Abstract
Context Diabetes is a common metabolic disease with long-term complications. Prunus mume Sieb. et Zucc. (Rosaceae) fruits have shown to ameliorate glucose intolerance. However, the antidiabetic effects of P. mume leaves have not been investigated.
Objective This study evaluated the effects of P. mume leaf 70% ethanol extract (PMLE) on alleviating diabetes in vivo and in vitro.
Materials and methods PMLE was fractionated into n-hexane, dichloromethane (CH2Cl2), ethyl acetate (EtOAc), n-butanol (BuOH) and water. Polyphenol and flavonoid contents in PMLE fractions were determined using Folin–Ciocalteu reagent and the aluminium chloride colorimetric method, respectively. We evaluated α-glucosidase inhibition using a microplate reader at 400 nm. Adipocyte differentiation by lipid accumulation was measured using Nile Red staining. Male imprinting control region (ICR) mice were injected with streptozotocin (STZ, 100 mg/kg, i.p.). High-fat diets were provided for three weeks prior to PMLE treatments to induce type 2 diabetes. PMLE (0, 5, 25 or 50 mg/kg) was administrated for four weeks with high-fat diets.
Results The EtOAc fraction of PMLE inhibited α-glucosidase activity (IC50 = 68.2 μg/mL) and contained 883.5 ± 14.9 mg/g of polyphenols and 820.1 ± 7.7 mg/g of flavonoids. The 50 mg/kg PMLE supplement reduced 40% of blood glucose level compared to obese/diabetes mice. Obese/diabetic mice treated with 50 mg/kg PMLE showed a lower level of triacylglycerol (320.7 ± 20.73 mg/dL) compared to obese/diabetes mice (494.9 ± 14.80 mg/dL).
Conclusion The data demonstrate that P. mume leaves exert antidiabetic effects that may be attributable to high concentrations of polyphenols and flavonoids.
Introduction
Diabetes is one of the most common metabolic diseases in the world (Fu & Prasad Citation2014). In 2014, the World Health Organization (WHO) estimated that the global incidence of diabetes in adults was 9% and that four out of five diabetes deaths occur in low or middle-income countries (WHO Citation2014).
Type 1 diabetes is characterized by inadequate insulin secretion by pancreatic β-cells, whereas type 2 diabetes (T2D) or non-insulin dependent diabetes mellitus (NIDDM), is characterized by insulin resistance and high blood glucose (Alberti & Zimmet Citation1998; Shin et al. Citation2013). Development of T2D results in insulin resistance, hyperinsulinemia, defective insulin secretion, decreased glucose uptake and glucose utilization by insulin (Gruenwald et al. Citation2010). Patients with T2D have a high risk of long-term complications including renal failure, blindness, hypertension, neuropathy, atherosclerosis, hyperlipidemia, slow-healing wounds and arterial diseases (U. K. Prospective Diabetes Study Group Citation1998; Lin & Sun Citation2010).
α-Glucosidases in the brush border of the small intestine and in the pancreas are responsible for the hydrolysis of starches and absorption of glucose, respectively (Deshpande et al. Citation2009). Evidence has accumulated to demonstrate that α-glucosidase inhibitors reduce digestion and absorption rates of carbohydrate, thereby ameliorating rapid increases in blood glucose levels in T2D patients (Deshpande et al. Citation2009). Krentz and Bailey (Citation2005) reported that T2D can be effectively controlled by strong inhibition of intestinal α-glucosidases and mild inhibition of pancreatic α-amylase.
Peroxisome proliferator activated receptor-gamma (PPARγ), the nuclear hormone receptor and transcription factors, plays a major role in adipocyte differentiation. Targeted deletion of PPARγ leads to elevated levels of plasma free fatty acids and triglycerides (TG), which are associated with insulin resistance (He et al. Citation2003). Thiazolidinediones (TZDs) are agonists for PPARγ and have been widely used to treat T2D for more than 20 years (Martens et al. Citation2002; Wilding Citation2006), owing to their ability to increase the sensitivity of muscle, adipose tissue and liver to the action of insulin (Bermudez et al. Citation2010). Therefore, changes in adipocyte differentiation rates, measured by changes in TG accumulation, can be used to indirectly screen plants extract for compounds with PPARγ agonist activity that represents potential antidiabetic compounds (Norisada et al. Citation2004).
