Abstract
A series of Co(II), Ni(II), and Cu(II) complexes of the type ML.2H2O have been synthesized with Schiff bases derived from 8-formyl-7-hydroxy-4-methylcoumarin and o-phenylenediamine/ethylenediamine. The structure of the complexes has been proposed in the light of analytical, spectral (IR, UV-Vis, ESR, and FAB-mass), magnetic, thermal, and fluorescence studies. The complexes are soluble in DMF and DMSO. The measured molar conductance values indicate that the complexes are non-electrolytes in nature. In view of IR, UV-Vis, and magnetic studies, it has been concluded that all the complexes possess octahedral geometry, in which ligand is coordinated to metal ion through the azomethine nitrogen and phenolic oxygen via deprotonation. Thermal studies provide useful information about the coordination of water molecules to the metal ion and the stability of the complexes. The redox behavior of the complexes has been investigated by an electrochemical method using cyclic voltammetry. The Schiff bases and their metal complexes have been screened for their antibacterial (Escherichia coli, Staphylococcus aureus, Pseudomonas aeruginosa, and Salmonella typhi) and antifungal activities (Aspergillus niger, Aspergillus flavus, and Cladosporium) by the minimum inhibitory concentration method. DNA binding with Co(II) and Cu(II) complexes has been studied by the agarose gel electrophoresis method.
Introduction
Coumarin is structurally the least complex member of a large class of compounds known as benzopyronesCitation1. Coumarin derivatives possess a wide range of biological activities, viz. antithromboticCitation2, antimicrobialCitation3, antiallergicCitation4, antiinflammatoryCitation5, antitumorCitation6, and anticoagulantCitation7, etc. Recently, coumarin derivatives have been evaluated in the treatment of human immunodeficiency virus (HIV), due to their ability to inhibit HIV integraseCitation8,Citation9. In vitro and in vivo studies have suggested the possible use of coumarins in the treatment of cancerCitation10. The coumarin nucleus is also present in the novobiocin, clorobiocin, and coumermycin A1 antibiotics. These antibiotics are active against methicillin-resistant Staphylococcus aureusCitation3 and are potent catalytic inhibitors of DNA gyrase. The interaction of transition metal complexes with DNA has been extensively studied for their usage as probes for DNA structure and their potential application in chemotherapyCitation11–13. One of the important DNA-related activities of transition metal complexes is that some of the complexes show the ability to cleave DNA. Very recently, Cu(II) complexes have been reported to be active in DNA strand scissionCitation14–16.
Such a wide spectrum of biological application of coumarins prompted us to synthesize some of the transition metal complexes with coumarin Schiff bases. Very recently, metal complexes with coumarin Schiff bases possessing good antimicrobial activity have been reported from our laboratoryCitation17,Citation18.
Thus, in continuation with our earlier work, we report here the antimicrobial activity and DNA cleavage property of Co(II), Ni(II), and Cu(II) metal complexes with newly synthesized Schiff bases derived from 8-formyl-7-hydroxy-4-methylcoumarin and o-phenylenediamine/ethylenediamine (Scheme 1). The Schiff bases and their metal complexes have been characterized by various spectral and analytical techniques.
