Abstract
Context Hamelia patens Jacq. (Rubiaceae) is traditionally used to treat wounds, inflammation and diabetes. However, there is still a lack of scientific evidence to support these applications.
Objective The objective of this study is to evaluate the anti-inflammatory, antioxidant and antidiabetic activities of Hamelia patens, and identify its bioactive compounds.
Materials and methods Four extracts were obtained by maceration and liquid–liquid extraction: HEX, DCM–EtOAc, MeOH–EtOAc and MeOH–Aq. The anti-inflammatory effect was evaluated orally on rat paw carrageenan-induced oedema over 6 h (50, 200 and 500 mg/kg), and topically in mouse ear oedema induced by 12-tetradecanoylphorbol-13-acetate (TPA) after 4 h (0.5 and 1 mg/ear). We also evaluated myeloperoxidase levels in ear tissue, 2,2-diphenyl-1-picrylhydrazyl (DPPH) scavenging ability, and in vitro α-glucosidase inhibition. The chemical compounds were separated by column chromatography and identified by spectroscopic analysis.
Results We found that the oral administration of the HEX extract at 500 and 200 mg/kg significantly decreased the carrageenan-induced inflammation after 1 and 3 h, respectively. The MeOH–EtOAc extract significantly inhibited myeloperoxidase activity (83.5%), followed by the DCM–EtOAc extract (76%), β-sitosterol/stigmasterol (72.7%) and the HEX extract (55%), which significantly decreased oedema induced by TPA at both doses, giving a similar effect to indomethacin. We also found that the MeOH–EtOAc, MeOH–Aq and DCM–EtOAc extracts showed good DPPH scavenging activity (IC50 values of 18.6, 93.9 and 158.2 μg/mL, respectively). The HEX extract showed the lowest α-glucosidase inhibition (an IC50 value of 26.07 μg/mL), followed by the MeOH–EtOAc extract (an IC50 value of 30.18 μg/mL), β-sitosterol/stigmasterol (IC50 34.6 μg/mL) and compound A ((6E,10E,14E,18E)-2,6,10,14,18,23-hexamethyl-2,6,10,14,18,22-tetracosahexaene, an IC50 value of 114.6 μg/mL), which were isolated for the first time from Hamelia patens.
Discussion and conclusion Hamelia patens possesses anti-inflammatory, antioxidant and α-glucosidase inhibitory activities, which support its traditional use. These effects can be attributed to the identified compounds.
Introduction
Medicinal plants have been used for centuries to treat many diseases, and contain bioactive compounds with potentially curative effects. The scientific study of medicinal plants based on the ethnobotanical information has produced many useful drugs; however, scientific research validating the empirical use of medicinal plants is still scarce. Hamelia patens Jacq. (Rubiaceae), a shrub native to lowland neotropical areas, is traditionally used to treat inflammation of the uterus. The Zapotec people in Mexico use it to treat inflammatory dermatological conditions, and it is also applied topically to wounds to stop bleeding and to promote rapid healing. An infusion of its leaves is also used to treat diabetes (Heinrich Citation2000; Leonti et al. Citation2001; Andrade-Cetto & Heinrich Citation2005; Ahmad et al. Citation2012; Cruz & Andrade-Cetto Citation2015).
Previous research has already demonstrated some of the biological activities of H. patens. Extracts of its aerial parts showed good wound-healing properties in a double incision wound-healing bioassay in animals (Gomez-Beloz et al. Citation2003), and the topical application of leaf extracts decreased croton oil-induced oedema in mice ears (Sosa et al. Citation2002). However, experimental studies in other inflammation models are needed to evaluate the effects of oral administration of extracts from this plant.
Immune cells respond to foreign antigens in order to destroy them; however, when the inflammatory response is exacerbated and persistent, it can damage tissues. Inflammation is, therefore, commonly associated with the progression of many diseases, including diabetes. Diabetes mellitus, considered a global health problem, is characterized by high blood glucose levels. A useful treatment involves α-glucosidase inhibitors, which reduce the absorption of glucose after meals (Barreiro et al. Citation2010; Kumar et al. Citation2011). However, there are no reports of the biological activity of H. patens that validate its traditional use for the treatment of diabetes.
Phytochemical studies show that H. patens contains flavanone glycoside, narirutin, rosmarinic acid and several alkaloids such as pteropodine, isopteropodine, rumberine, palmirine, maruquine, alkaloid A, tetrahydroalstonine, aricine, hameline, uncarine, speciophylline and ephedrine (Ripperger Citation1977; Aquino et al. Citation1990; Chaudhuri & Thakur Citation1991; Reyes-Chilpa et al. Citation2004; Paniagua-Vega et al. Citation2012; Martins & Nunez Citation2015). Some of these alkaloids were associated with a relaxant effect on the contraction of rat myometrium induced by potassium chloride, but other active compounds associated with the alleged properties of this plant are still to be identified. Here we investigate the anti-inflammatory, antioxidant and antidiabetic effects of extracts from H. patens to validate its traditional use, and to separate and identify its chemical compounds.