Some plant-originated compounds have been shown to reduce blood glucose levels with few side effects, in humans and in animal models of T2D (Modak et al. Citation2007). Prunus mume Sieb. et Zucc. (Rosaceae) is a popular ornamental plant, originating in Southwest China and widely cultivated throughout East Asia. The fruit of P. mume, plum, is processed into a dried form, pickle, extract, liquor or soft drink, and is known to have high phenolic content and antioxidant activities (Utsunomiya et al. Citation2002). The ethanol extract of P. mume fruits has been shown to stimulate glucose uptake by regulating PPARγ in C2C12 myotubes and ameliorates glucose intolerance and fat accumulation in mice fed with a high-fat diet (Shin et al. Citation2013). In addition, consumption of concentrated juice significantly increased the levels of plasma adiponectin and PPARγ mRNA expression in adipose tissue of Wistar rats (Utsunomiya et al. Citation2005). Therefore, we speculated that other parts of the P. mume plant might also have antidiabetic effects.
To validate the antidiabetic effects of P. mume leaves, we administered 70% ethanol extract of P. mume leaf (PMLE) to obese/diabetes mice and measured blood glucose level, total cholesterol, TG and body weight. We investigated the antidiabetic effects of P. mume leaves in vitro and in vivo for the first time.
Materials and methods
Chemical and reagents
Unless otherwise indicated, all chemicals used were purchased from Sigma Chemical Co. (St Louis, MO). AdipoRedTM Assay Reagent was obtained from Lonza Inc. (Walkersville, MD). Dexamethasone (DEX), 3-isobutyl-1-methylxanthine (IBMX) and rosiglitazone were also purchased from Sigma (St Louis, MO).
Plant and extract preparation
P. mume leaves were collected from Sancheong, Gyeongsangnam-do, South Korea during April and May 2013. Dr. Yong Joon Jeong identified the specimens and the voucher specimen of P. mume leaves (specimen number NMR-KR-14-00156) was deposited at the herbarium of Department of Life Science, Gachon University. We extracted the dried P. mume leaves (230 g) with 70% ethanol twice at room temperature for 24 h. The P. mume extract was then concentrated under reduced pressure at 40 °C using a rotary evaporator and stored at 4 °C until use. This crude extract (63.0 g) was suspended in distilled water and sequentially fractionated into n-hexane. The subsequent partition steps consecutively produced four fractions: dichloromethane (CH2Cl2), ethyl acetate (EtOAc), n-butanol (BuOH) and water ().
Figure 1. Extraction and fractionation of Prunus mume leaves (schematic diagram). CH2Cl2, dichloromethane-soluble fraction; EtOAc, ethyl acetate-soluble fraction; n-BuOH, butanol-soluble fraction.
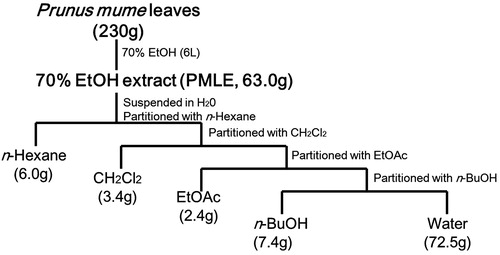
Figure 2. Effects of PMLE fractions on 3T3-L1 preadipocyte differentiation. 3T3-L1 preadipocytes were treated with the indicated concentrations of Prunus mume leaf extract fractions for 9 d. Differentiation was determined using the AdipoRed Assay as described in the Materials and methods. Bars represent means of relative values (% non-treated control) ± standard error of the mean.