Experimental
Physical measurements
Carbon, hydrogen, and nitrogen were estimated using a Carlo Erba EA1108 elemental analyzer. The infrared (IR) spectra of the Schiff bases and their Co(II), Ni(II), and Cu(II) complexes were recorded on a Hitachi 270 IR spectrophotometer in the 4000– 250 cm−1 region using a KBr disk. The electronic spectra of the complexes were recorded in high performance liquid chromatography (HPLC) grade dimethylformamide (DMF) and dimethylsulfoxide (DMSO) solvents on a Varian Cary 50-Bio ultraviolet (UV) spectrophotometer in the region of 200–1100 nm. The 1H nuclear magnetic resonance (NMR) and 13C NMR spectra of the Schiff bases were recorded in CDCl3 on a Bruker 300 MHz spectrometer at room temperature, using tetramethylsilane (TMS) as an internal reference. The electron spin resonance (ESR) spectrum was recorded on a Varian E-4X band spectrometer, and the field was set at 3000 G at a modulation frequency of 100 kHz under liquid nitrogen temperature using tetracyanoethylene (TCNE) as g-marker. Fast atom bombardment (FAB)-mass spectra were recorded on a Jeol SX 102/DA-6000 mass spectrometer/data system using argon/xenon (6 kV, 10 A) as the FAB gas. The accelerating voltage was 10 kV, and the spectra were recorded at room temperature and m-nitrobenzyl alcohol was used as the matrix. The mass spectrometer was operated in the positive ion mode. Fluorescence studies of Schiff bases and their metal complexes were recorded on a Hitachi F-7000 fluorescence spectrophotometer (Japan). Solutions (10−3 M concentration) were prepared in HPLC grade DMF and DMSO solvents and the experiment was carried out at room temperature. The electrochemistry of Cu(II) complexes was studied on a CH Instruments 1110A electrochemical analyzer (USA) in DMF containing 0.05 M n-Bu4NClO4 as the supporting electrolyte. The three-electrode system consisted of a glassy carbon electrode (3 mm diameter) as a working electrode, an Ag/AgCl (3 M KCl) reference electrode, and a platinum wire as auxiliary electrode. Thermogravimetric analysis data were measured from room temperature to 1000°C at a heating rate of 10°C/min. The data were obtained using a PerkinElmer Diamond TG/DTA instrument. Molar conductivity measurements were recorded on an Elico-CM-82 T conductivity bridge with a cell constant 0.51, and magnetic moment determination was carried out using a Faraday balance.
Synthesis
All the chemicals used were of reagent grade. 7-hydroxy-4-Methylcoumarin was synthesized according to the literature procedureCitation19.
Synthesis of 8-formyl-7-hydroxy-4-methylcoumarin
8-Formyl-7-hydroxy-4-methylcoumarin was prepared by the known methodCitation17. 7-Hydroxy-4-methylcoumarin (0.03 mol) and hexamine (0.07 mol) in glacial acetic acid (50 mL) were heated for 4–5 h, then 20% HCl (75 mL) was added and the mixture further heated for 20 min, then cooled and extracted with ether. The pale yellow-colored solid of 8-formyl-7-hydroxy-4-methylcoumarin that was obtained after extraction was recrystallized from hot ethanol: m.p. 176–177°C, yield 2.5 g.
Synthesis of Schiff bases H2LI and H2LII
The Schiff bases were synthesized by refluxing the reaction mixture of hot ethanol solution (30 mL) of o-phenylenediamine/ethylenediamine (0.01 mol) and hot ethanol solution (30 mL) of 8-formyl-7-hydroxy-4-methylcoumarin (0.02 mol) for 4–5 h with the addition of 2–3 drops of hydrochloric acid. The precipitate that formed during reflux was filtered, washed with cold EtOH, and recrystallized from EtOH.
Synthesis of Co(II), Ni(II), and Cu(II) complexes (1–6)
An alcoholic solution (45 mL) of Schiff base (1 mmol) was mixed with an alcoholic solution (10 mL) of 1 mmol of CoCl2.6H2O/NiCl2.6H2O/CuCl2.2H2O and refluxed on a water bath for 2 h. Then, to the reaction mixture, 2 mmol of sodium acetate was added and reflux was continued for 3 h. The separated complex was filtered, washed thoroughly with water, ethanol, and ether, and finally dried under vacuum over fused CaCl2.
Preparation of culture media
Nutrient broth (peptone, 10; yeast extract, 5; NaCl, 10; in g/L) was used for culturing of Escherichia coli and potato dextrose broth (potato, 250; dextrose, 20; in g/L) was used for the culture of Aspergillus niger. Media (50 mL) were prepared, and autoclaved for 15 min at 121°C under 15 lb pressure. The autoclaved media were inoculated with the seed culture. E. coli was incubated for 24 h and A. niger for 48 h at 37°C.
Isolation of DNA
The fresh bacterial culture (1.5 mL) was centrifuged to obtain a pellet which was then dissolved in 0.5 mL of lysis buffer (100 mM Tris, pH 8.0, 50 mM EDTA (ethylenediaminetetraacetic acid), 10% SDS (sodium dodecyl sulfate)). To this, 0.5 mL of saturated phenol was added and the mixture was incubated at 55°C for 10 min then centrifuged at 10,000 rpm for 10 min, and to the supernatant, an equal volume of chloroform–isoamyl alcohol (24:1) and one-twentieth volume of 3 M sodium acetate (pH 4.8) were added. The mixture was centrifuged at 10,000 rpm for 10 min, and to the supernatant, three volumes of chilled absolute alcohol were added. The precipitated DNA was separated by centrifugation, and the pellet was dried and dissolved in TAE buffer (10 mM Tris, pH 8.0, 1 mM EDTA) and stored in cold conditions.
