Abstract
Context: Medical therapies for alcohol-induced liver disease are often difficult to handle and limited in efficacy.
Objective: In an attempt to find natural therapeutics, here, we investigate the preventive effect of persimmon vinegar (PV) and its fractions against alcohol-induced hepatic injury, in addition to the underlying mechanism, in rats chronically administered with alcohol.
Materials and methods: Forty male Wistar rats were randomized into five groups (n = 8 per group); normal control (NC), ethanol control (EC), ethanol + PV, ethanol + water-insoluble PV fraction (PI) and ethanol + water-soluble PV fraction (PS). PV, PI or PS was orally administrated at the level of 100 mg/kg B.W by oral gavage every day for 4 weeks prior to ethanol administration. The liver sections were stained with hematoxylin & eosin and gene expression was assessed by real-time polymerase chain reaction.
Results: After a 4-week treatment, histological observation revealed that PV and its fractions mitigated alcohol-induced changes in the liver. CYP2E1 expression was significantly increased in the EC group compared with the NC group, but was significantly suppressed in the PV group compared with the EC group (p = 0.044). We also found significant decreases in hepatic mRNA expression of interleukin (IL)-1β, IL-12β, toll-like receptor (TLR)-4 and cyclooxygenase (COX)-2 in the PV-, PI- and PS-treated groups compared with those of the EC group.
Discussion and conclusion: Taken together, the present findings suggest that PV and its fractions hold great promise as natural remedies with anti-inflammatory activities that alleviate alcohol-induced liver damage.
Introduction
Chronic alcohol consumption is a leading etiological factor in chronic liver disease worldwide, causing fatty liver, alcoholic hepatitis, cirrhosis and hepatocellular carcinoma (Williams Citation2006). Alcoholic liver disease may not only be caused by nutritional disturbance but also by direct toxicity because alcohol is dominantly metabolized in the liver. Hepatocytes contain three main enzyme systems for alcohol metabolism, alcohol dehydrogenase (ADH), microsomal ethanol oxidizing system (MEOS) and catalase (Lieber Citation2005).
Cytochrome P450 2E1 (CYP2E1) is a key enzyme of MEOS, which was found to be inducible by chronic alcohol consumption and is referred to as an adaptive pathway. The induction of CYP2E1 has been shown to play a key role in the pathogenesis of alcoholic liver injury because of the oxidative stress it generates (Lieber Citation2004). The finding that hepatic CYP2E1 protein and mRNA were enhanced in the liver biopsies of alcoholic patients is considerable evidence to support this notion (Tsutsumi et al. Citation1989; Takahashi et al. Citation1993). The oxidative stress caused by excessive induction of CYP2E1 also promotes inflammation, which is aggravated by an increase in the pro-inflammatory cytokine, tumour necrosis factor-α (TNF-α), in Kupffer cells (Khan et al. Citation2011). The parenchymal cells of the liver also change their response to TNF-α, initiating a battery of intracellular pathways that lead to cell death, and activating cells to be more sensitive to the cytotoxic actions of TNF-α (Hoek & Pastorino Citation2002).
There has been a growing interest in elucidating the role of foods and food components as suppressors of alcohol-induced oxidative stress and liver damage, but with little success (Neuman Citation2003). Persimmon vinegar (PV) is produced by the fermentation of persimmon fruit, and, therefore, contains various bioactive compounds that can be found in persimmon, as well as useful substances produced by the microorganisms involved in the fermentation process. According to previous studies, PV decreased alcohol-induced fat deposition in the liver and improved alcohol clearance in rats (Moon & Cha Citation2008). The effects of PV on alcohol and fat metabolism have also been confirmed in humans (Kim Citation1999). However, little work has been performed to verify the underlying mechanism of the physiological signals. The present study was conducted to elucidate the underlying molecular mechanism leading to the protection against chronic alcohol-induced liver injury in rats. Furthermore, to gain insight as to the component of PV responsible for the observed effect, we compared the hepatoprotective activities of PV and its fractions.
Materials and methods
Chemicals and reagents
Ethyl alcohol, formalin solution, xylene, paraffin and potassium chloride were purchased from Sigma-Aldrich (St. Louis, MO).