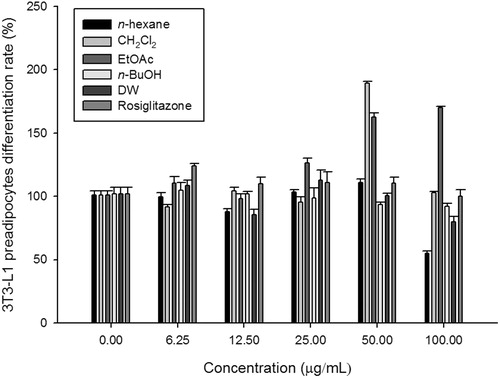
Determination of polyphenol content
The polyphenolic contents of the samples were determined using the Folin–Ciocalteu (FC) reagent method (Kaur & Kapoor Citation2002). Briefly, 200 μL of crude extract or solvent fraction (2 mg/mL) was made up to 3 mL with distilled water and then 0.5 mL of FC reagent was added. After mixing for 3 min, 2 mL of 20% (w/v) sodium carbonate was added and the mixture was allowed to stand for 60 min in the dark. The absorbance of the clear supernatant was measured at 700 nm using a spectrophotometer (LAMBDA 25 UV/Vis Spectrophotometer, PerkinElmer Inc., Waltham, MA). The polyphenol content in each sample was calculated based on a standard curve prepared using tannic acid (Sigma-Aldrich, St Louis, MO) and expressed as mg of tannic acid equivalent (TAE) per g of sample.
Determination of flavonoid content
Flavonoid content of both crude extracts and solvent fractions was determined using the aluminium chloride (AlCl3) colorimetric method (Chang et al. Citation2002). Briefly, 50 μL of crude extract or solvent fraction (2 mg/mL) was made up to 1 mL with methanol, mixed with 4 mL of distilled water and subsequently with 0.3 mL of 5% NaNO2 solution. After 5 min of incubation, 0.3 mL of 10% AlCl3 solution was added. The mixture was allowed to stand for 6 min, before adding 2 mL of 1 M NaOH solution. Water was added to the mixture to bring the final volume to 10 mL and the mixture was allowed to stand for 15 min. The absorbance was measured at 510 nm. The flavonoid content in each sample was calculated based on a standard curve, which was prepared using rutin (Sigma-Aldrich) and expressed as mg of rutin equivalent (RE) per g of sample.
Cell culture and adipocyte differentiation
Mouse 3T3-L1 preadipocytes were obtained from the American Type Culture Collection (Manassas, VA) and were grown in Dulbecco’s modified Eagle’s medium (DMEM) (HyClone, Logan, UT) supplemented with 10% fetal bovine serum (FBS, HyClone), 100 U/mL penicillin and 100 mg/mL streptomycin (HyClone) in an incubator with a humidified atmosphere of 5% CO2 at 37 °C. Upon reaching confluence, differentiation was initiated by incubating cells with DMEM containing 0.5 mM IBMX, 0.25 μM DEX and 10% FBS. After 48 h, the culture medium was changed to DMEM containing 10% FBS and test samples (0, 25, 50 and 100 μg/mL). Treatments lasted for 8 d with medium changes every 2 d. Differentiation of adipocytes was measured using AdipoRedTM Assay Reagent (Lonza, Walkersville, MD) according to the manufacturer’s protocol. Briefly, cells were fixed with 3% formaldehyde in PBS for 1 h at room temperature, washed three times with PBS and then stained with AdipoRedTM Assay Reagent for 10 min at room temperature. Fluorescence was measured with excitation at 485 nm and emission at 572 nm. Results were compared with those of untreated control cells and cells treated with 6.25 μM rosiglitazone (a TZD-class drug, positive control).
α-Glucosidase inhibition assay
α-Glucosidase inhibition activity was measured by mixing the following components sequentially: 250 μL potassium phosphate buffer (100 mM, pH 7.0), 150 μL 4-nitrophenyl-α-d-glucopyranoside (0.5 mM), 50 μg/mL plant extracts and 150 μL α-glucosidase (0.1 unit/mL in 10 mM potassium phosphate buffer). After incubating at 37 °C for 30 min, the reaction was stopped by the addition of 600 μL Na2CO3 (200 mM) and the absorbance was recorded at 400 nm.
