymphocytes predominantly regulate the pro-inflammatory conditions through cytokine production and modulation of immunocompetent cells (Semaeva et al. Citation2010). The plant lectin Con A is a typical T-lymphocyte mitogen responsible for blastoid differentiation of T-lymphocytes. Due to its lymphocyte activation property, Con A is widely used as a stimulator to study the anti-inflammatory properties in cultured lymphocytes.
NO and TNF-α are principal promoters, whereas COX and PGE2 are terminal mediators of the pathological conditions of chronic inflammatory response. In spite of being associated with anti-microbial activities, pro-inflammatory functions of NO eventually cause tissue damage. NO couples with superoxide radical to generate peroxynitrite which further stimulates the production of prostaglandins (Dey et al. Citation2014). Chronic release of NO and TNF-α is associated with various inflammatory conditions such as rheumatoid arthritis, multiple sclerosis, asthma, psoriasis, and Crohn’s disease (Kroncke et al. Citation1988; Cutler & Brombacher Citation2005). Cyclooxygenase (COX) is the principal molecule and key mediator of inflammation which catalyse the conversion of arachidonic acid to prostaglandins, eventually leading to the cardinal signs of inflammation like redness (increase in blood flow), swelling (vasodilation) and pain (induction of peripheral sensory neurons) (Ricciotti & Gerald Citation2011). Previously various plants of the yam family have demonstrated cyclooxygenase and prostaglandin inhibitory activities in different experimental models (Yang et al. Citation2009; Jin et al. Citation2010; Suzuki-Yamamoto et al. Citation2014).
Recently, it was shown (Dey & Chaudhuri Citation2014) that the same bioactive fraction of D. alata modulates murine immune response towards Th1 phenotype by up-regulating Th1 cytokines (IFN-γ and IL-2) and down-regulating Th2 cytokines (IL-4 and IL-10). The present NO inhibitory activity of D. alata corroborates the previous findings of Dey et al. (Citation2014) who demonstrated that potent NO inhibitory activity of D. alata on lipopolysaccharide stimulated murine peritoneal macrophages. Another group of researchers demonstrated that both the underground and areal tubers of D. alata inhibited free radical NO with an IC50 value of 223.75 ± 27.91 μg/mL and 209.42 ± 52.53 μg/mL, respectively. On the contrary, Liu et al. (Citation2007) have demonstrated that dioscorin, the chief storage protein of D. alata, stimulates the production of NO and TNF-α in LPS-induced RAW 264.7 cells. However, the present study showed that D. alata significantly (p < 0.001) inhibits both NO and TNF-α release from Con A-stimulated lymphocytes contributing to its anti-inflammatory activity. Furthermore, the anti-inflammatory activity of D. alata was further supported by the fact that COX and PGE2 levels were decreased by dose-dependent treatment of D. alata with high correlation (R2 = 0.9393) between COX-2 activity and PGE2 level ().
Dose-dependent univariate linear correlation analysis revealed high inter-correlation patterns between down-regulation of these pro-inflammatory mediators (). The inhibition of NO was not cell viability mediated because different studies have already confirmed that D. alata possess mitogenic activity and it has the ability to stimulate murine cellularity (Liu et al. Citation2007; Dey & Chaudhuri Citation2014; Dey et al. Citation2014). This may have resulted due to the inhibition of TNF-α level, which is a potent inducer of NO (Szabo Citation1995; Binder et al. Citation1999). This may be further supported by the fact that high correlation (R2 = 0.9621) resided between inhibition of NO and down-regulation of TNF-α level. At 80 μg/mL, the percentage inhibition of TNF-α was 37.03% compared with control. High correlation resided between TNF-α with COX-2 (R2 = 0.9787) activity and PGE2 (R2 = 0.984) levels. Previous studies also suggested that TNF-α induces COX-2 and PGE2 expression through transcription factors involved in inflammatory process (Chen et al. Citation2000; Nakao et al. Citation2002).