Preparation of PV and its fractions
PV, and its water-soluble (PS) and water-insoluble fractions (PI), were provided by Sempio Food Company (Seoul, Korea). Briefly, PV was evaporated to a concentrate, precipitated with absolute ethanol at 25 °C overnight, and centrifuged at 18,000 rpm for 10 min. The pellet (PI) was dried at 70 °C and the supernatant (PS) was evaporated to eliminate the ethanol. Total polyphenol content was measured using the Folin–Coicalteu colorimetric method, described previously by Singleton et al. (Citation1999), with the following results: 3.39, 5.19 and 23.90 g/L for PT, PI and PS, respectively.
Animal model and treatments
Six-week-old male Wistar rats were purchased from Central Lab Animal Inc. (Seoul, Korea) and acclimatized to the laboratory setting (23 ± 1 °C, 45 ± 5% humidity) with free access to a commercial pellet diet (Purina Inc., Gyeonggi-Do, Korea) and tap water for 7 d. The animals were cared for in accordance with the Guide for the Care and Use of Laboratory Animals, and the study protocol was approved by the Institutional Animal Care and Use Committee (IACUC approval no. 2012-01-077) of Ewha Women’s University (Seoul, Korea).
The rats were randomly assigned into five groups based on body weight (n = 8 per group); normal control (NC), ethanol control (EC), ethanol + PV (PV), ethanol + PI (PI) and ethanol + PS (PS). Ethanol (50%, v/v) was orally administered every day with a weekly increase in dose (2.5, 4, 5 and 6 mL/kg BW, per week) for 4 weeks. PV, PI or PS was also orally administrated at the level of 100 mg/kg BW by oral gavage every day prior to ethanol administration, for the same period of time.
Liver enzyme chemistry and histological observation
The serum samples were analyzed for alanine aminotransferase (ALT) and aspartate aminotransferase (AST) in a biochemical analyzer (7180, HITACHI, Tokyo, Japan). Small sections of fresh liver tissue were immediately fixed in 10% phosphate-buffered formalin solution. The formalin-fixed liver tissue was dehydrated in a 70–100% gradient of ethyl alcohol, dealcoholized in xylene, embedded in paraffin and cut to 5 μm sections. The liver sections were deparaffinized in xylene, rehydrated in a reverse-gradient series of ethyl alcohol and stained with hematoxylin and eosin (H&E).
Preparation of the liver microsomal fraction
Liver tissue was homogenized in 1.15% potassium chloride buffer (pH 7.4), and the homogenate was centrifuged at 1900 rpm for 10 min at 4 °C. The supernatant was then centrifuged at 9000 rpm for 20 min at 4 °C to precipitate the mitochondrial fraction and the resulting supernatant was subsequently centrifuged at 120,000 rpm for 1 h at 4 °C to pellet the microsomal fraction.
Western blotting analysis of CYP2E1
Microsomal protein was quantified spectrophotometrically by the Lowry assay using bovine serum albumin as a standard. Total cell lysates were fractionated by size using 12% SDS-PAGE and electrophoretically transferred onto polyvinylidene difluoride membranes (Bio-Rad, Hercules, CA). To detect the CYP2E1 and β-actin proteins, the membranes were incubated with primary monoclonal rabbit anti-CYP2E1 antibody (1:1000, Abcam, Cambridge, MA) and primary monoclonal mouse anti-β-actin antibody (1:500, Santa Cruz Biotechnology, Inc., Santa Cruz, CA). After washing in PBS, the membranes were incubated with anti-rabbit or anti-mouse horseradish peroxidase-labeled secondary antibodies (1:2000, Santa Cruz Biotechnology, Inc., Santa Cruz, CA) in 5% BSA in TBS-T for 2 h at room temperature. The blotted membranes were visualized by WEST-ZOL® plus Western blotting detection system (iNtRON Biotechnology, Inc., Seoul, Korea), and the immunoreactive bands were quantified by the ChemiDoc XRS + system with Image Lab software (Bio-Rad laboratories, Hercules, CA).
