Abstract
Context Catharanthus roseus (L.) G. Don (Apocynaceae) is still one of the most important sources of terpene indole alkaloids including anticancer and hypertensive drugs as vincristine and vinblastine. These final compounds have complex pathway and many enzymes are involved in their biosynthesis. Indeed, ajmalicine and catharanthine are important precursors their increase can lead to enhance levels of molecules of interest.
Objective This study aims at selecting the highest yield of hairy root line(s) and at identifying best times for further treatments. We study kinetics growth and alkaloids (ajmalicine and catharanthine) accumulation of three selected hairy root lines during the culture cycle in order to determine the relationship between biomass production and alkaloids accumulation.
Materials and methods Comparative analysis has been carried out on three selected lines of Catharanthus roseus hairy roots (LP10, LP21 and L54) for their kinetics of growth and the accumulation of ajamalicine and catharanthine, throughout a 35-day culture cycle. The methanolic extract for each line in different times during culture cycle is analyzed using liquid chromatography coupled to tandem mass spectrometry (LC-MS/MS).
Results Maximum accumulation of the alkaloids is recorded for LP10 line in which the peak of ajmalicine and catharanthine accumulation reached to 3.8 and 4.3 mg/g dry weight (DW), respectively. This increase coincides with an exponential growth phase.
Discussion and conclusion Our results suggest that the evolution of accumulation of ajmalicine and catharanthine are positively correlated with the development of the biomass growth. Significantly, for LP10 line the most promising line to continue optimizing the production of TIAs. Additionally, the end of exponential phase remains the best period for elicitor stimuli.
Introduction
Catharanthus roseus (L.) G. Don (Apocynaceae) is an important medicinal and ornamental plant. Its therapeutic benefit (value) is due to the presence of terpenoid indole alkaloids (TIAs), such as antihypertensive, ajmalicine and serpentine, and antitumoural alkaloids, vincristine and vinblastine, which have been used in chemotherapy since 1960s (van Der Heijden et al. Citation2004; Fernández-Pérez et al. Citation2013). The great pharmacological importance of vinblastine and vincristine is related with their low availability in C. roseus. A total of 1000 kg of dry leaves is required to produce 1 g of both of these alkaloids (Noble Citation1990). This presents a serious challenge to improve the performance and reduce the unit cost. Recently, it has been shown that vinblastine and vincristine can be semi-synthesized by using catharanthine and vindoline as precursors (Ishikawa et al. Citation2009).
For several years, plant tissue culture has been considered as a promising biotechnological approach to improve the secondary metabolites production (Malik et al. Citation2011, Citation2014). Organized cultures, especially transgenic hairy root cultures induced by Agrobacterium rhizogenes, represent a reliable alternative to undifferentiated tissue cultures, such as cell suspensions, for the production of valuable secondary metabolites (Mujib et al. Citation2012; Malik et al. Citation2016). The greatest advantage of hairy roots lies in their genotypic and biochemical stability as well as fast growth in hormone-free medium (Baíza et al. Citation1999; Giri & Narasu Citation2000; Georgiev et al. Citation2007; Makhzoum et al. Citation2013). In C. roseus, hairy roots have been reported to produce a high level of monoterpenoid indole alkaloids, mainly catharanthine, serpentine and ajmalicine (Vázquez-Flota et al. Citation1994; Moreno-Valenzuela et al. Citation2003; Batra et al. Citation2004). However, there is a lack of vindoline due to the absence of chloroplasts in the roots. Hence, neither vincristine nor vinblastin can be found in the roots of C. roseus (Ferreres et al. Citation2010).
In this paper, we report the induction and the establishment of different hairy root lines in C. roseus by using A. rhizogenes. Subsequently, the growth kinetics and accumulation of two monoterpenoid indole alkaloids (ajmalicine and catharanthine) of the three selected hairy root lines were measured. The objective was to determine the relationship between biomass production and alkaloids accumulation for choosing the high-performance line. In addition, our work also helps in identifying the ideal time to apply the elicitors’ treatment in the following steps of our research.