Phenolics are the primary mediators of bioactivities of herbal extracts. Aqueous-alcoholic solvent system is considered one of the best methods for isolation of total phenolic species from plant materials (Harborne Citation2001). In the traditional system, medicines are prepared mostly in polar solvents such as in water. Besides, many ethnopharmacological medicines are actually tinctures prepared by hydro-alcoholic solvent extraction process. The 70% hydro-methanol extract was previously demonstrated by other group of researchers to possess high amount of phenolic and flavonoid contents which contributed to its free radical scavenging activities (Das et al. Citation2012). The presence of gallic acid, syringic acid, PCA, and myricetin has been previously identified in other plants of the Dioscorea genus (Fritzemeier & Kindi Citation1984; Kindl Citation1985; Gao et al. Citation1997; Zuo Citation2002; Theerasin & Baker Citation2009; Chiu et al. Citation2013; Das et al. Citation2014). However, the present study for the first time () revealed the presence of different bioactive phenolics in the D. alata tuber. The anti-inflammatory activity of gallic acid was initially reported through paw edema model (Kroes et al. Citation1992). Later Kim et al. (Citation2006) reported potentials of gallic acid to down-regulate pro-inflammatory cytokines such as TNF-α and IL-6 in mast cells. The dietary phenol PCA increases immunoglobulin levels and decreases TNF-α levels in vivo (Pragasam et al. Citation2013), in addition, it inhibits thromboxane B2 production and LPS-induced PGE2 generation in vitro (Luceri et al. Citation2007). MY has demonstrated anti-inflammatory activity under xylene-induced ear edema, acetic acid-induced vascular permeability, carrageenan-induced paw edema, leukocyte migration, and cotton pellet granuloma models (Wang et al. Citation2010). Kim et al. (Citation2009) demonstrated that MY inhibits TNF-α-induced vascular endothelial growth factor expression by targeting MAPK kinase 4 (MKK4) and MEK1. Besides MY derivatives were demonstrated to inhibit some of the potent terminal mediators of inflammation such as cyclooxygenase, lipoxygenase, PGE2, PGI2, and PGD2 (Wang et al. Citation2010). 4HBA and SA are benzoic acid derivatives. Thierry et al. (Citation2012) demonstrated anti-oxidant and anti-inflammatory activities of different benzoic acid analogues on neutrophils. The phytochemicals identified by GC-MS also possess anti-inflammatory properties. Azulene derivatives inhibited NO production and expression of arachidonic acid pathway metabolites such as PGE2 in LPS-induced RAW 264.7 cells (Nishishiro et al. Citation2009). Aparna et al. (Citation2012) demonstrated through enzyme kinetics study that hexadecanoic acid competitively inhibits phospholipase A2, which is an initial trigger in the inflammatory process. Dietary linoleic acid (9,12-octadecadienoic acid) is an omega-6 fatty acid which acts as an anti-inflammatory nutrient or adjuvant (Kapoor & Huang Citation2006). Cinnamic acid derivatives are potent inhibitors of lipoxygenase (Hadjipavlou-Litina & Pontiki Citation2015) and demonstrated to possess anti-inflammatory activities through paw-edema model (Godoy et al. Citation2000). Besides the identified compounds are also active constituents of other medicinal plant which possess immunomodulatory properties.
Conclusion
The present study demonstrates that edible tuber of D. alata possesses potent anti-inflammatory activity. The results were further supported by phytochemical analysis which revealed the presence of different bioactive metabolites. Synergistic activities of these phytochemicals may be attributed to the anti-inflammatory activity of D. alata. Our future endeavour will be to study the effect of D. alata areal tuber on the murine immunocompetent cells.
Acknowledgements
The authors are grateful to Mr. Bijoy Mahanta for maintenance of the animals and Mr. Sandipan Ray for assistance during phytochemical analysis.