Quantitative real-time PCR assay
Total RNA was isolated from the liver homogenate using TRIzol (Invitrogen, Carlsbad, CA). Single-strand cDNA was synthesized using the High Capacity RNA-to-cDNA kit (Applied Biosystems, Foster City, CA). Quantitative RT-PCR was performed using the TaqMan method with the Step-One-Plus RT-PCR System (Applied Biosystems, Foster City, CA). Expression studies were carried out using gene-specific primers for β-actin, interleukin (IL)-1β, IL-12β, cluster of differentiation (CD)-14, toll-like receptor (TLR)-4 and cyclooxygenase (COX)-2 that were obtained from Applied Biosystems (Foster City, CA) (identifications are as follows: Rn00667869, Rn00580432_m1, Rn00575112_m1, Rn00572656_g1, Rn00569848_m1 and Rn01483828_m1). The amplification was performed starting with a 10 min template denaturation step at 95 °C, which was followed by 40 cycles at 95 °C for 15 s and 60 °C for 1 min. The relative amounts of mRNA were normalized to the amount of β-actin, and the relative amount of all RNAs was calculated using the comparative CT Method. All data are expressed as a relative quantity to each control value.
Statistical analysis
All results are expressed as the mean ± standard error (SE). All statistical calculation was performed using the SAS 9.3 program for Windows (SAS Institute, Cary, NY). The differences among all groups were analyzed by a one-way analysis of variance (ANOVA) with a post hoc Duncan’s multiple comparison test. Differences were considered statistically significant at a value of p < 0.05.
Results
Effects of PV, PI, and PS on body weight and food intake
After 4 weeks, rats treated with alcohol showed a slight decrease in body weight and a significant decrease in food intake compared with the control group (). There were no significant differences in the initial and final body weights among all the groups, or in food consumption among all groups with normal growth.
Effects of PV, PI, and PS on hepatic morphology
While the liver tissue of the NC group showed normal hepatic architecture (), the administration of alcohol for 4 weeks induced macro- and micro-vesicular steatoses (). Interestingly, it was found that the administration of PV (), PI () and PS () alleviated alcohol-induced liver damage. However, none of PV, PI, and PS treatment altered the activities of ALT and AST (data not shown).
Figure 2. Representative photomicrographs of liver tissues in the control and experimental rats. (A) NC, normal control, water; (B) EC, water + ethanol-treated control; (C) PV, total concentrate of PV (100 mg/kg BW) + ethanol; (D) PI, ethanol-insoluble fraction of PV (100 mg/kg BW) + ethanol; and (E) PS, ethanol-soluble fraction of PV (100 mg/kg BW) + ethanol. The liver sections of rats from each group was stained using haematoxylin and eosin (H&E), and the images were examined by light microscopy. The arrows indicate steatosis.
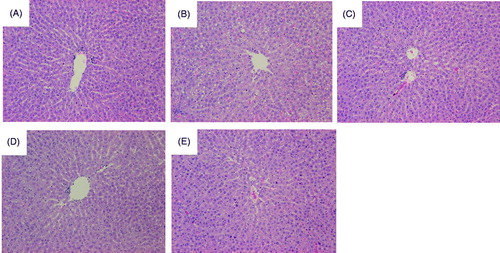
Effects of PV, PI, and PS on alcohol-induced CYP2E1 gene expression
Chronic ethanol administration resulted in significant increases of cytochrome P450 2E1 (CYP2E1) protein synthesis in the liver (). The CYP2E1 expression in the EC group was significantly increased compared with that of the NC group. Conversely, PV supplementation significantly decreased the CYP2E1 protein synthesis (p = 0.044). Similarly, both PI and PS treatment had tendencies to decrease CYP2E1 expression compared with that of the EC group, but there were no significant differences among these groups.
Figure 3. Effects of persimmon vinegar and its fractions on hepatic cytochrome P450 2E1 (CYP2E1) expression in alcohol-administered rats. Wistar rats were pretreated with PV and its fractions by gavage feeding for 4 weeks before ethanol administration. NC, normal control, water; EC, water + 50% ethanol-treated control; PV, 100 mg/kg BW total concentrate of PV + 50% ethanol; PI, 100 mg/kg BW ethanol-insoluble fraction of PV + 50% ethanol; PS, 100 mg/kg BW ethanol-soluble fraction of PV + 50% ethanol. Results represent the mean ± SE from three independent experiments. Values with different letters within the row are significantly different at p < 0.05 levels by a Duncan’s multiple range test.