Materials and methods
Plant materials
Two cultivars of C. roseus (Cooler lilac and Cooler blush) were selected for genetic transformation assays. To establish aseptic cultures, seeds were disinfected with ethanol (75%, w/v) for 30 s, followed by a treatment with sodium hypochlorite (12%, w/v) for 10 min. The disinfected seeds were then rinsed three times with sterile distilled water before inoculating in test tubes containing 20 mL of Murashige and Skoog (MS) medium (Murashige & Skoog Citation1962) supplemented with 30 g/L sucrose and 5.5 g/L agar. Test tubes were kept in dark for 5 d at 25 °C and then the etiolated seedlings were exposed to continuous light for 4 d. The 9-d old seedlings were placed on MS medium supplemented with 2 mg/L benzyl amino purine (BAP). Cultures are incubated at 25 ± 2 °C under a 16 h photoperiod. After 3 weeks, shoots obtained from seedlings were excised and used as explants for transformation studies.
Bacterial strains and culture conditions
Agrobacterium rhizogenes wild-type strains AR15834 and A4 were used to induce hairy roots in C. roseus. Agrobacterium strains were cultured for 2 d on yeast extract mannitol (YEM) medium (Wise et al. Citation2006) with 15 g/L agar and incubated at 28 °C under dark conditions. The bacterial cultures growing in YEM liquid medium at 28 °C for 72 h (with OD600 nm – 0.6–0.8) were used for transformation.
Induction and establishment of hairy root cultures
The buds of 3-week-old micro-propagated shoots were wounded and immersed into the bacterial suspension and incubated for 30 min. Shoots were then blotted dry using sterile filter paper. Infected explants were transferred on MS medium and co-cultivated for 2 and 3 d. But, the other part is directly transferred onto half strength B5 medium and used as the control (Gamborg et al. Citation1968) supplemented with 30 g/L sucrose, 8 g/L agar and 400 mg/L cefotaxime, without passing through the co-culture period. The inoculated explants showing hairy root induction were subcultured two times at 15-d intervals on half-strength B5 semi-solid medium, containing 5.5 g/L agar and 400 mg/L cefotaxime to eliminate the bacteria. After 2-weeks, at the last subculture, hairy root induction frequency was evaluated and expressed as the number of explants with hairy root/the number of the infected explants ×100. The emerging hairy roots were cut (length higher than 2.5 cm) and transferred onto semi-solid half strength B5 medium supplemented with 250 mg/L of cefotaxime. The antibiotic concentration was gradually lowered and finally removed completely after 3 months. All the cultures were maintained in complete darkness at 25 ± 2 °C.
Selection of hairy root lines and liquid adaptation
The selection of hairy root lines was done after the passage of regenerated roots on half strength B5 semi-solid medium without antibiotic. The hairy root lines characterized by a better growth and proliferation after 15 d of culture were selected and sub-cultured in half strength B5liquid medium. Approximately, 4–5 root tips (4–5 cm of length) for each line were collected from solid culture and transferred in Erlenmeyer flasks containing 50 mL of liquid B5/2 medium without cefotaxime. The cultures were incubated in orbital shaker set at 100 rpm and 25 ± 2 °C under dark conditions. After 20 d of liquid culture, the growth index was calculated for each line using the following formula:
PCR analysis
The transgenic nature of hairy roots was confirmed by detecting the presence of rolB gene by PCR amplification using the specific primers (F: 5′-GCG ACA ACG ATT CAA CCA TAT CG-3′, and R: 5′-TTT ACT GCA GCA GGC TTC ATG AC-3′). Total DNA was isolated from transformed and non-transformed roots using NucleoSpin DNA Plant Mini kit (Macherey-Nagle, Düren, Germany). Plasmid DNA isolated from A. rhizogenes cells (strains AR15834 and A4) was used as a positive control. The Ri plasmid was isolated with an alkaline lysis Miniprep. PCR amplification was carried out in a 20 μL reaction volume containing 2 μL DNA solution, 0.8 μL of each primer (forward and reverse), 2 μL of 10 × Taq polymerase buffer, 0.4 μL dNTP mix and 0.4 μL of Taq DNA polymerase. PCR reaction was performed at an initial denaturing temperature of 94 °C for 4 min, followed by 30 cycles of denaturing at 94 °C for 1 min, annealing at 57 °C for 1 min and extension at 72 °C for 1 min and ended with a final extension of 72 °C for 7 min. The PCR products were separated using electrophoresis on 1.2% agarose gel including DNA marker and visualized under UV light after staining with ethidium bromide.
