Abstract
Context Calligonum polygonoides L. subsp. comosum L’ Hér. (Polygonaceae), locally known as “arta”, is a slow-growing small leafless desert shrub.
Objective Isolation, structure elucidation and evaluation of cytotoxic activity of flavonoids from C. polygonoides aerial parts.
Materials and methods Flavonoids in the hydroalcoholic extract of the of C. polygonoides were isolated and purified using column chromatography and preparative HPLC. The structures of the isolated flavonoids were elucidated on the basis of spectroscopic data including 2D NMR techniques. The cytotoxic activity of the isolated flavonoids (6.25, 25, 50 and 100 μg/mL) was evaluated against liver HepG2 and breast MCF-7 cancer cell lines using sulphorhodamine-B assay.
Results A new flavonoid, kaempferol-3-O-β-D-(6″-n-butyl glucuronide) (1), and 13 known flavonoids, quercetin 3-O-β-D-(6″-n-butyl glucuronide) (2), kaempferol-3-O-β-D-(6″-methyl glucuronide) (3), quercetin-3-O-β-D-(6″-methyl glucuronide) (4), quercetin-3-O-glucuronide (5), kaempferol-3-O-glucuronide (6), quercetin-3-O-α-rhamnopyranoside (7), astragalin (8), quercetin-3-O-glucopyranoside (9), taxifolin (10), (+)-catechin (11), dehydrodicatechin A (12), quercetin (13), and kaempferol (14), were isolated from the aerial parts of C. polygonoides. Quercetin showed significant cytotoxic activity against HepG2 and MCF-7 cell lines with IC50 values of 4.88 and 0.87 μg/mL, respectively. Structure–activity relationships were analyzed by comparing IC50 values of several pairs of flavonoids differing in one structural element.
Discussion and conclusion The activity against breast cancer cell lines decreased by glycosylation at C-3. The presence of 2,3-double bond in ring C, carbonyl group at C-4 and 3’,4’-dihydroxy substituents in ring B are essential structural requirements for the cytotoxic activity against breast cancer cells.
Keywords:
Introduction
The genus Calligonum (Polygonaceae), from the Greek kalli “beautiful” and gony “knee joint”, includes 80 plant species distributed throughout Europe, North Africa, West and Central Asia (Boulos Citation2000). Calligonum polygonoides L. subsp. polygonoides and subsp. comosum (L’ Hér.) occur in Egypt. These two subspecies are often treated in the literature as separate species (Singh Citation2004; Samejo et al. Citation2013). Calligonum polygonoides L. subsp. comosum (L’ Hér.) is a shrub growing on sandy deserts of different phytogeographical regions in Egypt. This plant is traditionally used for treatment of stomach ailments and toothache by chewing the aerial parts. The root decoction is used for gum sores. The fresh flowers of the plant are used as food due to high content of sugar and nitrogenous components (Zouari et al. Citation2012). The aerial parts of the plant showed protective activity against haloperidol-induced oxidative stress (Abdel-Sattar et al. Citation2014), antiosteoporotic (Sabry et al. Citation2013), anti-ulcer and anti-inflammatory (Liu et al. Citation2001), hypoglycaemic (El-Hawary & Kholief Citation1990), cytotoxicity and antioxidant activities (Badria et al. Citation2007). The fruits of the plant showed antihelmentic activity (Degheidy et al. Citation2013). Antimicrobial activity for different plant parts was reported (Riadh et al. Citation2011; Alkhalifah Citation2013). Previous phytochemical studies on C. polygonoides reported the isolation of kaempferol, quercetin, (+)-catechin, dehydrodicatechin A, kaempferol-3-O-rhamnopyranoside, quercitrin, isoquercitrin, kaempferol-3-O-glucuronide, quercetin-3-O-glucuronide, pro-cyanidines, β-sitosterol-3-O-glucoside, violaxanthin and neoxanthin carotenoids (El-Sayyad & Wagner Citation1978; Badria et al. Citation2007).