Materials and methods
Chemicals
Activated carbon, silica gel (230–400 mesh, 60 Å), carrageenan, indomethacin (≥ 99%), 12-tetradecanoylphorbol-13-acetate (TPA), hexadecyltrimethylammonium bromide (HTAB), 3,3′,5,5′-tetramethylbenzidine (TMB), 2,2-diphenyl-1-picrylhydrazyl (DPPH), quercetin (≥ 98%), α-tocopherol (≥ 96%), α-glucosidase type I from Baker’s yeast, ρ-nitrophenol glucopyranoside and acarbose (≥ 95%) were purchased from Sigma-Aldrich Co. (St. Louis, MO). Plates precoated with silica gel 60 and 200 F245 were obtained from Macherey-Nagel (Düren, Germany).
Plant material
Leaves of Hamelia patens Jacq. (Rubiaceae) were collected in July 2012 from Ciudad Valles, Mexico. The plant was identified and authenticated by Alejandro Torres Montúfar of the Universidad Nacional Autónoma de México (UNAM), and a voucher specimen (1377324) was deposited at the National Herbarium MEXU (UNAM, México, D.F., México).
Extraction and fractionation
Air-dried leaves were powdered manually and an 800 g sample was subjected to sequential maceration in solvents of increasing polarity (hexane, dichloromethane and methanol; 3 × 5 L, 24 h each), resulting in a crude hexane extract (HEX, yield 22 g, 2.75% of the 800 g of leaves), a crude dichloromethane extract (yield 12 g, 1.5%) and a crude methanol extract (yield 125 g, 15.6%). Chlorophyll was removed with activated carbon, which was added to the hexane extract and separated by filtration. The hexane was evaporated under vacuum, and the concentrated extract (10 g) was fractionated by silica-gel flash chromatography (230–400 mesh, 60 Å, 49 × 600 mm) and eluted with increasing polarity of hexane (14 L), hexane/ethyl acetate 9.7:0.3 (18 L), 8:1 (8 L), 8:3 (5 L) and ethyl acetate (5 L). The eluates were analysed by TLC (silica gel 60 F245, hexane/ethyl acetate 8:1, ceric sulphate/H2SO4 solution). The fractions (200 mL) were grouped into 28 fractions. The dichloromethane and methanol extracts were diluted in water and filtered through celite to remove chlorophyll. The filtrate was partitioned with ethyl acetate to obtain an aqueous phase and an organic phase. Biological activity was evaluated in crude hexane extract (HEX), column fractions, the residue of the organic phase of the dichloromethane extract (DCM-EtOAc, yield 0.085%), the residue of the aqueous phase of the methanol extract that was extracted again with methanol (MeOH-Aq, yield 5.8%) and the residue of the organic phase of the methanol extract (MeOH-EtOAc, yield 3.2%) ().
Figure 1. Flow chart for the extraction, fractionation and compounds isolation from Hamelia patens. The leaves were subjected to sequential maceration with hexane, dichloromethane and methanol. Chlorophyll was removed with activated carbon or by filtration over celite. The hexane extract (HEX) without chlorophyll was fractionated by silica–gel column chromatography. Fractions 1 and 12 were purified by preparative plate chromatography, yielding two compounds. The dichloromethane and methanol extracts were subjected to liquid–liquid extraction with ethyl acetate (DCM–EtOAc and MeOH–EtOAc). The residue of the aqueous phase of the methanol extract was extracted again with methanol (MeOH–Aq).
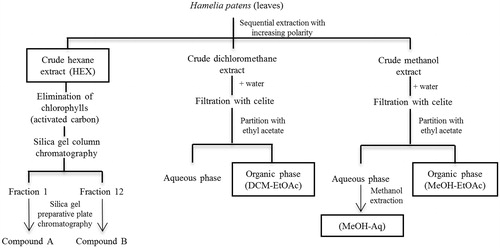
Isolation and structure elucidation
Fractions 1 and 12 (yield 1.9% and 4.3% respectively, with respect to HEX) were further purified by preparative layer chromatography on a plate precoated with silica gel 200 F245, and eluted with hexane and hexane/ethyl acetate 8:2, respectively. The structure elucidation of compound A (Rf: 0.43, yield 0.85% from HEX) and of compound B (Rf: 0.32, yield 1.47%) was performed by 1H and 13C nuclear magnetic resonance (NMR) (Bruker Avance III 400 MHz, Bruker Corporation, New Orleans, LA), infrared analysis (IR spectrophotometer Bruker Tensor 27, Bruker Corporation, New Orleans, LA) and mass spectrometry (Jeol, JMS AX-505 HA, Jeol Inc., Tokyo, Japan). The assignments were confirmed by DEPT, HMQC, HMBC and COSY-NMR experiments.