Declaration of interest
The authors report that they have no conflicts of interests. The authors are grateful to the Department of Biotechnology, Government of India, for financial assistance (Order no. BT/09/NE/TBP/2010).
References
- Akoruda MO. 1984. Genetic improvement of vegetable crops: yam (Dioscorea spp.). In: Kasloo M, editor. Genetic improvement of vegetable crops. New York: Pergamon Press. p. 717–733.
- Aparna V, Dileep KV, Mandal PK, Karthe P, Sadasivan C, Haridas M. 2012. Anti-inflammatory property of n-hexadecanoic acid: structural evidence and kinetic assessment. Chem Biol Drug Des. 80:434–439.
- Binder C, Schulz M, Hiddemann W, Oellerich M. 1999. Induction of inducible nitric oxide synthase is an essential part of tumor necrosis factor-alpha-induced apoptosis in MCF-7 and other epithelial tumor cells. Lab Invest. 79:1703–1712.
- Bligh EG, Dyer WJ. 1959. A rapid method for total lipid extraction and purification. Can J Biochem Phys. 37:911–917.
- Chen CC, Sun YT, Chen JJ, Chiu KT. 2000. TNF-alpha-induced cyclooxygenase-2 expression in human lung epithelial cells: involvement of the phospholipase C-gamma 2, protein kinase C-alpha, tyrosine kinase, NF-kappa B-inducing kinase, and I-kappa B kinase 1/2 pathway. J Immunol. 165:2719–2728.
- Chiu CS, Deng JS, Chang HY, Chen YC, Lee MM, Hou WC, Lee CY, Huang SS, Huang GJ. 2013. Antioxidant and anti-inflammatory properties of taiwanese yam (Dioscorea japonica Thunb. var. pseudojaponica (Hayata) Yamam.) and its reference compounds. Food Chem. 11:1087–1096.
- Cutler A, Brombacher F. 2005. Cytokine therapy. Ann N Y Acad Sci. 1056:16–29.
- Das A, Chaudhuri D, Ghate NB, Chatterjee A, Mandal N. 2014. Phytochemical analysis, antioxidant and anticancer potential of leaf extracts from edible greater yam, Dioscorea alata L., from north-east India. Int J Phytopharmacol. 5:109–119.
- Das A, Chaudhuri D, Mandal N, Chatterjee A. 2012. Study of antioxidant and reactive oxygen species scavenging activity of the edible tuber of “greater yam” (Dioscorea alata L.) from north-east India. Asian J Pharm Clin Res. 5:74–84.
- Dey P, Chaudhuri TK. 2014. In vitro modulation of TH1 and TH2 cytokine expression in mitogen induced splenic T-lymphocytes by the edible tubers of Dioscorea alata. Food Sci Hum Wellness. 3:1–8.
- Dey P, Roy S, Chaudhuri TK. 2014. Stimulation of murine immune response by the tubers of Dioscorea alata L. of north-eastern region of India. Proc Zool Soc. 67:140–148.
- Fritzemeier KH, Kindi H. 1984. Two different pathways leading to phenanthrenes and 9,10-dihydrophenanthrenes of the genus Dioscorea. Z Naturforsch. 39c:217–221.
- Gao H, Hou B, Kuroyanagi M, Wu L. 1997. Constituents from anti-tumor-promoting active part of Dioscorea bulbifera L. in JB6 mouse epidermal cells. Asian J Tradit Med. 2:104–109.
- Godoy ME, Rotelli A, Pelzer L, Tonn CE. 2000. Antiinflammatory activity of cinnamic acid esters. Molecules 5:547–548.
- Hadjipavlou-Litina D, Pontiki E. 2015. Aryl-acetic and cinnamic acids as lipoxygenase inhibitors with antioxidant, anti-inflammatory, and anticancer activity. Methods Mol Biol. 1208:361–377.