Numerous plant-derived compounds are beneficial for the prevention and treatment of cancer. Among these compounds are flavonoids which are naturally occurring polyphenolic metabolites ubiquitously present in higher plants. Anticarcinogenic activity of flavonoids may be mediated by mitigation of oxidative damage, inactivation of carcinogen, inhibition of proliferation, promotion of differentiation, induction of cell-cycle arrest and apoptosis, impairment of tumour angiogenesis and suppression of metastasis (Sak Citation2014). Flavonoids have been shown to exert minimal effects on normal cells with a significant cytotoxic activity against various human cancer cells. This makes flavonoids ideal agents for developing anticancer drugs (Plochmann et al. Citation2007; Ben Sghaier et al. Citation2011). Although most of the biological actions of flavonoids depend on their structure, this point is still unclear for anticancer activity.
In this report, phytochemical investigation on the aerial parts of C. polygonoides L. subsp. comosum was carried out. A novel kaempferol glycoside and 13 known flavonoids were isolated from the hydroalcoholic extract. The structures of the isolated compounds were elucidated on the basis of spectroscopic data. The cytotoxic activity of these compounds was evaluated in human liver cancer cells (HepG-2) and breast cancer cells (MCF-7). Structure–activity relationships were analyzed by comparing the IC50 value of several pairs of flavonoids differing only in one structural element.
Materials and methods
General
HPLC (Agilent 1260 Infinity, Waldbronn, Germany) equipped with an Agilent 1260 Infinity preparative pump (G1,361A), Agilent 1260 diode array detector VL (G1,315D), Agilent 1260 Infinity thermostated column compartment (G1,361A) and Agilent 1260 Infinity preparative Autosampler (G2,260A) was used. Separation and quantitation were performed on a ZORBAX SB-C18 preparative column (21.2 × 250 mm i.d, 7 μm particle size) (USA). Jeol mass spectrophotometer (Jeol Inc., Tokyo, Japan) was used for measuring mass spectrometry. NMR spectra were recorded using Bruker Avance III 400 MHz for 1H and 100 MHz for 13C (Bruker AG, Fällanden, Switzerland) with BBFO Smart Probe and Bruker 400 EON Nitrogen-Free Magnet NMR spectrometer. Data were analyzed using Topspin 3.1 Software (Bruker AG, Fällanden, Switzerland). The UV spectra were acquired in methanol using Shimadzu UV-1,601PC UV–Visible, scanning spectrophotometer (Shimadzu Corp., Tokyo, Japan). Stationary phases used were normal phase silica gel 60, polyamide (Sigma-Aldrich Chemicals, Darmstadt, Germany) and sephadex LH-20 (E Merck). TLC was carried out using precoated silica gel F254 TLC plates. Tris buffer (Applichem, Darmstadt, Germany), Dulbecco’s Modified Eagle Medium (DMEM), Trypan blue, fetal bovine serum, penicillin/streptomycin antibiotic and Trypsin-EDTA (Sigma Aldrich Chemical Co., St. Louis, MO) were used for measuring cytotoxicity.
Plant material
Calligonum polygonoides was collected from western desert, Giza governorate, Egypt, during April 2012 during the flowering stage. The plant was taxonomically identified by Dr. Abdelhalim Mohamed (Plant Taxonomy Department, Agricultural Research Center, Cairo, Egypt). Voucher specimen (BUPD-40) was deposited at the Department of Pharmacognosy, Faculty of Pharmacy, Beni-Suef University. The aerial parts were separated from freshly collected plant material, air-dried in shade at room temperature, powdered and stored in an airtight container till use.