DNA cleavage experiment
DNA cleavage experiments were done according to the literature procedureCitation20.
Agarose gel electrophoresis
Cleavage products were analyzed by the agarose gel electrophoresis methodCitation20. Test samples (1 mg/mL) were prepared in DMF. Samples (25 μg) were added to the isolated DNA of E. coli and A. niger. The samples were incubated for 2 h at 37°C, and then 20 μL of the DNA sample (mixed with bromophenol blue dye in a 1:1 ratio) was loaded carefully into the electrophoresis chamber wells along with standard DNA marker containing TAE buffer (4.84 g Tris base, pH 8.0, 0.5 M EDTA/L) and finally loaded on agarose gel, and a constant 50 V of electricity was passed for around 30 min. The gel was removed and stained with 10.0 μg/mL ethidium bromide for 10–15 min, and the bands observed under a Vilberlourmate gel documentation system and photographed to determine the extent of DNA cleavage. The results were then compared with standard DNA marker.
In vitro antibacterial and antifungal assay
The biological activities of synthesized Schiff bases and their Co(II), Ni(II), and Cu(II) complexes have been studied for their antibacterial and antifungal activities by agar and potato dextrose agar diffusion methods, respectively, in DMF solvent against Escherichia coli, Staphyllococcus aureus, Psuedomonas aeruginosa, and Salmonella typhi bacterial species and Aspergillus niger, A. flavus, and Cladosporium fungal speciesCitation21. A stock solution (1 mg/ mL) of test chemical was prepared by dissolving 10 mg of the test compound in 10 mL of DMF solvent. The stock solution was suitably diluted with sterilized distilled water to obtain concentrations of of 100, 50, and 25 μg/mL. A control for each dilution was prepared by diluting 10 mL of solvent instead of stock solution with sterilized distilled water.
The bacteria were subcultured in agar medium. Petri dishes were incubated for 24 h at 37ºC. The standard antibacterial drug, gentamicin, was also screened under similar conditions for comparison. The fungi were subcultured in potato dextrose agar medium. The standard antifungal drug, fluconazole, was used for comparison. Petri dishes were incubated for 48 h at 37ºC. Sterile disks were used in the agar media. Activity was determined by measuring the diameter of the zone showing complete inhibition (mm).
Compounds showing promising antibacterial/antifungal activity were selected for minimum inhibitory concentration (MIC) studiesCitation21.
Results and discussion
The synthesis of Schiff bases is schematically presented in Scheme 1. The Schiff bases H2LI and H2LII with CoCl2.6H2O/NiCl2.6H2O/CuCl2.2H2O in EtOH give octahedral complexes (1–6) where the coordination sphere of the central metal ion is occupied by two water molecules. All the Co(II), Ni(II), and Cu(II) complexes were colored, stable, and non-hygroscopic in nature. The complexes were insoluble in common organic solvents but soluble in DMF and DMSO. The elemental analyses showed that the Co(II), Ni(II), and Cu(II) complexes have 1:1 stoichiometry of the type ML.2H2O, where L stands for a doubly deprotonated ligand. The molar conductance values are too low to account for any dissociation of the complexes in DMF, indicating the non-electrolytic nature of the complexes in DMF ().
Table 1. Elemental analysis of Schiff bases and their Co(II), Ni(II), and Cu(II) complexes along with molar conductance and magnetic moment data.
Infrared spectra
The prominent infrared spectral data of Schiff bases and their Co(II), Ni(II), and Cu(II) complexes are presented in .
Table 2. The important infrared frequencies (in cm−1) of Schiff bases and their metal complexes.
The IR spectra of the Schiff bases exhibited a characteristic high-intensity band at 1613– 1632 cm−1, which is attributed to the ν(C=N) vibrationCitation22. The broad band around 3072– 3062 cm−1 and a strong band at 1306– 1305 cm−1 in the spectra of the Schiff bases are assigned to H-bonded –OH stretching and phenolic ν(C-O) vibrations, respectively. Another strong band at 1735– 1720 cm−1 is assigned to ν(C=O), the lactonyl carbon of the coumarin moietyCitation23.