Animals
Four-week-old male imprinting control region (ICR) mice (SLC Inc. Shizuoka, Japan) were housed in an air-conditioned animal room with a 12 h light/dark cycle at a temperature of 22 ± 1 °C and humidity of 50 ± 10%. Mice were provided a laboratory diet and water. All experimental protocols involving the use of animals were conducted in accordance with National Institutes of Health guidelines and approved by the Committee on Animal Care, Gachon University.
Induction of T2D and dyslipidaemia
After one week of acclimatization, mice were allowed to fast for 4 h, and were singly injected with either vehicle or low-dose streptozotocin (STZ, 100 mg/kg, i.p. in 0.1 M citrate-phosphate buffer, pH 4.5). After three weeks, vehicle-injected mice were randomly divided into two groups (n = 6 per group) and fed either a normal diet (CON) or a high-fat/high-cholesterol diet (HFD). The STZ-injected mice were also randomly divided into five groups (n = 6 per group) and fed ND (STZ) or HFD (PMLE 0) for three weeks. Subsequently, STZ/HFD mice were administered 5, 25 or 50 mg/kg PMLE for four weeks (PMLE 5, PMLE 25 and PMLE 50).
Measurement of serum glucose, total cholesterol and TG levels
At the end of the study, all animals were fasted for 6 h and blood was collected from the abdominal vena cava under anaesthesia with diethyl ether. Blood was centrifuged at 3000×g for 15 min at 4 °C to obtain serum. The serum concentrations of glucose, total cholesterol and TG were enzymatically measured using commercial kits (QuantiChromTM Glucose Assay Kit, EnzyChromTM Cholesterol Assay Kit and EnzyChromTM Triglyceride Assay Kit, BioAssay Systems, Hayward, CA).
Statistical analysis
Results are expressed as mean ± standard error of the mean. Differences between groups were determined by one-way analysis of variance followed by a modified t-test with Bonferroni correction for comparisons between individual groups; p < 0.05 and p < 0.01 were considered significant.
Results
Antidiabetic activity of P. mume EtOH extract
To evaluate antidiabetic activity of P. mume, α-glucosidase inhibition and adipocyte differentiation were measured in EtOH extracts of P. mume leaves. Treatment of 50 μg/mL PMLE inhibited 90.5% of α-glucosidase activity. We also found that 3T3-L1 cells treated with PMLE (25, 50 and 100 μg/mL) led to increase in adipocyte differentiation rates (118.2, 148.3 and 145.6%) compared with control. Adipocyte differentiation rate of positive control (rosiglitazone, 6.25 μg/mL) was 200.59%.
Comparison of antidiabetic effects between PMLE fractions
To determine the antidiabetic effects of each PMLE fraction, the IC50 of α-glucosidase inhibition and adipocyte differentiation were measured in each PMLE fraction ( and ). We were able to observe IC50 values of α-glucosidase inhibition using layers of EtOAc (68.2 ± 5.37 μg/mL) and n-BuOH (75.8 ± 18.76 μg/mL). Further, 3T3-L1 preadipocytes treated with 50 or 100 μg/mL of the EtOAc layer showed significantly increased cell differentiation rates compared with those of non-treated control cells ().
Table 1. α-Glucosidase inhibition activities of fractions from 70% ethanol extract of Prunus mume leaf.
Comparisons of yield, total polyphenol and total flavonoid contents between PMLE and its fractions
To determine the active components of P. mume leaves, PMLE was further fractionated into five layers: n-hexane, CH2Cl2, EtOAc, n-BuOH and water. We measured the phytochemical contents of each of the fractions as well as PMLE. The EtOAc layer exhibited the highest polyphenol and flavonoid content, whereas the n-hexane layer showed the lowest polyphenol and flavonoid content ().