Extraction and isolation
The dried powdered plant of C. polygonoides (2 kg) was exhaustively extracted by cold maceration (×4, each 48 h) with aqueous ethanol (70%). The extract was evaporated under reduced pressure. The obtained residue was suspended in distilled water (1 L) and successively partitioned with n-hexane (5 × 500 mL), methylene chloride (5 × 500 mL), ethyl acetate (6 × 500 mL) and n-butanol (6 × 500 mL). The different fractions were evaporated to dryness to yield 2, 5, 10 and 45 g residues. The n-butanol fraction (10 g) was subjected to polyamide column chromatography (120 × 2.8 cm) using H2O–MeOH mixture with 10% increment increase in polarity. Subfractions were monitored with TLC. Subfraction B1 (150 mg) eluted with 50% H2O–MeOH mixture was subjected to Sephadex LH-20 column chromatography using MeOH to yield two subfractions B1A and B1B. Subfraction B1A was purified by RP-HPLC using H2O:0.03% formic acid (A) and MeOH:0.03% formic acid (B) in a gradient mode: A/B 80/20–50/50; 5 min, 50/50–50/50; 15 min, 50/50–0/100; 10 min with a flow rate of 10 mL/min to afford compound 1 (tR = 29.44 min, 24 mg), 2 (tR = 27.21 min, 15 mg), 3 (tR = 18.56 min, 14 mg) and 4 (tR = 14.42 min, 6 mg). Subfraction B1B was further purified on Sephadex LH-20 column using 75% MeOH in H2O to afford compound 5 (15 mg) and compound 6 (10 mg). Subfraction B2 (180 mg) eluted with 60–70% MeOH:H2O mixture from the polyamide column was subjected to Sephadex LH-20 column chromatography using 80% MeOH in H2O, to afford compound 7 (7 mg) and a mixture of two compounds. This mixture was further purified on silica gel column using CH2Cl2:MeOH with 4% increase in polarity until 100% MeOH. Two subfractions were obtained and were further purified on Sephadex LH-20 columns using 75% MeOH in H2O to afford compound 8 (9 mg) and compound 9 (12 mg).
The ethyl acetate fraction (10 g) was subjected to polyamide column chromatography (120 × 2.8 cm) using H2O–MeOH mixture with 10% increment increase in polarity. Two main subfractions (E1 and E2) were obtained. Subfraction E1 (1 g) eluted with aqueous methanol (50–60%) was subjected to further purification on silica gel column (100 g) using n-hexane:ethyl acetate with 10% increase in polarity until 100% ethyl acetate. Two subfractions were obtained and were further purified on Sephadex LH-20 column using 80% MeOH to afford compound 10 (10 mg), compound 11 (70 mg) and compound 12 (8 mg). Subfraction E2 (50 mg) eluted with aqueous methanol (90% and 100%) was further purified with Sephadex LH-20 column (10 g, 60 × 1 cm) to afford compound 13 (10 mg), and compound 14 (5 mg).
Kaempferol-3-O-β-D-(6″-n-butyl glucuronide) (1)
Yellow amorphous powder; mp 170–173°C; UV (MeOH) λmax nm: 266, 304sh, 350; HR-ESI-MS negative m/z 518.102 [M]− (calcd for C25H26O12, 518.124). 1H NMR (CD3OD, 400 MHz) and 13C NMR (CD3OD, 100 MHz), see .
Table 1. The 1H- and 13C NMR data for compound 1 in CD3OD.
Cytotoxic activity
Human hepatocarcinoma cell line (HepG2) and human caucasian breast adenocarcinoma cell line (MCF-7) were obtained from the American Type Culture Collection (ATCC, Alexandria, MN) through the Tissue Culture Unit, the Egyptian Organization for Biological Products and Vaccines, Vacsera, Egypt. The cytotoxicity was carried out using sulphorhodamine-B assay following the method reported by Vichai and Kirtikara (Citation2006). Adriamycin (doxorubicin) was used as a positive control. All the procedure, reagents and buffers used in the assay were prepared according to a reported method (Abdelgawad et al. Citation2014). In brief, cells were seeded in 96 well micro-titre plates at a concentration of 1000–2000 cells/100 μL/well. After 24 h, cells will be incubated for 72 h with various concentrations of the tested flavonoid or the positive control (6.25, 25, 50 and 100 μg/mL) prepared in dimethylsulphoxide. For each concentration, 3 wells were used. The plates were incubated for 72 h. The medium is discarded and the cells were fixed with 150 μL cold trichloroacetic acid for 1 h at 4 °C. The plates were washed with distilled water using and stained with 50 μL 0.4% sulphorhodamine-B dissolved in 1% acetic acid for 30 min at room temperature in the dark. The plates were washed with 1% acetic acid to remove the unbound dye and air-dried for 24 h. The dye was solubilized with 150 μL/well of 10 mM tris base (pH 7.4) for 5 min on a shaker at 1600 rpm. The optical density (OD) of each well was measured spectrophotometrically at 490 nm with an ELISA microplate reader. The mean background absorbance was automatically subtracted and mean values of each concentration was calculated. The experiment was repeated three times. The percentage of cell survival was calculated as follows:
The IC50 value was defined as the concentration of the compound required to inhibit 50% of cell growth.