Experimental animals
Female Wistar rats (150–180 g) were provided by the School of Medicine, Universidad Autónoma de San Luis Potosí (UASLP), and CD1 mice (25–30 g) were provided by the Instituto de Fisiología Celular, UNAM. All animals were kept at a controlled temperature (22–25 °C), light/dark cycles (12 h) and with food and water ad libitum. The experiments were conducted in accordance with the Mexican guidelines for the production, care and use of laboratory animals (NOM-062-ZOO-1999) and the protocol of this study was approved by the local bioethics committee of the Unidad Académica Multidisciplinaria Zona Huasteca, UASLP (Acta 1-2014).
Carrageenan-induced paw oedema
Oral anti-inflammatory activity was performed as previously described (Winter et al. Citation1962). The basal volume (V0) of the right paw of the rats was measured with a plethysmometer (Ugo Basile, Monvalle, Italy) before the administration of the drugs, and samples were then immediately administered orally. After 1 h, inflammation was induced with carrageenan (1% in saline solution) by subcutaneous injection into the right hind paw (100 μL). Paw oedema volume (Vt) was measured 1, 2, 3, 4, 5 and 6 h after carrageenan injection. The results were expressed as variation of paw oedema volume (Vt − V0) in mL for each group (n = 6). Indomethacin was used as a non-steroidal anti-inflammatory (7.5 mg/kg). The control group received only the vehicle (oil or Tween-water).
TPA-induced ear oedema
Topical anti-inflammatory activity was performed as previously described (Young et al. Citation1983). CD1 mice were anesthetised with pentobarbital (n = 5) and inflammation was induced with TPA (2.5 μg). TPA and the substances under study were applied topically on both sides of the ear. The left ears of the mice were treated with only 20 μL of the corresponding vehicles (ethanol, chloroform, 1:1 methanol: dichloromethane and 1:1 acetone: water). After 4 h, the mice were sacrificed via CO2 asphyxiation and a biopsy (7 mm diameter) was taken from each ear. The control groups received only the corresponding vehicles. The ear oedema was measured by the weight difference between the right ear and the left ear.
Myeloperoxidase measurement
Myeloperoxidase activity was determined in ear biopsy samples 4 h after application of TPA, based on the method described previously (Bradley et al. Citation1982). Ear samples were homogenised in phosphate-buffered saline (PBS, 80 mM, pH 5.4) containing HTAB (0.5%). The homogenates were centrifuged at 12 000 rpm, 4 °C for 10 min. The following substances were added to each well of a 96-well plate at 37 °C: the supernatant (10 μL), PBS without HTAB (180 μL) and 0.0017% hydrogen peroxide (20 μL). The reaction was started with 20 μL of 18.4 mM TMB in dimethylformamide. The plate was incubated at 37 °C for 5 min with stirring, and the reaction was stopped with 20 μL of 2M H2SO4. The optical density was measured at 450 nm using a BioTek microplate reader Synergy HT (BioTek Instruments, Inc., Winooski, VT). Basal levels of myeloperoxidase activity were measured in the left ears. The percentage inhibition in each group was calculated using the formula: I% = (C − T/C) × 100, where C and T are the mean of optical density in the control and the treated group, respectively. The assay was performed in triplicate.
DPPH radical scavenging activity
Antioxidant activity was determined using DPPH, based on the method described previously (Brand-Williams et al. Citation1995). Samples (50 μL) at different concentrations were mixed with 150 μL DPPH (a final concentration of 100 μM). The reaction was incubated in the dark at 37 °C for 30 min and the optical density was then measured at 515 nm. Antioxidant activity was expressed as IC50 and compared with standard antioxidants such as quercetin and α-tocopherol. A DPPH solution without sample was used as the control. All curves were determined in triplicate.
α-Glucosidase inhibition
Briefly, 25 μL of samples at different concentrations were mixed with 150 μL of PBS, 25 μL of reduced glutathione and 25 μL of α-glucosidase (0.2 U/L from Saccharomyces cerevisiae). The reaction was then started with 25 μL of ρ-nitrophenyl glucopyranoside (23.2 mM), incubated at 37 °C for 15 min and stopped with 50 μL of 1 M Na2CO3. The optical density was measured at 405 nm. The inhibition of α-glucosidase was expressed as IC50 and compared with standard α-glucosidase inhibitors such as quercetin and acarbose. A reaction mixture with dimethyl sulphoxide solution (50%) without sample was used as the control (enzyme activity, 100%). All curves were determined in triplicate.
Statistical analysis
Statistical analysis was performed using the GraphPad Prism 5 statistical package (GraphPad Software, San Diego, CA). The results from the carrageenan-induced paw oedema were analysed by two-way ANOVA followed by Bonferroni’s post-test. The results from other experiments were analyzed by one-way ANOVA followed by Tukey’s post-test and Kruskal–Wallis with Dunn's multiple comparison test. p values <0.05 were considered significantly different.