Growth kinetics of selected hairy root lines
The three best lines of hairy roots showing good growth on liquid medium were selected to study the biomass growth kinetics and alkaloids accumulation. About 200 mg fresh weight (approximately five root tips of 4–5 cm) of each hairy root line (20-d-old) maintained onto B5/2 semi-solid medium, was collected and inoculated into 50 mL of liquid B5/2 medium in 250 mL Erlenmeyer flasks. The cultures were kept on shaker (set at 100 rpm) in a growth chamber under dark conditions at 25 ± 2 °C. After 8 d of culture, the hairy roots were harvested after every third day during 35 d of culture, and their dry weight were recorded. The harvested roots were dried in an incubator (60 °C, for 48 h), and then used later for extraction and determination of alkaloids content.
Extraction and analysis of alkaloids
Alkaloids extraction from the dry plant biomass was carried out according to protocol given by Guirimand et al. (Citation2010). Dry hairy roots (25 mg) were taken for alkaloids extraction. Catharanthine and ajmalicine were identified and quantified using liquid chromatography coupled to tandem mass spectrometry (LC-MS/MS) (ThermoFisher Scientific, San Jose, CA). LC-MS/MS analysis was performed on a C18 column (150 mm × 2.1 mm and 5 μm porosity – Alltima, Alltech, Saint Jean de Gonville, France) with a flow rate of 0.2 mL/min at a constant temperature of 25 °C. The mobile phase made up of eluent A (0.1% formic acid in water) and eluent B (0.1% formic acid in methanol) in the following gradient program: 30% B for 30 min, 80% B at 30 min, 98% B at 30,1 min until 5 min and 30% B at 35.1 min until 6 min. The compounds of interest were identified through specific MS2 scans in the addition of MS full scan (50–1000 m/z). Then, they were quantified according UV absorption at 254 nm and retention times of reference which are obtained based on authentic standards of ajmalicine and catharanthine (Sigma-Aldrich, Saint-Quentin-Fallavier, France). Raw data were processed using Xcalibur software (version 2.1 Thermo Scientific, Waltham, MA).
Statistical analyses
The statistical analyses were performed by one-way ANOVA and completed with the Tukey post hoc test. The correlation between biomass growth and ajmalicine and/or catharanthine contents in hairy root lines was determined and quantified by correlation factor. The difference between samples was considered statistically significant at p ≤ 0.05.
Results and discussion
Induction of C. roseus hairy roots
Putative transformants were obtained by co-culturing the explants with A. rhizogenes for 0–3 d. After 12 d of culture (
Table 1. Response of explants of C. roseus cultivars infected with Agrobacterium rhizogenes strains A4 and AR15834 after different co-cultured period.
Figure 1. Morphology of induced hairy roots. (A and B) Induction of hairy root on the wounded site of explants after the 30th day of culture in half-strength B5 semi-solid medium; (C, D and E) the selected hairy root lines; (F, G and H) growth of the LP10 hairy roots line after the 14th, 29th and 32nd days of culture in half-strength B5 liquid medium, respectively.
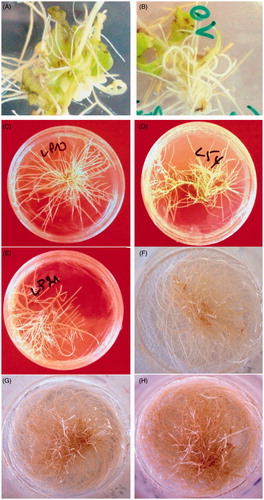
The statistical analysis demonstrates a significant effect (p < 0.05) of co-culture time on the induction frequency that increased progressively with the increase in co-culture period particularly with the AR 15834 strain. Indeed, the frequency of root induction reached 90 and 100% for Cooler lilac and Cooler blush, respectively, in case of 3-d co-culture period. The effect of co-culture period on induction frequency is highly significant in the cultivar cooler blush, which showed an increase of 20% and 25% of root induction on explants with strains A4 and AR15834, respectively.