Statistical analysis
The cytotoxicity data were analyzed by one-way analysis of variance (ANOVA) followed by Dunnett’s test for comparison with control data.
Results and discussion
Isolation of flavonoids from C. polygonoides
Phytochemical investigation of n-butanol and ethyl acetate-soluble fractions of the hydroalcoholic extract of C. polygonoides led to isolation of 14 flavonoids (); one of these compounds is new (1) and four compounds (2, 4, 8 and 10) were isolated for the first time from the genus Calligonum. The known compounds were identified as quercetin 3-O-β-D-(6″-n-butyl glucuronide) (2) (Lin et al. Citation2011), kaempferol-3-O-β-d-(6″-methyl glucuronide) (3) (Al Sayed et al. Citation2010), quercetin-3-O-β-d-(6″-methyl glucuronide) (4) (Li et al. Citation2009), quercetin-3-O-glucuronide (mequilianin) (5), kaempferol-3-O-glucuronide (6), quercetin-3-O-α-rhamnopyranoside (quercitrin) (7) (Badria et al. Citation2007), astragalin (8) (Markham & Ternai Citation1976), quercetin-3-O-glucopyranoside (isoquercitrin) (9) (Badria et al. Citation2007), taxifolin (10) (Kim et al. Citation2009), (+)-catechin (11), dehydrodicatechin A (12), quercetin (13), kaempferol (14) (Badria et al. Citation2007) based on spectroscopic analysis and comparison with literature data.
Structure elucidation of compound 1
Compound 1 was isolated as a yellow amorphous solid. The UV spectrum showed absorptions at 266 and 350 nm. The 1D and 2D NMR spectra including distortionless enhancement by polarization transfer with retention of quaternaries (DEPT-Q), heteronuclear single quantum correlation (HSQC) and heteronuclear multiple bond connectivity (HMBC) experiments have shown that compound 1 is a kaempferol glycoside (Badria et al. Citation2007). The 1H NMR spectrum exhibited two 1H signals at δH 6.33 and 6.16 due to the m-coupled protons attached to C-8 and C-6 of ring A, respectively. The 1H NMR spectrum also showed the presence of two doublet signals at δH 8.01 (2H, d, J = 8.4 Hz) and 6.85 (2H, d, J = 7.6 Hz) due to the p-substituted ring B. Attachment of the sugar moiety to the flavonoid nucleus at C-3 was confirmed from the HMBC correlation between the anomeric proton signal at δH 5.29 and δC 133.9 of C-3 of ring C in the kaempferol aglycone. The anomeric proton signal at δH 5.29 showed HSQC correlation with a signal at δC 103.2. This assigned the carbon signal to C-1″ of the sugar moiety. The signal at δC 103.2 showed a HMBC correlation with proton signal at δH 3.82. The latter proton signal showed another HMBC correlation with the quaternary carbon signal at δC 168.7. This assigned the proton signal at δH 3.82 to H-5″ of the sugar moiety and suggested the presence of a glucuronic acid moiety. The HSQC correlation between the proton signal at δH 3.82 and the carbon signal at δC 75.5, assigned this carbon signal to C-5″. The signal at δC 75.5 showed a HMBC correlation with an overlapped signal at δH 3.53–3.64. Therefore, this proton signal was assigned to H-4″ of the glucuronic acid moiety. The HSQC correlation between the signals at δH 3.53–3.64 and δC 71.3 assigned this carbon signal to C-4″. Another HMBC correlation between H-4″ signal at δH 3.53–3.64 and δC 76.0 assigned this carbon signal to C-3″. This left another methine carbon signal at δC 74.0 that was assigned to C-2″. The 1H NMR spectrum showed the presence of methyl proton signal at δH 0.83, two aliphatic proton signals at δH 1.51 and 1.26, and a signal at δH 4.06 for a proton attached to an oxygenated carbon. This suggested the presence of a short aliphatic chain. The HMBC correlation between proton signal at δH 4.06 and the carbon signal at δC 168.7 of C-6″, suggested the attachment of the aliphatic chain to the glucuronic acid moiety at C-6″. The proton signal at δH 4.06 showed a HSQC correlation to carbon signal at δC 64.8 (C-1‴), and another HMBC correlation to the carbon signal at δC 30.1. This assigned the latter carbon signal to C-2‴. The latter carbon signal showed a HMBC correlation to proton signal at δH 1.26. Therefore, this proton signal was assigned to H-3‴ that showed a HSQC correlation to carbon signal at δC 18.6 (C-3‴). The methyl protons at 0.83 showed a HMBC correlation to the latter carbon signal. This suggested that compound 1 is kaempferol-3-O-β-D-(6″-n-butyl glucuronide). This was confirmed from the negative HR-ESI-MS at m/z 518.102 [M]−, calculated for the molecular formula C25H26O12: 518.142.