(6E,10E,14E,18E)-2,6,10,14,18,23-Hexamethyl-2,6,10,14,18,22-tetracosahexaene
Colourless oil; IR (KBr) 2963, 2927, 2855, 1667, 1603 cm − 1; 1H-NMR (CDCl3, 400 MHz) δ 1.6 (18H, s, 6CH3, C-25, C-26, C-27, C-28, C-29, C-24), 1.68 (6H, s, 2CH3, C-1, C-30), 2.07 (10H, m, 5CH2, C-4, C-8, C-12, C-16, C-20), 2.01 (2H, m, CH2, C-21), 1.97 (8H, m, 4CH2, C-5, C-9, C-13, C-17), 5.15 (1H, bt, 1CH=, C-22), 5.12 (3H, bt, 3CH=, C-3, C-7, C-11), 5.09 (2H, bt, 2CH=, C-15, C-19); 13C-NMR (CDCl3, 100 MHz) δ 15.98 (CH3, C-25), 17.66 (CH3, C-26, C-27, C-28, C-29), 16.03 (CH3, C-30), 25.67 (CH3, C-1, C-24), 26.77 (CH2, C-4, C-12), 26.67 (CH2, C-8), 28.27 (CH2, C-16, C-20), 29.70 (CH2, C-21), 39.74 (CH2, C-5, C-9, C-13, C-17), 124.27 (CH, C-3, C-15, C-22), 124.31 (CH, C-7, C-11, C-19), 131.23 (C, C-2, C-23), 134.89 (C, C-6, C-14), 135.10 (C, C-10, C-18); EI MS (positive ion mode) M+ m/z 410.
Results and discussion
The four extracts obtained from H. patens leaves () were evaluated for oral and topical anti-inflammatory activities. Initially, we showed that injection of carrageenan into a rat paw, and the topical application of TPA to mouse ear increased oedema with time in the control group. Only the HEX extract significantly decreased the carrageenan-induced oedema after 1, 3, 4, 5 and 6 h at doses of 500 mg/kg, and between 3 and 6 h at 200 mg/kg, compared with the control (). In contrast, the HEX extract at 50 mg/kg, and the MeOH-Aq and MeOH–EtOAc extracts at low and high doses resulted in an oedema similar to that produced by the control (data not shown).
Figure 2. Effect of the HEX extract and its fractions from Hamelia patens on carrageenan-induced paw oedema. The oedema volume was measured in rats treated orally with: (A) vehicle, HEX-50, HEX-200 and HEX-500 (HEX extracts at 50, 200 and 500 mg/kg, respectively), indomethacin (7.5 mg/kg) and (B) fractions 1 and 12 obtained from the HEX extract. Values represent the mean ± SEM, n = 6, *p < 0.05, **p < 0.01, ***p < 0.001 significantly different compared with the vehicle control group (two-way ANOVA followed by Bonferroni’s post-test).
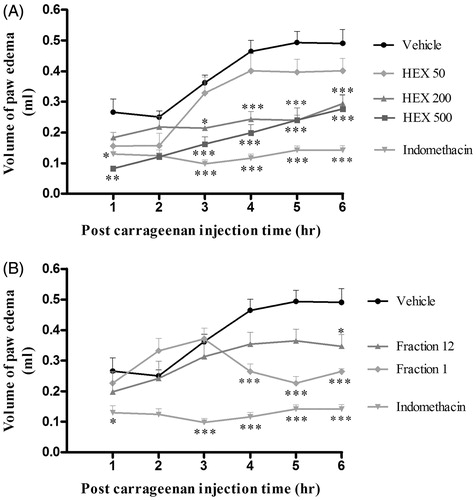
Unlike oral administration, the topical administration of each extract, except for the MeOH-Aq extract, significantly decreased the oedema induced by TPA (). In particular, the DCM–EtOAc and MeOH–EtOAc extracts showed a higher inhibition at the lowest dose (0.5 mg/ear), producing the same effect as indomethacin, which significantly decreased the oedema in both models. Similarly, Sosa et al. (Citation2002) found that the topical application of hexane, chloroform and methanol extracts from the leaves of H. patens had an anti-inflammatory effect against croton oil-induced ear oedema in mice; but no active compound or possible mechanisms were reported. Thus, we determined the myeloperoxidase activity in order to evaluate neutrophil infiltration in ear samples. Neutrophils are cells that contribute the most in an acute inflammatory response, releasing myeloperoxidase, an abundant enzyme that makes up 5% of their mass. Myeloperoxidase levels are a standard marker of neutrophil infiltration in tissue (Bradley et al. Citation1982). We found that the application of TPA to the ear increased myeloperoxidase activity compared with basal activity levels. The MeOH–Aq extract at 1 and 0.5 mg/ear showed similar myeloperoxidase inhibition to the control group, while the MeOH–EtOAc, DCM–EtOAc and HEX extracts at both doses, as well as indomethacin (71.5%), showed high myeloperoxidase inhibition (83.5, 76 and 55%, respectively) (), which corresponds to their anti-inflammatory activity in TPA-induced oedema.