On the other hand, the co-culture period showed a strongly significant effect on the number of induced hairy roots per explant (). Indeed, this number increased two times as compared to the non-infected explants. The best results were recorded for Cooler lilac co-cultured for 3 d with strain A4 (an average of 10.27 hairy roots per explants; ), showing that period of co-culture was a very important step in the transformation by A. rhizogenes. This step ensures the attachment of bacteria, and then the transfer and integration of T-DNA in the plant genome (Herath et al. Citation2005).
Genetic transformation for some recalcitrant plant species can be achieved in vitro by co-cultivating A. rhizogenes with wounded tissue (Makhzoum et al. Citation2011). Our research showed that the period of co-culture was very important in order to complete the transformation process and enhancing the induction frequency, especially for cooler blush cultivar. Our studies are consistent with several studies on C. roseus, which have confirmed the effect of co-culture period (Choi et al. Citation2004; Taneja et al. Citation2010; Wang et al. Citation2012).
Selection of efficient hairy root lines
Among the 1229 hairy root lines induced in two cultivars, 40 hairy root lines were selected on the basis of their degree of lateral ramification and without callus formation. After 15 d of culture on B5/2 semi-solid medium; 10 efficient lines (line for each cultivar) were selected for their good biomass growth and high ramification degree. There was considerable variability in the morphology and growth patterns of each individual hairy root lines, which can be explained by the fact that each root clone was resulting from an independent transformation event (Batra et al. Citation2004). This genetic variation in hairy root lines could possibly be due to the various modes of expression of Ri bacterium genes according to their integration place, number of copies and the sense of insertion in the plant genome (Chandra Citation2012).
In order to determine the ability to adapt and potential productivity of selected lines in terms of biomass, the hairy root lines were cultured on liquid medium. After 20 d of culture, the established hairy root cultures increased in biomass between 2 and 24 times of the initial inoculums. Three lines L54, LP10 and LP21 () were selected on the basis of their high biomass production (
Table 2. Variation of growth index in selected hairy root lines after 20 d of culture in half-strength B5 liquid medium.
Confirmation of transgenic hairy root lines
PCR results () revealed the presence of a single PCR band corresponding to the rolB gene in the three selected hairy root lines (lanes 4–6) and in Ri plasmid DNA (positive control, lanes 2 and 3). On the other hand, the genomic DNA isolated from the non-transformed roots (control) of the sterile seedlings (negative control, lane 1) did not show any amplification of rolB gene. These results confirmed the transgenic nature of the three selected hairy root lines. This transformation was a consequence of an integration of the bacterial rolB gene into the plant genome (White et al. Citation1985; Gelvin Citation2009).
Figure 2. PCR amplification of rolB gene in hairy root lines of C. roseus. Lane M, molecular weight marker (1000 bp ladder); lane 1, DNA from non-transformed roots; lane 2, DNA from the plasmid A4 (positive control (1); lane 3, DNA from the plasmid 15834 (positive control (2); lane 4, DNA from HR line L54; lane 5, DNA from HR line LP21; lane 6, DNA from HR line LP10.
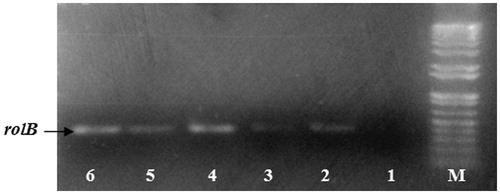
Growth kinetics of hairy root lines
In order to determine the growth kinetics for three selected lines, the dry weight (DW) was used as a measure of biomass accumulation after the culture period of 35 d. As shown in , the hairy root growth was characterized by three development phases. The first days of culture, ranging from 8 to 14 d for LP10 and L54 and from 8 to 11 d for LP21, corresponds to a lag phase, during which the hairy roots adapt to liquid medium and are characterized by a weak and constant dry weight evolution.