Cytotoxic activity of the isolated flavonoids
The 14 isolated flavonoids were tested for their cytotoxic effects on liver cancer (HepG2) and breast cancer (MCF-7) cell lines ( and ). The tested flavonoids belong to flavan, flavanone and flavonol types and present as aglycones and glycosides. Cytotoxic activity of flavonoids was reported to be related to the origin of cancer cells (Chang et al. Citation2008). In case of liver cancer cells, for example, fluctuation in cytotoxic constants of flavonoids may be caused by exposure of cells to different metabolites (Sak Citation2014). In the current study, quercetin and astragalin exhibited the highest cytotoxic effect on HepG2 cells. Quercetin, quercetin-3-O-glucuronide and taxifolin exhibited the highest cytotoxic effect against MCF-7 cells. Quercetin seemed to be the most potent in decreasing the survival percent of HepG2 and MCF-7 cell lines than other compounds with IC50 values of 4.88 and 0.87 μg/mL, respectively. It was less active against HepG2 but more effective against MCF-7, compared with the standard compound doxorubicin. Quercetin was reported to be used in combination with doxorubicin to protect against doxorubicin-induced cardiotoxicity and hepatotoxicity (Dong et al. Citation2014). Although quercetin showed the highest cytotoxic effects among the tested flavonoids, quercetin effect at lower concentration, below (IC50), exhibited a plateau as shown from its dose–response curve (). Except for quercetin-3-O-glucuronide, all the tested glycosides showed the least cytotoxic activity against MCF-7 cells. Dehydrodicatechin A also showed low activity against MCF-7 cells and was the least active against HepG2 cells.
Figure 2. Dose–response curves for cytotoxic effects of the flavonoids isolated from the hydroalcoholic extract of Calligonum polygonoides. Numbers written above each graph correspond to chemical structures in . Cytotoxicity was assessed on HepG2 (solid line) and MCF-7 (dotted line).
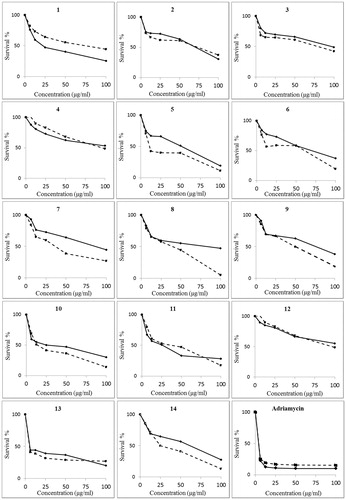
Table 2. Cytotoxic activity (IC50, μg/mL) of flavonoids isolated from Calligoum polygonoides.