Figure 3. Topical effect of the extracts from Hamelia patens at 1 and 0.5 mg/ear on (A) TPA-induced ear oedema (weight of biopsy) measured at 4 h and (B) percentage inhibition of myeloperoxidase. Hexane extract (HEX), organic phase of the dichloromethane extract (DCM–EtOAC), methanol extraction of the aqueous phase of the crude methanol extract (MeOH–Aq) and organic phase of the methanol extract (MeOH–EtOAc). Values represent the mean ± SEM, n = 5, **p < 0.01, ***p < 0.001 significantly different compared with control (TPA + vehicle), #p < 0.001 significantly different compared with the group at 1 mg/ear (one-way ANOVA followed by Tukey’s post-test).
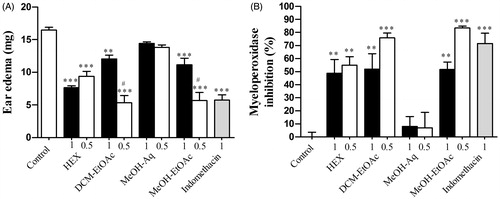
The carrageenan-induced oedema model is widely used to test and discover new anti-inflammatory drugs, as there is a significant correlation between the doses of non-steroidal anti-inflammatory drugs necessary to inhibit carrageenan-induced oedema in rats and the effective clinical doses used in humans (Otterness et al. Citation1979). Given that only the HEX extract showed an anti-inflammatory effect in this model, it was purified by column chromatography. Fraction 12 at 50 mg/kg significantly decreased the oedema only after 6 h, while fraction 1 at 50 mg/kg significantly decreased the oedema between 4 and 6 h (), which is an improvement on the effect of the HEX extract at the same doses. Thus, compounds from fractions 1 and 12 were separated by preparative chromatography.
We identified compound A as a triterpene, (6E,10E,14E,18E)-2,6,10,14,18,23-hexamethyl-2,6,10,14,18,22-tetracosahexaene, from fraction 1 (), and compound B as a mixture of β-sitosterol and stigmasterol from fraction 12. This is the first report of isolation and identification of these compounds from H. patens.
Figure 4. Chemical structure of (6E,10E,14E,18E)-2,6,10,14,18,23-hexamethyl-2,6,10,14,18,22-tetracosahexaene isolated from the HEX extract of Hamelia patens.

The 1H-NMR and 13C-NMR signals of compound A are similar to the sesquiterpene (tanacetene) reported by Mahmood et al. (Citation2002), but the mass spectrum indicates that compound A (M+ m/z 410) is a triterpene (tanacetene dimer) with six isoprene units, four of which are joined head to tail, and the fifth tail to tail. This compound is very similar to the triterpene squalene, which has six isoprene units, four of which are joined head to tail and the third tail to tail. Thus, compound A is an isomer of squalene. There are very few studies concerning (6E,10E,14E,18E)-2,6,10,14,18,23-hexamethyl-2,6,10,14,18,22-tetracosahexaene, and no biological activity has been reported before. We found that fraction 1 showed anti-inflammatory activity; therefore, compound A may contribute to this activity. Recent studies have demonstrated that squalene has anti-inflammatory activity both in vivo and in vitro, by inhibiting the nuclear factor kappa B and the production of pro-inflammatory cytokines (IL-1β and TNF-α). It also downregulated cyclooxygenase 2 and inducible nitric oxide synthase (Li et al. Citation2013; Sanchez-Fidalgo et al. Citation2015).
Compound B was identified to be a mixture of β-sitosterol and stigmasterol, and had a crystalline appearance. The 1H-NMR and 13C-NMR spectra were almost identical to the mixture of β-sitosterol and stigmasterol reported before (Hamdan et al. Citation2011). Previously, a short scientific note reported the presence of β-sitosterol in flowers and stems of H. patens (Subrahmanyam et al. Citation1973). Other similar compounds such as stigmast-4-ene-3-6-dione have also been identified in H. patens (Ripperger Citation1978). β-Sitosterol and stigmasterol are common plant sterols, and have been widely studied over the last 15 years. Several studies show that these phytosterols have anti-inflammatory activity in carrageenan and TPA models, as well as reducing myeloperoxidase activity and pro-inflammatory cytokines (IL-1β, IL-6 and TNF-α) (Navarro et al. Citation2001; Ammar et al. Citation2009; Nirmal et al. Citation2012; Hernandez-Valle et al. Citation2014; Ju et al. Citation2015). We found that compound B significantly decreased inflammation and myeloperoxidase levels induced by TPA, with high percentage inhibition, which is very similar to previous reports (). Thus, β-sitosterol and stigmasterol may contribute to the anti-inflammatory effect of the HEX extract.