In comparison with the spectra of the Schiff bases, all the complexes exhibited the band of ν(C=N) in the region 1598– 1622 cm−1; the shift of the band to lower wave numbersCitation24 indicates that the azomethine nitrogen is coordinated to the metal ion. The disappearance of the broad band due to H-bonded –OH stretching in the spectra of metal complexes gives evidence for the formation of M-O bonds via deprotonation. Further, the high-intensity band due to phenolic C-O at 1306– 1305 cm−1 in the Schiff bases appeared as a medium- to high-intensity band in the 1331– 1358 cm−1 region in the complexes. Rupini et al. and El-Sharief et al. have reported similar features of ν(C=N) and phenolic OH groups of the Schiff bases of formyl coumarinCitation24,Citation25. The presence of coordinated water molecules in the complexes are confirmed by a broad band around 3433– 3449 cm−1 and two weak bands in the regions 800–750 and 720– 700 cm−1 due to ν(OH) rocking and wagging modes of vibrations, respectivelyCitation24,Citation26. The new bands in the regions 381–375 and 524– 480 cm−1 in all complexes are assigned to stretching frequencies of (M-O) and (M-N) bonds, respectivelyCitation27,Citation28. The unaltered position due to ν(C=O) in all the complexes confirms its non-involvement in coordination.
Thus, the IR spectral data provide strong evidence for complexation of the potentially tetradentate Schiff bases with the ONNO sequence.
NMR spectral study of Schiff bases
The Schiff bases have been characterized by 1H and 13C NMR spectra and also 2D 1H-1H HOMOCOSY (correlation spectroscopy) to ensure ligand purity in solution and elucidate the differently positioned proton and carbon. The 1H NMR and 13C NMR spectral data are produced in .
Table 3. The important 1H NMR and 13C NMR data for Schiff bases.
In the 1H NMR spectrum of H2LI, signals at 14.7 and 2.4 ppm are ascribed to phenolic –OH and aromatic –CH3, respectively. A characteristic singlet proton signal at 9.42 ppm is assigned to –CH=N. In addition to these, the multiplet signals around 6.1–7.6 ppm are due to aromatic protons. In the case of H2LII, the singlets at 14.3, 9.1, and 2.5 ppm are attributed to phenolic –OH, –CH=N, and aromatic –CH3, respectively. In addition to these, the multiplet signals around 6.1–7.7 ppm and triplet around 2.1 ppm are due to aromatic and CH2-CH2 protons, respectively. In both Schiff bases, phenolic –OH proton signals disappeared after the addition of D2OCitation29.
In the 13C NMR spectra of H2LI and H2LII, the signals appearing in the region 111.3–158.6 ppm are assigned to aromatic carbons. The signals at 172.8–165.6, 144.3–142.3, and 38.4–36.8 ppm are due to C=O, C=N, and CH3, respectively. In the case of H2LII an additional signal at 21.4 ppm is ascribed to the carbon of CH2-CH2.
In the two-dimensional 1H-1H HOMOCOSY NMR spectra, the signals observed are due to the interaction of proton and proton, in which the proton values are recorded along both the y-axis and the x-axis. A representative two-dimensional NMR spectrum of H2LI is discussed here. The spectrum clearly included all the protons of the aromatic region observed as a cross peak in the region 6.1–7.7 ppm. The peaks due to CH=N and CH3 were well resolved and a signal was observed at corresponding coinciding points of the y- and the x-axis.
Thus, the NMR results support the IR inferences of the Schiff bases.
Electronic spectra
The electronic spectra of the Co(II) complexes exhibited absorption bands in the region 8000–10, 000 cm−1 and 18,000–20, 000 cm−1, corresponding to the transitions 4T1g (F) → 4T2g (F) (ν1); 4T1g (F) → 4T1g (P) (ν3), respectively. In the present investigation, brownish Co(II) complexes showed absorption bands around 9654–9648 and 18,865–18, 876 cm−1, corresponding to ν1 and ν3 transitions, respectively. These bands are characteristic of high-spin octahedral Co(II) complexesCitation26. However, the ν2 band was not observed because of its proximity to the strong ν3 transition.