The following phase is an exponential phase which can be decomposed into two sub-phases; the first one (ranging from the 14 to 23 d for lines LP10 and L54 and from 11 to 21 d for line LP21) was a weak exponential phase with a small-scale of biomass accumulation. The second one was a true exponential phase with a large-scale biomass accumulation, which was represented by an expedited growth extended until the 32nd day for lines LP10 and L54 and to the 29th day for line LP21. Within this phase, the LP10 line showed a quick and high growth in which the dry weight reached 0.35 g (30-fold the initial weight) at 32 d of culture. The line L54 showed lesser biomass accumulation 0.24 g DW at the 32th day (12-fold the initial weight), whereas the LP21 line showed the lowest dry weight of 0.1 g at the 29th day (10-fold the initial weight) as compared with control. According to Baíza et al. (Citation1998), during the first phase, the low increase in biomass was due to the beginning of the development of lateral roots induced by cell division of intercalary meristematic zones. According to the same authors’, in the second phase, the divided cells were elongated due to the absorption of water, which explained the large growth of biomass.
The last phase was the stationary phase and it reached the 32nd day for LP10 and L54 lines and on the 29th day for the LP21. This phase was characterized by a constant DW for the LP10 line or by a decrease in DW for L54 and LP21 lines. Constancy of growth biomass in stationary phase occurred when the laterals roots had the same size as the main roots. Further, the lateral roots no longer form new lateral roots. Furthermore, the biomass growth stability in the stationary phase can be explained by the exhaustion of nutrients in the culture medium (Harfi et al. Citation2013).
Evolution of catharanthine and ajmalicine accumulation
Transformed root lines can be a promising source for a continuous production of secondary metabolites. This production can be lasting over successive generations without the loss of biosynthetic stability (Giri & Narasu Citation2000). Transformed roots of C. roseus are known to accumulate a large number of monomer alkaloids such as serpentine, ajmalicine and catharanthine (Guillon et al. Citation2008). In the present study, the presence of ajmalicine and catharanthine at all the stages of growth cycle ( and ) in LP10, LP21 and L54 lines were revealed and quantified by LC-MS/MS ().
Figure 4. Evolution of the catharanthine accumulation in the three selected hairy root lines of C. roseus.
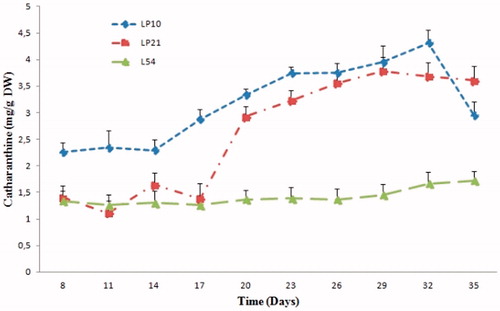
Figure 6. LCMS/MS of the alkaloids of methanolic extract of Catharanthus roseus roots. (A and B) Single ion chromatogram (SIC) of catharanthine [M + H]+parent ion (m/z = 143.50–144.50 + 172.50–173.50) and ajmalicine [M + H]+parent ion (m/z = 143.50–144.50 + 177.50–178.50 + 209.50–210.50 + 221.50–222.50), respectively. (C and D) MS spectrum of catharanthine and ajmalicine, respectively. (E and F) MS2 spectrum of catharanthine and ajmalicine, respectively.
![Figure 6. LCMS/MS of the alkaloids of methanolic extract of Catharanthus roseus roots. (A and B) Single ion chromatogram (SIC) of catharanthine [M + H]+parent ion (m/z = 143.50–144.50 + 172.50–173.50) and ajmalicine [M + H]+parent ion (m/z = 143.50–144.50 + 177.50–178.50 + 209.50–210.50 + 221.50–222.50), respectively. (C and D) MS spectrum of catharanthine and ajmalicine, respectively. (E and F) MS2 spectrum of catharanthine and ajmalicine, respectively.](/cms/asset/4b2f9d74-ee24-4e32-b16e-1619cf3c5149/iphb_a_1140213_f0006_b.jpg)
The time for the fastest growing changes in the hairy root LP10 line () during the 36 d culture period showed that ajmalicine and catharanthine contents are significantly increased up to 17 d and then reached to maximum level at days 29 and 32, respectively. The accumulation of ajamalicine and catharanthine stopped at the end of exponential phase. A peak of ajmalicine and catharanthine accumulation in the line LP10 reached 3.8 and 4.3 mg/g DW, respectively.