Table 1. Topical effect of β-sitosterol and stigmasterol on TPA-induced ear oedema and myeloperoxidase inhibition.
The use of antioxidants has been associated with beneficial effects for the prevention and treatment of various diseases associated with oxidative stress (Rajendran et al. Citation2014). In this context, we determined antioxidant activity in H. patens by estimating IC50 values of DPPH radical scavenging activity for all samples. The HEX extract, its fractions, compound A and β-sitosterol/stigmasterol showed extremely low free radical scavenging activity. However, the MeOH–EtOAc extract exhibited potent activity, similar to the antioxidant α-tocopherol. The MeOH–Aq and DCM–EtOAc extracts also showed potential antioxidant activity, although the IC50 values were higher than those of α-tocopherol (). Thus, the extracts obtained with polar solvents (MeOH–EtOAc and MeOH–Aq) exhibited more antioxidant activity than the extracts obtained with solvents of medium or low polarity (DCM–EtOAc, HEX), a result similar to that observed in others studies (Ramos et al. Citation2003; Ruiz-Terán et al. Citation2008; Kumar et al. Citation2010).
Table 2. DPPH radical scavenging activity and α-glucosidase inhibition of extracts and fractions from H. patens.
The antioxidant activity in the polar extracts may be due to the presence of other compounds previously identified in H. patens, such as sesterpenes, triterpenes and polyphenols, which have been reported in methanol and acetone extracts (Aquino et al. Citation1990; Rios & Aguilar-Guadarrama Citation2006; Ruiz-Terán et al. Citation2008; Kumar et al. Citation2010). Some of these compounds have been evaluated in other studies, for example, catechin (a flavanol) decreases the production of nitric oxide, reactive oxygen species and several pro-inflammatory mediators in vitro, and ursolic acid (a pentacyclic triterpene) has various immunomodulatory properties, and strongly reduces the DPPH radical (Do Nascimento et al. Citation2014; Syed Hussein et al. Citation2015; Xu et al. Citation2015). Therefore, these bioactive compounds may be responsible for the antioxidant and anti-inflammatory activities of the DCM–EtOAc, MeOH–Aq and MeOH–EtOAc extracts. However, further studies are necessary to characterise the polar extracts and to identify the compounds responsible for their bioactivity.
Several studies show that polyphenols exhibit both antioxidant and anti-inflammatory activities (Calixto et al. Citation2003). We found that the MeOH–EtOAc extract showed the highest antioxidant activity, together with high anti-inflammatory activity (TPA model). Thus, the antioxidant activity of polar extracts could contribute to their anti-inflammatory effects, as oxidative stress is closely linked to inflammation. Reactive oxygen species participate in the onset of the inflammatory response because they can activate transcription factors such as the nuclear factor-kappa B, which mediates the gene expression of inflammatory mediators (Morgan & Liu Citation2011; Korbecki et al. Citation2013).
We evaluated the in vitro inhibition of α-glucosidase to validate the traditional use of H. patens in the treatment of diabetes. Patients with non-insulin-dependent diabetes mellitus use α-glucosidase inhibitors, as they delay carbohydrate digestion and absorption, reducing postprandial hyperglycaemia (Kumar et al. Citation2011). We found that the HEX extract showed the highest α-glucosidase inhibition, followed by the MeOH–EtOAc extract, β-sitosterol/stigmasterol, fraction 12, fraction 1 and compound A. In contrast, the DCM–EtOAc and MeOH–Aq extracts were inactive. Quercetin (positive control) is an effective α-glucosidase inhibitor, but acarbose (positive control) gave a very high IC50 value, perhaps because it works better against mammalian α-glucosidase, whereas we used yeast α-glucosidase (). Previous studies reported a hypoglycaemic effect of β-sitosterol and stigmasterol isolated from Dillenia indica L. (Dilleniaceae) (Kumar et al. Citation2013); but the antidiabetic effect of compound A was evaluated here for the first time. Compounds A and B showed good α-glucosidase inhibition, but did not achieve the IC50 value of the HEX extract. This demonstrates that they may act synergistically to achieve the observed antidiabetic effect of the HEX extract. This is the first study concerning inhibitory activity against α-glucosidase in H. patens, demonstrating that it may be a source of hypoglycaemic agents, which could have beneficial effects for the treatment of diabetes.
Conclusion
Hamelia patens possesses anti-inflammatory, antioxidant and α-glucosidase inhibitory activities, a result that validates its traditional use for the treatment of inflammation and diabetes. These effects can be attributed to the presence of (6E,10E,14E,18E)-2,6,10,14,18,23-hexamethyl-2,6,10,14,18,22-tetracosahexaene, β-sitosterol and stigmasterol, which were isolated for the first time from H. patens, and exhibited significant antidiabetic activity. The results suggest that H. patens is a source of antidiabetic and anti-inflammatory agents, but further investigations are necessary to elucidate their mechanisms of action.