The IC50 value of quercetin against MCF-7 cells was 0.87 μg/mL. Taxifolin, that differs from quercetin only in lacking the 2,3-double bond of ring C, had an IC50 value of 15.90 μg/mL. This indicates that the 2,3-double bond in ring C is important for the cytotoxic activity against the breast cancer cell lines. It is noteworthy to mention that flavones were reported to show greater activity against HepG2 compared with flavanones (Li et al. Citation2008). The IC50 value of catechin, that lacks the C-4 carbonyl group in ring B compared with taxifolin, was 27.78 μg/mL. This indicates that flavanones have higher cytotoxic activity on breast cancer cells than flavones. Moreover, the difference in cytotoxicity against breast cancer cells of quercetin (IC50 value of 0.87 μg/mL) compared with kaempferol (IC50 value of 27.81 μg/mL) suggests that that number of OH groups in B ring is important. Our results are in agreement with a previous study (Chang et al. Citation2008) reporting that the presence of 2,3-double bond and ortho-hydroxylation in ring B are essential for flavonoid cytotoxicity. shows the important structure features that affect the cytotoxic activity of flavonoids against breast cancer cells.
Figure 3. Structure elements in flavonoids that increase (solid circle) or decrease (dotted circle) cytotoxic activity against breast cancer cells.
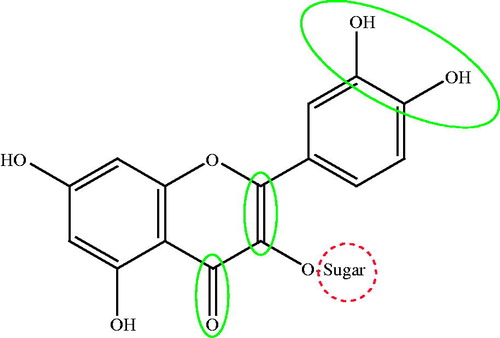
In conclusion, a novel kaempferol glycoside was isolated from the hydroalcoholic extract of C. polygonoides along with 13 known flavonoids. Isolation of structurally related flavonoids enabled to test the cytotoxic actions of these compounds against two human cell lines. Cytotoxic action of flavonoids against liver cancer cell lines showed more fluctuation than breast cancer cell lines. The cytotoxicity of flavonoids against breast cancer cell lines decreased by glycosylation. The presence of 2,3-double bond in ring C, carbonyl group at C-4 and ortho-hydroxylation in ring B increases the cytotoxicity of flavonoids against breast cancer cells. More studies are required to compare the effect of cytotoxic flavonoids in combination with commercially used cytotoxic agents aiming at decreasing side effects induced by these drugs.
Disclosure statement
The authors report no conflicts of interest. The authors alone are responsible for the content and writing of the article.
References
- Abdelgawad M, Abdellatif K, Ahmed O. 2014. Design, synthesis and anticancer screening of novel pyrazole derivatives linking to benzimidazole, benzoxazole and benzothiazole. Med Chem. 2:2161–2168.
- Abdel-Sattar EA, Mouneir SM, Asaad GF, Abdallah HM. 2014. Protective effect of Calligonum comosum on haloperidol-induced oxidative stress in rat. Toxicol Ind Health. 30:147–153.
- Al Sayed E, Martiskainen O, Sinkkonen J, Pihiaja K, Ayoub N, Singab AE, El-Azizi M. 2010. Chemical composition and bioactivity of Pleiogynium timoriense (Anacardiaceae). Nat Prod Commun. 5:545–550.
- Alkhalifah DH. 2013. In-vitro antibacterialactivity of ethanol extract of Calligonum comosum plant against four human pathogens in Saudi Arabia. Int J Plant Anim Environ Sci. 3:170–175.
- Badria FA, Ameen M, Akl MR. 2007. Evaluation of cytotoxic compounds from Calligonum comosum L. growing in Egypt. Z Naturforsch C, J Biosci Naturforsch C. 62:656–660.
- Ben Sghaier M, Skandrani I, Nasr N, Franca MGD, Chekir-Ghedira L, Ghedira K. 2011. Flavonoids and sesquiterpenes from Tecurium ramosissimum promote antiproliferation of human cancer cells and enhance antioxidant activity: a structure-activity relationship study. Environ Toxicol Pharmacol. 32:336–348.
- Boulos L. (2000). Flora of Egypt. Cairo, Egypt: Al Hadara Publishing Inc.