Table 2. The yield, total polyphenol and total flavonoid contents of ethanol extract and various fractions of Prunus mume leaf ethanol extract.
Effects of PMLE on plasma glucose level, lipid profile and body weight in STZ/HFD mice
To confirm the effects of PMLE observed in vitro, an in vivo study was undertaken using T2D-induced mice. Compared with the control group, the groups with STZ/HFD-induced T2D showed elevated blood glucose levels. However, treatment with PMLE (5, 25 or 50 mg/kg) decreased blood glucose levels in a dose-dependent manner (). Further, compared with the controls, the groups with T2D showed significantly elevated serum total cholesterol and TG levels. PMLE administration also significantly reduced those levels. PMLE (50 mg/kg) treatment significantly decreased body weight compared with the STZ/HFD group. There was no significant difference in the food intake between STZ/HFD-treated groups and PMLE-treated STZ/HFD groups, consumed significantly more than the control group.
Table 3. Effects of Prunus mume leaf extract (PMLE) on plasma glucose, lipids and body weight in streptozotocin (STZ)/high-fat/high-cholesterol diet (HFD)-induced mice.
Discussion
T2D is a chronic metabolic disorder that causes economic burden due to its long-term complications. A number of studies have focused on the use of natural products to ameliorate high levels of blood glucose and insulin resistance (Newman & Cragg Citation2012). Fat metabolism is affected by insulin resistance, thereby worsening hyperglycaemia, dyslipidaemia and atherosclerosis (Pansuria et al. Citation2012). Synthetic antidiabetic drugs have several side effects, hence we present this discovery of regulatory agents of glucose levels and lipid metabolism from medicinal plants particularly important in the treatment of diabetes and other metabolic diseases (Hasani-Ranjbar et al. Citation2013).
The purpose of this study was to evaluate the antidiabetic effects of P. mume leaves using in vivo model and in vitro system. We chose P. mume leaves because the fruits of this plant have known antidiabetic effects (Shin et al. Citation2013), however the antidiabetic effects of P. mume leaves have not been investigated. In this study, we fractionated PMLE into five layers and found that the EtOAc layer exhibited the most profound effects on adipocyte differentiation and α-glucosidase inhibition. This enhanced α-glucosidase inhibition may be attributable to high levels of polyphenols extracted by EtOAc (). Polyphenols, a large group of compounds that have benzene rings with more than one hydroxyl group, are found widely in plants. Polyphenols range in structure from simple molecules (phenolic acids, phenylpropanoids and flavonoids) to highly polymerized compounds (lignin and tannins). Other studies have demonstrated that polyphenol-rich extracts of black tea, seaweed or Ascophyllum nodosum inhibit the activity of α-glucosidase (Pantidos et al. Citation2014; Striegel et al. Citation2015). In addition, studies have demonstrated that polyphenols and flavonoids affect carbohydrate metabolism. They inhibit carbohydrate digestion and glucose absorption in the intestine and stimulate insulin secretion from the pancreatic β-cells. Polyphenols and flavonoids also modulate glucose release from liver, hepatic glucose output, activation of insulin receptors and glucose uptake in the insulin-sensitive tissues (Hanhineva et al. Citation2010).
PPARγ is involved in carbohydrates and lipid metabolism, and improves insulin sensitivity. Therefore, many researchers have attempted to discover plant ingredients with PPARγ agonist activity for development as potential antidiabetic therapies (Wang et al. Citation2014). Shin et al. (Citation2013) showed that treatment with P. mume fruits increases transcriptional activity and expression of PPARγ. As expected, we found that the ethanol extract of P. mume leaves effectively stimulated adipocyte differentiation. Therefore, we speculated that P. mume leaves have PPARγ agonist activity.