Acknowledgements
Thanks are due to Héctor Ríos Olivares, Elizabeth Huerta Salazar, María Del Rocío Patiño Maya and Francisco Javier Pérez Flores for their collaboration in spectroscopic analysis.
Declaration of interest
The authors report that they have no conflicts of interest. This work was supported by the grants PROMEP 3597. CONACYT provided the fellowship 208981 to Jiménez-Suárez V.
References
- Ahmad A, Pandurangan A, Singh N, Ananad P. 2012. A mini review on chemistry and biology of Hamelia Patens (Rubiaceae). Pharmacogn J. 4:1–4.
- Ammar S, Edziri H, Mahjoub MA, Chatter R, Bouraoui A, Mighri Z. 2009. Spasmolytic and anti-inflammatory effects of constituents from Hertia cheirifolia. Phytomedicine 16:1156–1161.
- Andrade-Cetto A, Heinrich M. 2005. Mexican plants with hypoglycaemic effect used in the treatment of diabetes. J Ethnopharmacol. 99:325–348.
- Aquino R, Ciavatta ML, De Simone F, Pizza C. 1990. A flavanone glycoside from Hamelia patens. Phytochemistry 29:2359–2360.
- Barreiro O, Martin P, Gonzalez-Amaro R, Sanchez-Madrid F. 2010. Molecular cues guiding inflammatory responses. Cardiovasc Res. 86:174–182.
- Bradley PP, Priebat DA, Christensen RD, Rothstein G. 1982. Measurement of cutaneous inflammation: estimation of neutrophil content with an enzyme marker. J Invest Dermatol. 78:206–209.
- Brand-Williams W, Cuvelier ME, Berset C. 1995. Use of a free radical method to evaluate antioxidant activity. Food Sci Technol-Leb. 28:25–30.
- Calixto JB, Otuki MF, Santos AR. 2003. Anti-inflammatory compounds of plant origin. Part I. Action on arachidonic acid pathway, nitric oxide and nuclear factor kappa B (NF-kappaB). Planta Med. 69:973–983.
- Cruz EC, Andrade-Cetto A. 2015. Ethnopharmacological field study of the plants used to treat type 2 diabetes among the Cakchiquels in Guatemala. J Ethnopharmacol. 159:238–244.
- Chaudhuri PK, Thakur RS. 1991. Hamelia patens: a new source of ephedrine1. Planta Med. 57:199.
- Do Nascimento PG, Lemos TL, Bizerra AM, Arriaga AM, Ferreira DA, Santiago GM, Braz-Filho R, Costa JG. 2014. Antibacterial and antioxidant activities of ursolic acid and derivatives. Molecules 19:1317–1327.
- Gomez-Beloz A, Rucinski JC, Balick MJ, Tipton C. 2003. Double incision wound healing bioassay using Hamelia patens from El Salvador. J Ethnopharmacol. 88:169–173.
- Hamdan D, El-Readi MZ, Tahrani A, Herrmann F, Kaufmann D, Farrag N, El-Shazly A, Wink M. 2011. Chemical composition and biological activity of Citrus jambhiri Lush. Food Chem. 127:394–403.
- Heinrich M. 2000. Ethnobotany and its role in drug development. Phytother Res. 14:479–488.
- Hernandez-Valle E, Herrera-Ruiz M, Salgado GR, Zamilpa A, Ocampo ML, Aparicio AJ, Tortoriello J, Jimenez-Ferrer E. 2014. Anti-inflammatory effect of 3-O-[(6′-O-palmitoyl)-beta-d-glucopyranosyl sitosterol] from Agave angustifolia on ear edema in mice. Molecules 19:15624–15637.
- Ju A, Cho YC, Cho S. 2015. Methanol extracts of Xanthium sibiricum roots inhibit inflammatory responses via the inhibition of nuclear factor-kappaB (NF-kappaB) and signal transducer and activator of transcription 3 (STAT3) in murine macrophages. J Ethnopharmacol 174:74–81.
- Korbecki J, Baranowska-Bosiacka I, Gutowska I, Chlubek D. 2013. The effect of reactive oxygen species on the synthesis of prostanoids from arachidonic acid. J Physiol Pharmacol. 64:409–421.
- Kumar A, Kaur R, Arora S. 2010. Free radical scavenging potential of some Indian medicinal plants. J Med Plants Res. 4:2034–2042.
- Kumar S, Kumar V, Prakash O. 2013. Enzymes inhibition and antidiabetic effect of isolated constituents from Dillenia indica. Biomed Res Int. 2013:382063.
- Kumar S, Narwal S, Kumar V, Prakash O. 2011. α-Glucosidase inhibitors from plants: a natural approach to treat diabetes. Pharmacogn Rev. 5:19–29.
- Leonti M, Vibrans H, Sticher O, Heinrich M. 2001. Ethnopharmacology of the Popoluca, Mexico: an evaluation. J Pharm Pharmacol. 53:1653–1669.