The greenish Ni(C28H18N2O6).2H2O complex exhibited three bands at 10,582, 16,393 and 26, 178 cm−1, attributed to the 3A2g →3T2g (ν1); 3A2g → 3T1g (F) (ν2); and 3A2g → 3T1g (P) (ν3) transitions, respectively, which indicate octahedral geometry around the Ni(II) ion. The ligand field parameters are presented in “Supplementary material” Table S1. The value of the ν2/ν1 ratio was found to be around 1.549, and the μeff value is around 3.167, which is within the range of 2.8–3.5 BM, suggesting the octahedral environment. The values of the nephelauxetic parameter, β, indicate the low covalent character of the metal–ligand σ bondsCitation30. Hence, the ligand field parameters correlate with the electronic spectral and magnetic properties.
The electronic spectra of the Cu(II) complex displayed two prominent bands. A low-intensity broad band at 16, 658 cm−1 is assignable to the 2Eg → 2T2g transition. Another high-intensity band at 25, 523 cm−1 is due to symmetry-forbidden ligand → metal charge transfer. On the basis of the electronic spectra, a distorted octahedral geometry around the Cu(II) ion is suggestedCitation31.
Magnetic studies
The magnetic moments obtained at room temperature are listed in . The magnetic measurement for the Co(II) complexes showed magnetic moment values of 4.9 and 4.75, which are well within the range of 4.3–5.2 BM, and the Ni(II) complexes showed magnetic moment values of 3.3 and 3.1, within the range of 2.8–3.5 BM, suggestingCitation32,Citation33 consistency with their octahedral environment. The Cu(II) complexes exhibited magnetic moment values of 1.74 and 1.75 BM, slightly higher than the spin-only value of 1.73 BM expected for one unpaired electron, which offers the possibility of a distorted octahedral geometryCitation34.
ESR spectroscopy
The ESR spectrum of a representative Cu(II) complex, Cu(C28H18N2O6).2H2O, showed a g value of 2.00277. The gι and g⊥ values were found to be 2.0273 and 2.1240, respectively. The gav was calculated to be 2.0919. The Cu(II) complex spectrum was reversed axial, with g⊥ > gι. The complex would thus have an essentially dzCitation2 ground state, which suggests either a compressed tetragonal, cis-octahedral, or distorted octahedral structureCitation35,Citation36. Thus, the ESR spectral results provide further evidence to support the magnetic and electronic spectral results.
FAB-mass spectral studies of Schiff bases and their complexes
The FAB-mass spectrum of H2LI is depicted in “Supplementary material” Figure S1. The spectrum showed a molecular ion peak at m/z 480, which is equivalent to its molecular weight. In addition, the fragment peaks at m/z 465 and 292 are due to the cleavage of CH3 and C10H8O3, respectively. In the case of H2LII the molecular ion peak at m/z 432 is ascribed to C24H20N2O6. The peaks at 417 and 241 are assigned to the cleavage of CH3 and C10H8O3, respectively.
The FAB-mass spectra of Co(II), Ni(II), and Cu(II) complexes with H2LI showed a molecular ion peak M+ at m/z 573, 572, and 577, respectively, equivalent to their molecular weights. All the fragments of the species [ML.2H2O]+ undergo demetallation to form the species [L + 2H]+ giving a fragment ion at m/z 480. The fragmentation peaks observed in the case of Cu(C28H18N2O6).2H2O at 541, 354, 250, and 63 correspond to 2H2O, C11H7O3, C6H4N2, and C11H7O3, respectively. Also, the species [ML.2H2O]+ undergoes demetallation to form the species [L + 2H]+ giving a fragment ion at m/z 480. Similar features are evident for other complexes. All these fragmentation patterns were well observed in the FAB-mass spectra.
Thermal studies
The thermal behavior of Co(II), Ni(II), and Cu(II) complexes has been studied as a function of temperature. The thermal behavior of all the complexes is almost the same. Hence, the representative Co(C28H18N2O6).2H2O, Ni(C28H18N2O6).2H2O, and Cu(C28H18N2O6).2H2O complexes are discussed.
The Cu(C28H18N2O6).2H2O complex is represented in “Supplementary material” Figure S2. The thermal decomposition of the Cu(II) complex took place in three steps as indicated by differential thermal gravimetry (DTG) curves around 205–255, 335–370, and 505–535ºC, corresponding to the mass loss of coordinated water molecules, aminophenol, and formyl coumarin moieties, respectively. In the case of Co(II) and Ni(II) complexes, decomposition took place in three steps corresponding to the loss of coordinated water molecules, aminophenol, and formyl coumarin moieties around 210–240, 270–300, and 495–530ºC, respectively. Both water molecules of all the complexes depart in a considerably narrow (~50 K) one-step process. This may be due to an easier diffusion of water molecules through the crystal lattice of the complexes. Finally, the metal complexes decomposed gradually with the formation of metal oxides above 535ºC. The nature of the proposed chemical change with the temperature range and the percentage of metal oxide obtained are given in “Supplementary material” Table S2.