On the other hand, the LP21 line showed an alkaloid accumulation profiles similar to that of the LP10 line, but with a slight lower concentration of ajmalicine and catharanthine, so that the maximum level of accumulation was recorded at days 29th and 32nd with a content of 1.97 and 3.7 mg/g DW, respectively.
However, for the L54 line, ajmalicine and catharanthine accumulation was relatively constant during the culture cycle, the content varied between 1.4 and 1.5 mg/g DW for ajamlicine, and from 2.2 to 2.6 mg/g DW for catharanthine. We observed that in the line L54, the content of the ajmalicine was slightly higher than the catharanthine. Nevertheless, the production of catharanthine was higher than that of the ajmalicine in LP10 and LP21 lines. Ferreres et al. (Citation2010) found similar results, wherein the content of catharanthine was higher than that of ajmalicine, in which the concentrations of ajmalicine and catharanthine were 1.6 and 3.2 mg/g DW, respectively. In contrast, other research groups noted a higher concentration of the ajmalicine than that catharanthine in hairy roots of C. roseus. Although their production yields were 0.1–1.25 mg/g DW (Guillon et al. Citation2008) and 0.1–0.7 mg/g DW (Vázquez-Flota et al. Citation1994), respectively, for catharanthine and ajmalicine. The previous results reveal the importance of our results, in which the concentrations of catharanthine and ajmalicine are significantly higher especially for the LP10, with a content of 3.8 and 4.3 mg/g DW, respectively.
Correlation between biomass and alkaloids accumulation
Our results showed a good relationship between the biomass production and the accumulation of ajmalicine and catharantine in three selected hairy root lines (
Table 3. Relationship between biomass growth and alkaloids accumulation of the three selected lines in C. roseus.
Conclusion
Many hairy root lines have been induced from the buds of C. roseus, which show the efficiency of transformation process. Our results obtained from three selected hairy root lines suggest that the evolution of accumulation of ajmalicine and catharanthine is positively correlated with the development of the biomass growth. In addition, production peak of both TIAs is synchronized with the maximum accumulation of biomass; this leads us to conclude that there is a linear correlation between the kinetics of biomass growth and accumulation of ajmalicine and catharanthine. Moreover, our study shows that LP10 line is the most effective and promising line to continue optimizing the production of TIAs. In addition, the end of exponential phase, from the 23rd day until the 32th day, remains the best period to apply elicitation.
In order to significantly reduce the cost of pharmacologically important alkaloids obtained from this plant species, more studies are required to be focused on increasing the content by using new elicitors (in hairy root culture) in combination with other techniques like immobilization, two phase culture, etc. Interestingly, it will also be very useful to study the effects of locus and the chromatin structure on the level of rol genes or other TIAs’ genes and target the best loci with more open chromatin positioning and accessible for transcription and activation and less repressive states.
Funding information
The authors wish to thank the Algerian ministry of high education and scientific research (Algeria) and the ENSAIA of Nancy (France) for supporting this study.
Disclosure statement
The authors report no conflicts of interest. The authors alone are responsible for the content and writing of this article.
References
- Almagro L, Perez AJL, Pedreño MA. 2011. New method to enhance ajmalicine production in Catharanthus roseus cell cultures based on the use of cyclodextrins. Biotechnol Lett. 33:381–385.
- Baíza AM, Quiroz A, Ruíz JA, Maldonado-Mendoza I. 1998. Growth patterns and alkaloid accumulation in hairy root and untransformed root cultures of Datura stramonium. Plant Cell Tissue Organ Cult. 54:123–130.
- Baíza AM, Quiroz-Moreno A, Ruíz JA, Loyola-Vargas VM. 1999. Genetic stability of hairy root cultures of Datura stramonium. Plant Cell Tissue Organ Cult. 59:9–17.
- Batra J, Dutta A, Singh D, Kumar S, Sen J. 2004. Growth and terpenoid indole alkaloid production in Catharanthus roseus hairy root clones in relation to left-and right-termini-linked Ri T-DNA gene integration. Plant Cell Rep. 23:148–154.