- Chang H, Mi M, Ling W, Zhu J, Zhang Q, Wei N, Zhou Y, Tang Y, Yuan J. 2008. Structurally related cytotoxic effects of flavonoids on human cancer cells in vitro. Arch Pharm Res. 31:1137–1144.
- Degheidy NS, Sharaf EM, Fathi SM. 2013. Field evaluation of anthelmentic efficacy of Calligonum comosum against fasciolosis in sheep at Taif, KSA. Global Veterinaria. 11:377–384.
- Dong Q, Chen L, Lu Q, Sharma S, Li L, Morimoto S, Wang G. 2014. Quercetin attenuates doxorubicin cardiotoxicity by modulating Bmi-1 expression. Br J Pharmacol. 171:4440–4454.
- El-Hawary Z, Kholief T. 1990. Biochemical studies on some hypoglycemic agents (II) effect of Calligonum comosum extract. Arch Pharm Res. 13:113–116.
- El-Sayyad S, Wagner H. 1978. A phytochemical study of Calligonum comosum L. Henry. Planta Med. 33:262–264.
- Kim KH, Chang SW, Ryu SY, Choi SU, Lee KR. 2009. Phytochemical constituents of Nelumbo nucifera. Nat Prod Sci. 15:90–95.
- Li N, Liu JH, Zhang J, Yu BY. 2008. Comparative evaluation of cytotoxicity and antioxidative activity of 20 flavonoids. J Agric Food Chem. 56:3876–3883.
- Li H, Ma Q, Liu Y, Qian J, Zhou J, Zhao Y. 2009. Chemical constituents from Polygonum perfoliatum. Chin J App Environ Biol. 15:615–620.
- Li H, Ma Q, Liu Y, Qian J, Zhou J, Zhao Y. 2011. Evaluation of the potential hypoglycemic and Beta-cell protective constituents isolated from Corni fructus to tackle insulin-dependent diabetes mellitus. J Agric Food Chem. 59:7743–7751.
- Liu XM, Zakaria MN, Islam MW, Radhakrishnan R, Ismail A, Chen HB, Chan K, Al-Attas A. 2001. Anti-inflammatory and anti-ulcer activity of Calligonum comosum in rats. Fitoterapia. 72:487–491.
- Markham K, Ternai B. 1976. 13C NMR of flavonoids – II: flavonoids other then flavone and flavonol aglycones. Tetrahedron. 32:2607–2612.
- Plochmann K, Korte G, Koutsilieri E, Richling E, Riederer P, Rethwilm A, Schreier P, Scheller C. 2007. Structure-activity relationships of flavonoid-induced cytotoxicity on human leukemia cells. Arch Biochem Biophys. 460:1–9.
- Riadh H, Imen F, Abdelmajid Z, Sinda F. 2011. Detection and extraction of anti-Listerial compounds from Calligonum comosum, a medicinal plant from arid regions of Tunisia – compounds Calligonum, medical from regions Tunisia. Afr J Tradit Complement Altern Med J Compl Med. 8:322–327.
- Sabry L, Sattar MA, Amin HA, Sattar EA. 2013. Antiosteoporotic effect of some herbal extracts versus alendronate on an animal model of osteoporosis. Life Sci J. 10:177–187.
- Sak K. 2014. Cytotoxicity of dietary flavonoids on different human cancer types. Pharmacogn Rev Rev. 8:122–146.
- Samejo MQ, Memon S, Bhanger MI, Khan KM. 2013. Chemical composition of essential oil from Calligonum polygonoides Linn. Nat Prod Res. 27:619–623.
- Singh G. 2004. Influence of soil moisture and nutrient gradient on growth and biomass production of Calligonum polygonoides in Indian desert affected by surface vegetation. J Arid Environ. 56:541–558.
- Vichai V, Kirtikara K. 2006. Sulforhodamine B colorimetric assay for cytotoxicity screening. Nat Protoc. 1:1112–1116.
- Zouari S, Dhief A, Aschi-Smiti S. 2012. Chemical composition of essential oils of Calligonum comosum cultivated at the South-Eastern of Tunisia: a comparative study between flowering and fructification stages. J Essent Oil Bear Pl. 15:320–327.