- Li C, Xi F, Mi J, Wu Z, Chen W. 2013. Two new 3,4;9,10-seco-cycloartane type triterpenoids from Illicium difengpi and their anti-inflammatory activities. Evid Based Complement Alternat Med. 2013:942541.
- Mahmood U, Kaul VK, Singh B. 2002. Sesquiterpene and long chain ester from Tanacetum longifolium. Phytochemistry 61:913–917.
- Martins D, Nunez CV. 2015. Secondary metabolites from Rubiaceae species. Molecules 20:13422–13495.
- Morgan MJ, Liu Z-G. 2011. Crosstalk of reactive oxygen species and NF-κB signaling. Cell Res. 21:103–115.
- Navarro A, Delas Heras B, Villar A. 2001. Anti-inflammatory and immunomodulating properties of a sterol fraction from Sideritis foetens Clem. Biol Pharm Bull. 24:470–473.
- Nirmal SA, Pal SC, Mandal SC, Patil AN. 2012. Analgesic and anti-inflammatory activity of β-sitosterol isolated from Nyctanthes arbortristis leaves. Inflammopharmacology 20:219–224.
- Otterness IG, Wiseman EH, Gans DJ. 1979. A comparison of the carrageenan edema test and ultraviolet light-induced erythema test as predictors of the clinical dose in rheumatoid arthritis. Agents Actions 9:177–183.
- Paniagua-Vega D, Cerda-Garcia-Rojas CM, Ponce-Noyola T, Ramos-Valdivia AC. 2012. A new monoterpenoid oxindole alkaloid from Hamelia patens micropropagated plantlets. Nat Prod Commun. 7:1441–1444.
- Rajendran P, Nandakumar N, Rengarajan T, Palaniswami R, Gnanadhas EN, Lakshminarasaiah U, Gopas J, Nishigaki I. 2014. Antioxidants and human diseases. Clin Chim Acta. 436:332–347.
- Ramos A, Visozo A, Piloto J, Garcia A, Rodriguez CA, Rivero R. 2003. Screening of antimutagenicity via antioxidant activity in Cuban medicinal plants. J Ethnopharmacol. 87:241–246.
- Reyes-Chilpa R, Rivera J, Oropeza M, Mendoza P, Amekraz B, Jankowski C, Campos M. 2004. Methanol extracts of Hamelia patens containing oxindole alkaloids relax KCl-induced contraction in rat myometrium. Biol Pharm Bull. 27:1617–1620.
- Rios MY, Aguilar-Guadarrama AB. 2006. Indolic alkaloids, terpenes, sterols and flavonoids from the leaves of Hamelia patens Jacquin (Rubiaceae). Rev Cubana Plant Med. 11:1–4.
- Ripperger H. 1977. Isolation of isopteropodin from Hamelia patens. Pharmazie 32:415–416.
- Ripperger H. 1978. Isolation of stigmast-4-ene-3,6-dione from Hamelia patens and Clitoria ternatea. Pharmazie 33:82–83.
- Ruiz-Terán F, Medrano-Martínez A, Navarro-Ocaña A. 2008. Antioxidant and free radical scavenging activities of plant extracts used in traditional medicine in Mexico. Afr J Biotechnol. 7:1886–1893.
- Sanchez-Fidalgo S, Villegas I, Rosillo MA, Aparicio-Soto M, De la Lastra CA. 2015. Dietary squalene supplementation improves DSS-induced acute colitis by downregulating p38 MAPK and NFκB signaling pathways. Mol Nutr Food Res. 59:284–292.
- Sosa S, Balick MJ, Arvigo R, Esposito RG, Pizza C, Altinier G, Tubaro A. 2002. Screening of the topical anti-inflammatory activity of some Central American plants. J Ethnopharmacol. 81:211–215.
- Subrahmanyam K, Rao J, Rao K. 1973. Short scientific notes: chemical examination of Hamelia patens (Rubiaceae). Curr Sci. 42:841.
- Syed Hussein SS, Alfarizal Kamarudin MN, Kadir HA. 2015. (+)-Catechin attenuates NF-kappaB activation through regulation of Akt, MAPK, and AMPK signaling pathways in LPS-induced BV-2 microglial cells. Am J Chin Med. 43:927–952.
- Winter CA, Risley EA, Nuss GW. 1962. Carrageenin-induced edema in hind paw of the rat as an assay for antiiflammatory drugs. Proc Soc Exp Biol Med. 111:544–547.
- Xu H, Zhang M, Li XL, Li H, Yue LT, Zhang XX, Wang CC, Wang S, Duan RS. 2015. Low and high doses of ursolic acid ameliorate experimental autoimmune myasthenia gravis through different pathways. J Neuroimmunol. 281:61–67.
- Young JM, Wagner BM, Spires DA. 1983. Tachyphylaxis in 12-O-tetradecanoylphorbol acetate-and arachidonic acid-induced ear edema. J Invest Dermatol. 80:48–52.