- Chandra S. 2012. Natural plant genetic engineer Agrobacterium rhizogenes: role of T-DNA in plant secondary metabolism. Biotechnol Lett. 34:407–415.
- Choi PS, Kim YD, Choi KM, Chung HJ, Choi DW, Liu JR. 2004. Plant regeneration from hairy-root cultures transformed by infection with Agrobacterium rhizogenes in Catharanthus roseus. Plant Cell Rep. 22:828–831.
- Ciau-Uitz R, Miranda-Ham ML, Coello-Coello J, Chí B, Pacheco LM, Loyola-Vargas VM. 1994. Indole alkaloid production by transformed and non-transformed root cultures of Catharanthus roseus. Vitro Cell Dev Biol Plant. 30:84–88.
- Fernández-Pérez F, Almagro L, Pedreño MA, Gómez Ros LV. 2013. Synergistic and cytotoxic action of indole alkaloids produced from elicited cell cultures of Catharanthus roseus. Pharm Biol. 51:304–310.
- Ferreres F, Pereira DM, Valentão P, Oliveira JMA, Faria J, Gaspar L, Sottomayor M, Andrade PB. 2010. Simple and reproducible HPLC-DAD-ESI-MS/MS analysis of alkaloids in Catharanthus roseus roots. J Pharm Biomed Anal. 51:65–69.
- Gamborg OL, Miller RA, Ojima K. 1968. Nutrient requirements of suspension cultures of soybean root cells. Exp Cell Res. 50:151–158.
- Gelvin SB. 2009. Agrobacterium in the genomics age. Plant Physiol. 150:1665–1676.
- Georgiev MI, Pavlov AI, Bley T. 2007. Hairy root type plant in vitro systems as sources of bioactive substances. Appl Microbiol Biotechnol. 74:1175–1185.
- Giri A, Narasu ML. 2000. Transgenic hairy roots. Recent trends and applications. Biotechnol Adv. 18:1–22.
- Guillon S, Gantet P, Thiersault M, Rideau M, Trémouillaux-Guiller J. 2008. Hairy roots of Catharanthus roseus: efficient routes to monomeric indole alkaloid production. In: Ramawat KG, Merillon JM, editors. Bioactive molecules and medicinal plants. Heidelberg, Berlin: Springer. p. 285–295.
- Guirimand G, Courdavault V, Lanoue A, Mahroug S, Guihur A, Blanc N, Giglioli-Guivarćh N, St-Pierre B, Burlat V. 2010. Strictosidine activation in Apocynaceae: towards a “nuclear time bomb”? BMC Plant Biol. 10:182.
- Harfi B, Khelifi L, Bekhouche M, Khelifi-Slaoui M. 2013. Study of biomass growth kinetic’s and hyoscyamine accumulation in hairy roots of three species of Datura. Wulfenia J. 20:306–313.
- Herath SP, Suzuki T, Hattori K. 2005. Factors influencing Agrobacterium mediated genetic transformation of kenaf. Plant Cell Tissue Organ Cult. 82:201–206.
- Hirano H, Komamine A. 1994. Correlation of betacyanin synthesis with cell division in cell suspension cultures of Phytolacca americana. Physiol Plant. 90:239–245.
- Hughes EH, Hong S-B, Gibson SI, Shanks JV, San KY. 2004. Metabolic engineering of the indole pathway in Catharanthus roseus hairy roots and increased accumulation of tryptamine and serpentine. Metab Eng. 6:268–276.
- Ishikawa H, Colby DA, Seto S, Va P, Tam A, Kakei H, Rayl TJ, Hwang I, Boger DL. 2009. Total synthesis of vinblastine, vincristine, related natural products, and key structural analogues. J Am Chem Soc. 131:4904–4916.
- Makhzoum A, Bjelica A, Petit-Paly G, Bernards MA. 2015. Novel plant regeneration and transient gene expression in Catharanthus roseus. Results J Biol. 6:1–9.
- Makhzoum A, Petit-Paly G, St Pierre B, Bernards MA. 2011. Functional analysis of the DAT gene promoter using transient Catharanthus roseus and stable Nicotiana tabacum transformation systems. Plant Cell Rep. 30:1173–1182.