To support the findings of our in vitro experiments, PMLE (0, 5, 25 or 50 mg/kg) was administered to STZ/HFD mice. Several reports have shown that this model displays insulin resistance and mildly impaired insulin secretion, both of which are distinct characteristics of the metabolic disturbances observed in patients with T2D (Zhang et al. Citation2008; Koo et al. Citation2014). The present study suggests that PMLE has potent hypoglycaemic and hypolipidaemic activities in STZ/HFD-induced T2D.
To our knowledge, this is the first study to report the antidiabetic effects of P. mume leaves in vitro and in vivo. To understand the mechanisms by which P. mume leaves modulate blood glucose level and blood lipid profiles, future research should study how PMLE affects the activity and expression of PPARγ and glucose transporter-4. This work should analyse the flavonoid profiles and components of PMLE and its fractions using high performance liquid chromatography.
Conclusions
In conclusion, the novel antidiabetic plant material, P. mume leaves, was evaluated for their α-glucosidase inhibition and adipocyte differentiation activities. The antidiabetic effects of P. mume leaves may be attributable to the presence of significant amount of polyphenols and flavonoids. In mice, high levels of phytoconstituents in P. mume leaves ameliorated the increase in body weight, total cholesterol and TG induced by STZ injection and high-fat diet consumption.
Disclosure statement
The authors report no conflicts of interest. The authors alone are responsible for the content and writing of this article.
References
- Alberti K, Zimmet P. 1998. Definition, diagnosis and classification of diabetes mellitus and its complications. Part 1: Diagnosis and classification of diabetes mellitus provisional report of a WHO consultation. Diabetic Med. 15:539–553.
- Bermudez V, Finol F, Parra N, Parra M, Perez A, Penaranda L, Vilchez D, Rojas J, Arraiz N, Velasco M. 2010. PPAR-gamma agonists and their role in type 2 diabetes mellitus management – agonists their in 2 mellitus. Am J Ther J. 17:274–283.
- Chang C-C, Yang MH, Wen HM, Chern JC. 2002. Estimation of total flavonoid content in propolis by two complementary colorimetric methods. J Food Drug Anal. 10:178–182.
- Deshpande MC, Venkateswarlu V, Babu RK, Trivedi RK. 2009. Design and evaluation of oral bioadhesive controlled release formulations of miglitol, intended for prolonged inhibition of intestinal α-glucosidases and enhancement of plasma glucagon like peptide-1 levels. Int J Pharmaceutics. 380:16–24.
- Fu J, Prasad HC. 2014. Changing epidemiology of metabolic syndrome and type 2 diabetes in Chinese youth. Curr Diab Rep. 14:1–10.
- Gruenwald J, Freder J, Armbruester N. 2010. Cinnamon and health. Crit Rev Food Sci Nutr Rev Sci. 50:822–834.
- Hanhineva K, Törrönen R, Bondia-Pons I, Pekkinen J, Kolehmainen M, MykkänenH, Poutanen K. 2010. Impact of dietary polyphenols on carbohydrate metabolism. Int J Mol Sci. 11:1365–1402.
- Hasani-Ranjbar S, Jouyandeh Z, Abdollahi M. 2013. A systematic review of anti-obesity medicinal plants – an update. J Diabetes Metab Disord. 12:28.
- He W, Barak Y, Hevener A, Olson P, Liao D, Le J, Nelson M, Ong E, Olefsky JM, Evans RM. 2003. Adipose-specific peroxisome proliferator-activated receptor γ knockout causes insulin resistance in fat and liver but not in muscle. Proc Natl Acad Sci U S A. 100:15712–15717.
- Kaur C, Kapoor HC. 2002. Anti-oxidant activity and total phenolic content of some Asian vegetables. Int J Food Sci Tech. 37:153–161.
- Koo HJ, Kwak JH, Kang SC. 2014. Anti-diabetic properties of Daphniphyllum macropodum fruit and its active compound. Biosci Biotechnol Biochem Biotech. 78:1392–1401.
- Krentz AJ, Bailey CJ. 2005. Oral antidiabetic agents: current role in type 2 diabetes mellitus. Drugs. 65:385–411.