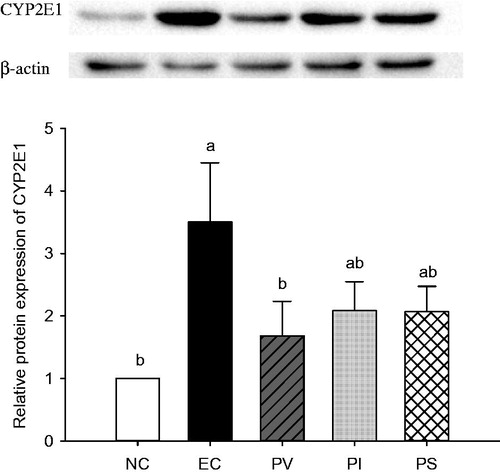
Effects of PS, PI, and PS on inflammatory mediator mRNA expression
The hepatic mRNA expression levels of IL-1β, IL-12β, TLR4 and COX-2 were measured by quantitative RT-PCR analyses to determine the ethanol-induced changes in the transcriptional levels of inflammatory mediators in the liver. With respect to the transcriptional level of IL-1β, the EC group had a tendency to increase compared with the NC group, while the PI and PS groups were significantly decreased as compared with the EC group (p = 0.008, ). The IL-12β mRNA level in the liver of the EC group was significantly increased compared with the NC group, whereas there were significant decreases in the PV, PI and PS groups (p = 0.001, ). The mRNA expression of TLR4 in the EC group was significantly increased compared with the NC group, and showed significant decreases in the PI and PS groups as compared with the EC group (p = 0.014, ). The COX-2 mRNA synthesis in the liver of the EC group was markedly increased compared with the NC group, while that of the PV, PI and PS groups were significantly decreased as compared with the EC group (p = 0.0003, ).
Figure 4. Effects of persimmon vinegar and its fractions on hepatic mRNA expression of (A) IL-1β, (B) IL-12β, (C) TLR4 and (D) COX-2 in alcohol-administered rats. Wistar rats were pretreated with PV and its fractions by gavage feeding for 4 weeks before ethanol administration. NC, normal control, water; EC, water + 50% ethanol treated control; PV, 100 mg/kg BW total concentrate of PV +50% ethanol; PI, 100 mg/kg BW ethanol-insoluble fraction of PV + 50% ethanol; PS, 100 mg/kg BW ethanol-soluble fraction of PV + 50% ethanol. Results represent the mean ± SE from three independent experiments. Values with different letters within the row are significantly different at p < 0.05 levels by a Duncan’s multiple range test.
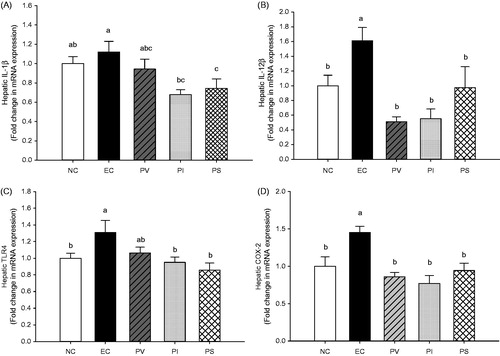
Discussion
The importance of ethanol-mediated oxidative stress in the pathogenesis of alcoholic liver disease (ALD) is now well recognized (Albano Citation2008). Chronic alcohol exposure increases the generation of reactive oxygen species (ROS) including hydroxyl radicals (•OH−), superoxide (•O2−) and hydrogen peroxide (H2O2). It also triggers a decrease in cellular antioxidant levels, which causes oxidative stress in many tissues, especially in the liver (Wu & Cederbaum Citation2003). Among many potential sources of ROS in response to alcohol exposure, CYP2E1 is considered to be a major contributor to ROS production (Ding et al. Citation2012). Therefore, the present study aimed to investigate whether PV and its fractions exerted any direct effect on the major enzyme involved in ethanol metabolism. The removal of alcohol is mostly dependent on the oxidation of alcohol and its metabolic byproduct via alcohol dehydrogenase (ADH), CYP2E1 and aldehyde dehydrogenase (ALDH) (Lieber Citation2004). Although initial alcohol exposure has a major impact on the hepatic redox state as a result of ethanol metabolism by ADH, repeated exposure to ethanol involves ethanol metabolism via CYP2E1 (Park et al. Citation2013). During the catalytic cycle of CYP2E1, significant amounts of ROS are generated, which induce oxidant stress and cellular damage (Leung & Nieto Citation2013). The free radicals induced by ethanol lead to lipid peroxidation, resulting in the damage of membrane lipids, proteins and DNA (Masalkar & Abhang Citation2005). The results of the present study show that ethanol-induced overexpression of CYP2E1 was significantly inhibited by PV treatment ().