- Makhzoum AB, Sharma P, Bernards MA, Trémouillaux-Guiller J. 2013. Hairy roots: an ideal platform for transgenic plant production and other promising applications. In: Gang DR, editor. Phytochemicals, plant growth, and the environment, recent advances in phytochemistry. NewYork: Springer. p. 95–142.
- Malik S, Bhushan S, Sharma M, Ahuja PS. 2011. Physico-chemical factors influencing the shikonin derivatives production in cell suspension cultures of Arnebia euchroma (Royle) Johnston, a medicinally important plant species. Cell Biol Int. 35:152–157.
- Malik S, Bíba O, Grúz J, Arroo RRJ, Strnad M. 2014. Biotechnological approaches for producing aryltetralin lignans from Linum species. Phytochem Rev. 13:893–913.
- Malik S, Bhushan S, Sharma M, Ahuja PS. 2016. Biotechnological approaches to the production of shikonins: a critical review with recent updates. Crit Rev Biotechnol. 36:. 327–340.
- Moreno-Valenzuela OA, Minero-García Y, Chan W, Mayer-Geraldo E, Carbajal E, Loyola-Vargas VM. 2003. Increase in the indole alkaloid production and its excretion into the culture medium by calcium antagonists in Catharanthus roseus hairy roots. Biotechnol Lett. 25:1345–1349.
- Mujib A, Ilah A, Aslam J, Fatima S, Siddiqui ZH, Maqsood M. 2012. Catharanthus roseus alkaloids: application of biotechnology for improving yield. Plant Growth Regul. 68:111–127.
- Murashige T, Skoog F. 1962. A revised medium for rapid growth and bio assays with tobacco tissue cultures. Physiol Plant. 15:473–497.
- Noble RL. 1990. The discovery of the vinca alkaloids-chemotherapeutic agents against cancer. Biochem Cell Biol. 68:1344–1351.
- Rai GK, Rai NP, Kumar S, Yadav A, Rathaur S, Singh M. 2012. Effects of explant age, germination medium, pre-culture parameters, inoculation medium, pH, washing medium, and selection regime on Agrobacterium-mediated transformation of tomato. Vitro Cell Dev Biol Plant. 48:565–578.
- Taneja J, Jaggi M, Wankhede DP, Sinha AK. 2010. Effect of loss of T-DNA genes on MIA biosynthetic pathway gene regulation and alkaloid accumulation in Catharanthus roseus hairy roots. Plant Cell Rep. 29:1119–1129.
- Tao J, Li L. 2006. Genetic transformation of Torenia fournieri L. mediated by Agrobacterium rhizogenes. South Afr J Bot. 72:211–216.
- Toivonen L, Balsevich J, Kurz WGW. 1989. Indole alkaloid production by hairy root cultures of Catharanthus roseus. Plant Cell Tissue Organ Cult. 18:79–93.
- Van Der Heijden R, Jacobs DI, Snoeijer W, Hallard D, Verpoorte R. 2004. The Catharanthus alkaloids: pharmacognosy and biotechnology. Curr Med Chem. 11:607–628.
- Vázquez-Flota F, Moreno-Valenzuela O, Miranda-Ham ML, Coello-Coello J, Loyola-Vargas VM. 1994. Catharanthine and ajmalicine synthesis in Catharanthus roseus hairy root cultures. Plant Cell Tissue Organ Cult. 38:273–279.
- Wang Q, Xing S, Pan Q, Yuan F, Zhao J, Tian Y, Chen Y, Wang G, Tang K. 2012. Development of efficient Catharanthus roseus regeneration and transformation system using Agrobacterium tumefaciens and hypocotyls as explants. BMC Biotechnol. 12:12.
- White FF, Taylor BH, Huffman GA, Gordon MP, Nester EW. 1985. Molecular and genetic analysis of the transferred DNA regions of the root-inducing plasmid of Agrobacterium rhizogenes. J Bacteriol. 164:33–44.
- Wise AA, Liu Z, Binns AN. 2006. Culture and maintenance of Agrobacterium strains: Agrobacterium protocols. In: Wang K, editor. Methods in molecular biology. Totowa, NJ: Springer, Humana Press Inc. vol. 343. p. 3–13.