- Lin Y, Sun Z. 2010. Current views on type 2 diabetes. J Endocrinol. 204:1–11.
- Martens FMAC, Visseren FLJ, Lemay J, de Koning EJP, Rabelink TJ. 2002. Metabolic and additional vascular effects of thiazolidinediones. Drugs. 62:1463–1480.
- Modak M, Dixit P, Londhe J, Ghaskadbi S, Devasagayam TPA. 2007. Indian herbs and herbal drugs used for the treatment of diabetes. J Clin Biochem Nutr. 40:163–173.
- Newman DJ, Cragg GM. 2012. Natural products as sources of new drugs over the 30 years from 1981 to 2010. J Nat Prod. 75:311–335.
- Norisada N, Masuzaki H, Fujimoto M, Inoue G, Hosoda K, Hayashi T, Watanabe M, Muraoka S, Yoneda F, Nakao K. 2004. Antidiabetic and adipogenic properties in a newly synthesized thiazolidine derivative, FPFS-410. Metab Clin Exp. 53:1532–1537.
- Pansuria M, Xi H, Li L, Yang X-F, Wang H. 2012. Insulin resistance, metabolic stress, and atherosclerosis. Front Biosci (Schol Ed). 4:916–931.
- Pantidos N, Boath A, Lund V, Conner S, McDougall GJ. 2014. Phenolic-rich extracts from the edible seaweed, Ascophyllum nodosum, inhibit α-amylase and α-glucosidase: potential anti-hyperglycemic effects. J Funct Foods. 10:201–209.
- Shin EJ, Hur HJ, Sung MJ, Park JH, Yang HJ, Kim MS, Kwon DY, Hwang J-T. 2013. Ethanol extract of the Prunus mume fruits stimulates glucose uptake by regulating PPAR-γ in C2C12 myotubes and ameliorates glucose intolerance and fat accumulation in mice fed a high-fat diet. Food Chem. 141:4115–4121.
- Striegel L, Kang B, Pilkenton SJ, Rychlik M, Apostolidis E. 2015. Effect of black tea and black tea pomace polyphenols on α-glucosidase and α-amylase inhibition, relevant to type 2 diabetes prevention. Front Nutr. 2:1–6.
- U. K. Prospective Diabetes Study Group. 1998. Intensive blood-glucose control with sulphonylureas or insulin compared with conventional treatment and risk of complications in patients with type 2 diabetes (UKPDS 33). Lancet. 352:837–853.
- Utsunomiya H, Takekoshi S, Gato N, Utatsu H, Motley ED, Eguchi K, Fitzgerald TG, Mifune M, Frank GD, Eguchi S. 2002. Fruit-juice concentrate of Asian plum inhibits growth signals of vascular smooth muscle cells induced by angiotensin II. Life Sci. 72:659–667.
- Utsunomiya H, Yamakawa T, Kamei J, Kadonosono K, Tanaka S-I. 2005. Anti-hyperglycemic effects of plum in a rat model of obesity and type 2 diabetes, Wistar fatty rat. Biomed Res. 26:193–200.
- Wang L, Waltenberger B, Pferschy-Wenzig E-M, Blunder M, Liu X, Malainer C, Blazevic T, Schwaiger S, Rollinger JM, Heiss EH. 2014. Natural product agonists of peroxisome proliferator-activated receptor gamma (PPARγ): a review. Biochem Pharmacol. 92:73–89.
- WHO. 2014. Global health estimates: deaths by cause, age, sex and country, 2000–2012. Geneva: WHO.
- Wilding J. 2006. Thiazolidinediones, insulin resistance and obesity: finding a balance. Int J Clin Pract. 60:1272–1280.
- Zhang M, Lv X-Y, Li J, Xu Z-G, Chen L. 2008. The characterization of high-fat diet and multiple low-dose streptozotocin induced type 2 diabetes rat model. Exp Diabetes Res. 2008:Article ID 704045, 9 pages. doi:10.1155/2008/704045.