- Harborne JB. 2001. Phytochemical methods a guide to modern techniques of plant analysis. New York: Springer Science & Business Media.
- Hibbs JB, Taintor RR, Vavrin Z, Rachlin EM. 1988. Nitric oxide: a cytotoxic activated macrophage effector molecule. Biochem Biophys Res Commun. 157:87–94.
- Jin M, Suh SJ, Yang JH, Lu Y, Kim SJ, Kwon S, Jo TH, Kim JW, Park YI, Ahn GW, et al. 2010. Anti-inflammatory activity of bark of Dioscorea batatas DECNE through the inhibition of iNOS and COX-2 expressions in RAW 264.7 cells via NF-κB and ERK1/2 inactivation. Food Chem Toxicol. 48:3073–3079.
- Kapoor R, Huang YS. 2006. Gamma linolenic acid: an antiinflammatory omega-6 fatty acid. Curr Pharm Biotechnol. 7:531–534.
- Khare CP. 2007. Indian medicinal plants: an illustrated dictionary. New Delhi, India: Springer.
- Kim JE, Kwon JY, Lee DE, Kang NJ, Heo YS, Lee KW, Lee HJ. 2009. MKK4 is a novel target for the inhibition of tumor necrosis factor-alpha-induced vascular endothelial growth factor expression by myricetin. Biochem Pharmacol. 77:412–421.
- Kim SH, Jun CD, Suk K, Choi BJ, Lim H, Park S, Lee SH, Shin HY, Kim DK, Shin TY. 2006. Gallic acid inhibits histamine release and pro-inflammatory cytokine production in mast cells. Toxicol Sci. 91:123–131.
- Kindl H. 1985. Biosynthesis of stilbenes. In: Higuchi T, editor. Biosynthesis and biodegradation of wood components. Japan: Academic Press. p. 361–362.
- Kroes BH, van den Berg AJ, Quarles van Ufford HC, van Dijk H, Labadie RP. 1992. Anti-inflammatory activity of gallic acid. Planta Med. 58:499–504.
- Kroncke KD, Fehsel K, Kolb-Bachofen V. 1988. Inducible nitric oxide synthase in human diseases. Clin Exp Immunol. 113:147–156.
- Lima CM, Lima AK, Melo MG, Serafini MR, Oliveira DL, de Almeida EB, Barreto RS, Nogueira PC, Moraes VR, Oliveira ER, et al. 2013. Bioassay-guided evaluation of Dioscorea villosa-an acute and subchronic toxicity, antinociceptive and anti-inflammatory approach. BMC Complement Altern Med. 13:135.
- Liu SF, Chang SY, Lee TC, Chuang LY, Guh JY, Hung CY, Hung TJ, Hung YJ, Chen PY, Hsieh PF, et al. 2012. Dioscorea alata attenuates renal interstitial cellular fibrosis by regulating Smad-and epithelial-mesenchymal transition signaling pathways. PLoS One 7:e47482.
- Liu YW, Shang HF, Wang CK, Hsu FL, Hou WC. 2007. Immunomodulatory activity of dioscorin, the storage protein of yam (Dioscorea alata cv. Tainong No. 1) tuber. Food Chem Toxicol. 45:2312–2318.
- Luceri C, Giannini L, Lodovici M, Antonucci E, Abbate R, Masini E, Dolara P. 2007. p-Coumaric acid, a common dietary phenol, inhibits platelet activity in vitro and in vivo. Br J Nutr. 97:458–463.
- Maithili M, Dhanabal SP, Mahendran S, Vadivelan R. 2011. Antidiabetic activity of ethanolic extract of tubers of Dioscorea alata in alloxan induced diabetic rats. Indian J Pharmacol. 43:455–459.
- Mbiantcha M, Kamanyi A, Teponno RB, Tapondjou AL, Watcho P, Nguelefack TB. 2011. Analgesic and anti-inflammatory properties of extracts from the bulbils of Dioscorea bulbifera L. var sativa (Dioscoreaceae) in mice and rats. Evid Based Complement Alternat Med. 2011:912935.