The most favoured current hypothesis suggests that the immune response related to ALD is a consequence of the alcohol-induced increase in the absorption of gut-derived endotoxin (Vidali et al. Citation2008). Activated kupffer cells in the liver then release pro-inflammatory cytokines (TNF-α, IL-1β, IL-6 and IL-12β), reactive oxygen species (ROS) and nitric oxide (NO) (Albano Citation2008). These cytokines and chemokines lead to the intrahepatic recruitment and activation of granulocytes that are found characteristically in acute severe alcoholic liver inflammation (Hu et al. Citation2009). Specifically, the production of pro-inflammatory cytokines from Kupffer cells is stimulated by the activation of oxidant-sensitive NF-κB (Arteel Citation2003). Kupffer cells play a key role in the hepatic inflammation in ALD. Gut-derived endotoxin, a trigger of inflammation, binds to LPS-binding protein after entering the portal circulation, and this complex protein is recognized by TLR4 and its co-receptor, CD14, leading to the activation of TLR4-mediated downstream pathways (Ding et al. Citation2012). There is growing evidence to suggest that the LPS-TLR4-signalling pathway plays a critical role in ethanol-induced liver injury (Uesugi et al. Citation2001; Hritz et al. Citation2008). The present study indicates that hepatic mRNA expression of TLR4 is markedly increased in ethanol-administered rats, whereas PI and PS administration attenuates the ethanol-induced TLR4 mRNA expression ().
IL-1β activates macrophages to generate a full range of inflammatory responses including neutrophil infiltration, and its activation promotes liver inflammation in ALD (Wang et al. Citation2012). From our present findings, PI and PS administration significantly decreased the transcriptional level of hepatic IL-1β as compared with that of the EC group (). In addition, the mRNA expression of hepatic IL-12β was significantly decreased in all the PV, PI and PS groups as compared with the EC group ().
Endotoxin and lipid peroxidation promote ALD by upregulating COX-2 in Kupffer cells. Increased COX-2 expression results in enhanced production of pro-inflammatory eicosanoids, which may contribute to liver injury (Nanji et al. Citation1997). In the present study, hepatic mRNA expression of COX-2 in the EC group was significantly increased compared with the NC group. Conversely, PV, PI and PS administration significantly alleviated the ethanol-induced expression of COX-2 in the liver ().
Taken together, the effects of PV and its fractions on the enzyme activity involved in alcohol metabolism and hepatic inflammatory mediators were confirmed in chronic alcohol-administered rats. These findings may help to explain the underlying mechanisms for the hepatoprotective actions of PV and its fractions at the molecular level.
Conclusion
In conclusion, the hepatoprotective effects of PV and its fractions against alcohol-induced liver damage are attributed to the following: (1) mitigation of alcohol-induced hepatic steatosis, (2) downregulation of CYP2E1 expression, and (3) suppression of gene expression of a variety of inflammatory mediators.
Funding information
This study was supported by the Korean Institute of Planning & Evaluation for Technology in Food, Agriculture, Forestry, & Fisheries (IPET) and the Ministry of Science, ICT, & Future Planning (NRF 2012M3A9C4048761). The funding sources had no involvement in the collection, analysis, or interpretation of the data, the writing of this report, or the decision to submit this manuscript for publication.
Acknowledgements
The authors would like to thank the Sempio Food Company (Seoul, Korea) for their gift of PT, PI and PS.
Disclosure statement
The authors report that they have no conflicts of interest.
References
- Albano E. 2008. Oxidative mechanisms in the pathogenesis of alcoholic liver disease. Mol Aspects Med. 29:9–16.
- Arteel GE. 2003. Oxidants and antioxidants in alcohol-induced liver disease. Gastroenterology. 124:778–790.
- Ding RB, Tian K, Huang LL, He CW, Jiang Y, Wang YT, Wan JB. 2012. Herbal medicines for the prevention of alcoholic liver disease: a review. J Ethnopharmacol. 144:457–465.
- Hoek JB, Pastorino JG. 2002. Ethanol, oxidative stress, and cytokine-induced liver cell injury. Alcohol. 27:63–68.
- Hritz I, Mandrekar P, Velayudham A, Catalano D, Dolganiuc A, Kodys K, Kurt-Jones E, Szabo G. 2008. The critical role of toll-like receptor (TLR) 4 in alcoholic liver disease is independent of the common TLR adapter MyD88. Hepatology. 48:1224–1231.
- Hu S, Yin S, Jiang X, Huang D, Shen G. 2009. Melatonin protects against alcoholic liver injury by attenuating oxidative stress, inflammatory response, and apoptosis. Eur J Pharmacol. 616:287–292.
- Khan AQ, Nafees S, Sultana S. 2011. Perillyl alcohol protects against ethanol induced acute liver injury in Wistar rats by inhibiting oxidative stress, NFκ-B activation and proinflammatory cytokine production. Toxicology. 279:108–114.
- Kim K. 1999. Effects of sport-drink with persimmon vinegar on ethanol oxidation and recovery of physiological function. Exerc Sci. 8:495–505.
- Leung TM, Nieto N. 2013. CYP2E1 and oxidant stress in alcoholic and non-alcoholic fatty liver disease. J Hepatol. 58:395–398.
- Lieber CS. 2004. Alcoholic fatty liver: its pathogenesis and mechanism of progression to inflammation and fibrosis. Alcohol. 34:9–19.
- Lieber CS. 2005. Metabolism of alcohol. Clin Liver Dis. 9:1–35.
- Masalkar PD, Abhang SA. 2005. Oxidative stress and antioxidant status in patients with alcoholic liver disease. Clin Chim Acta. 355:61–65.
- Moon YJ, Cha YS. 2008. Effects of persimmon-vinegar on lipid metabolism and alcohol clearance in chronic alcohol-fed rats. J Med Food. 11:38–45.
- Nanji AA, Miao L, Thomas P, Rahemtulla A, Khwaja S, Zhao S, Peters D, Tahan SR, Dannenberg AJ. 1997. Enhanced cyclooxygenase-2 gene expression in alcoholic liver disease in the rat. Gastroenterology. 112:943–951.
- Neuman MG. 2003. Cytokines-central factors in alcoholic liver disease. Alcohol Res Health. 27:307–316.
- Park HY, Ha SK, Eom H, Choi I. 2013. Narirutin fraction from citrus peels attenuates alcoholic liver disease in mice. Food Chem Toxicol. 55:637–644.
- Singleton VL, Orthofer R, Lamuela-Raventos RM. 1999. Methods in enzymology. San Diego (CA): Academic Press.
- Takahashi T, Lasker JM, Rosman AS, Lieber CS. 1993. Induction of cytochrome P-4502E1 in the human liver by ethanol is caused by a corresponding increase in encoding messenger RNA. Hepatology. 17:236–245.
- Tsutsumi M, Lasker JM, Shimizu M, Rosman AS, Lieber CS. 1989. The intralobular distribution of ethanol-inducible P450IIE1 in rat and human liver. Hepatology. 10:437–446.
- Uesugi T, Froh M, Arteel GE, Bradford BU, Thurman RG. 2001. Toll-like receptor 4 is involved in the mechanism of early alcohol-induced liver injury in mice. Hepatology. 34:101–108.
- Vidali M, Stewart SF, Albano E. 2008. Interplay between oxidative stress and immunity in the progression of alcohol-mediated liver injury. Trends Mol Med. 14:63–71.
- Wang HJ, Gao B, Zakhari S, Nagy LE. 2012. Inflammation in alcoholic liver disease. Annu Rev Nutr. 32:343–368.
- Williams R. 2006. Global challenges in liver disease. Hepatology. 44:521–526.
- Wu D, Cederbaum AI. 2003. Alcohol, oxidative stress, and free radical damage. Alcohol Res Health. 27:277–284.