- Mebius RE, Kraal G. 2005. Structure and function of the spleen. Nat Rev Immunol. 5:606–616.
- Mollica JQ, Cara DC, Auriol MD, Oliveira VB, Cesar IC, Brandao MGL. 2013. Anti-inflammatory activity of American yam Dioscorea trifida L.f. in food allergy induced by ovalbumin in mice. J Funct Food. 5:1975–1984.
- Nakao S, Ogtata Y, Shimizu E, Yamazaki M, Furuyama S, Sugiya H. 2002. Tumor necrosis factor alpha (TNF-alpha)-induced prostaglandin E2 release is mediated by the activation of cyclooxygenase-2 (COX-2) transcription via NFkappaB in human gingival fibroblasts. Mol Cell Biochem. 238:11–18.
- Nishishiro M, Kurihara T, Wakabayashi H, Sakagami H. 2009. Effect of tropolone, azulene and azulenequinone derivatives on prostaglandin E2 production by activated macrophage-like cells. Anticancer Res. 29:379–383.
- Pragasam SJ, Venkatesan V, Rasool M. 2013. Immunomodulatory and anti-inflammatory effect of p-coumaric acid, a common dietary polyphenol on experimental inflammation in rats. Inflammation 36:169–176.
- Ricciotti E, FitzGerald GA. 2011. Prostaglandins and inflammation. Arterioscler Thromb Vasc Biol. 31:986–1000.
- Semaeva E, Tenstad O, Skavland J, Enger M, Iversen PO, Gjertsen BT, Wiig H. 2010. Access to the spleen microenvironment through lymph shows local cytokine production, increased cell flux, and altered signaling of immune cells during lipopolysaccharide-induced acute inflammation. J Immunol. 184:4547–4556.
- Shang HF, Cheng HC, Linag HJ, Liu HY, Liu SY, Hou WC. 2007. Immunostimulatory activities of yam tuber mucilages. Bot Stud. 48:63–70.
- Suzuki-Yamamoto T, Tanaka S, Tsukayama I, Takafuji M, Hanada T, Arakawa T, Kawakami Y, Kimoto M, Takahashi Y. 2014. Dioscorea japonica extract down-regulates prostaglandin E2 synthetic pathway and induces apoptosis in lung cancer cells. J Clin Biochem Nutr. 55:162–167.
- Szabo C. 1995. Alterations in nitric oxide production in various forms of circulatory shock. New Horizons 3:2–32.
- Theerasin S, Baker AT. 2009. Analysis and identification of phenolic compounds in Dioscorea hispida Dennst. As J Food Ag-Ind. 2:547–560.
- Thierry F, Ange MM, Thierry R, Gianangelo G, Philippe N, Didier S. 2012. Antioxidant and anti-inflammatory like properties of benzoic acid analogs on the oxidant response of neutrophils: structure/redox potential relationship study. Free Radic Biol Med. 53(Suppl 1).
- Wang SJ, Tong Y, Lu S, Yang R, Liao X, Xu YF, Li X. 2010. Anti-inflammatory activity of myricetin isolated from Myrica rubra Sieb. et Zucc. Leaves. Planta Med. 76:1492–1496.
- Yang MH, Yoon KD, Chin YW, Park JH, Kim J. 2009. Phenolic compounds with radical scavenging and cyclooxygenase-2 (COX-2) inhibitory activities from Dioscorea opposita. Bioorg Med Chem. 17:2689–2694.
- Zuo GH. 2002. Studies on the chemical constituents and anti-tumor promoting activity of Dioscorea bulbifera L. and Chaenomeles sinensis (Thouin) Koehne [Ph.D. thesis]. Shenyang Pharmaceutical University; [cited 2015 Jul 6] Available from: http://www.dissertationtopic.net/doc/1571995.