Electrochemistry
The cyclic voltammogram of the Cu(C28H18N2O6).2H2O complex is depicted in “Supplementary material” Figure S3. A cyclic voltammogram of the Cu(II) complex displayed two reduction peaks, the first at Epc = –1.5894 V, with corresponding oxidation peak at Epa = 0.4938 V, and a second at Epc = –2.3235 V,with a corresponding oxidation peak at Epa = −0.2410 V. The peak separation of the first couple (νEp) is 1.0956 V at 200 mV/s and that of the second couple is 2.0825 V. The most significant feature of the Cu(II) complex is the Cu(II)/Cu(I) couple, and the redox process proceeds through the formation of the radical ion. The difference between forward and backward peak potentials can provide a rough evaluation of the degree of reversibility of the one-electron transfer reaction. The analysis of cyclic voltammetric responses with varying scan rates 50–200 mV/s gave evidence for the quasi-reversible one-electron oxidation state (“Supplementary material” Figure S4). The ratio of cathodic to anodic peak height was less than one. However, the peak current increased with an increase of the square root of the scan rate. This establishes the electrode process as diffusion controlledCitation37. The separation in peak potentials increased at higher scan rates. These characteristic features are consistent with the quasi-reversibility of the Cu(II)/Cu(I) couple.
Fluorescence spectroscopy
The emission spectra of the Schiff bases and the complexes were studied in DMF and DMSO.
H2LI is characterized by an emission band around 486 nm in DMF and 490 nm in DMSO (“Supplementary material” Figure S5), due to the formation of the phenoxide anion. Upon the addition of 2–3 drops of aqueous alkali (2% NaOH) to the Schiff base solution, we observed λmax values at 458 nm in DMF and 461 nm in DMSO. In the case of H2LII, an emission band around 472 nm in DMF and 477 nm in DMSO was observed. On the addition of 2–3 drops of aqueous alkali (2% NaOH) to the Schiff base solutions, we observed λmax values at 446 nm in DMF and 452 nm in DMSO, respectively. The shift in λmax values after the addition of 2–3 drops of aqueous alkali (2% NaOH) in both Schiff bases clearly indicates that the proton transferred (H-bonded ion pair) species exist in equilibriumCitation38,Citation39.
The emission spectra of the Co(II), Ni(II), and Cu(II) complexes with H2LI were characterized by an emission band around 476/483 nm, 476/464 nm, and 501/501 nm in DMF/DMSO (“Supplementary material” Figure S6). In the case of Co(II), Ni(II), and Cu(II) complexes of H2LII emission bands were observed at 454 nm, 459 nm, and 462 nm, respectively, in DMF and at 455 nm, 461 nm, and 468 nm, respectively, in DMSO. The shift of the λmax value of the complexes compared with the Schiff bases is possibly due to the deprotonation of the hydroxyl group.
Biological results
In vitro antibacterial and antifungal activity
The microbial results are systematized in . The antibacterial and antifungal studies suggested that all the Schiff bases were biologically active, and some of their metal complexes showed significantly enhanced antibacterial and antifungal activity. It is, however, knownCitation40,Citation41 that chelation tends to make Schiff bases act as more powerful and potent bactereostatic agents, thus inhibiting the growth of bacteria and fungi more than the parent Schiff bases. It is suspected that factors such as solubility, conductivity, dipole moment, and cell permeability mechanism (influenced by the presence of metal ions) may be possible reasons for the increase in activity.
Table 4. Biological results for Schiff bases and their metal complexes.
In the antibacterial studies it was observed that both Schiff bases were potentially active against E. coli, S. typhi, and S. aureus. All the Co(II), Ni(II), and Cu(II) metal complexes showed much enhanced activity against S. typhi, and Cu(II) complexes exhibited promising results against E. coli. A most interesting finding was that both the Schiff bases and their metal complexes showed higher activity compared to the standard drug against S. aureus. Both the Schiff bases and their Co(II), Ni(II), and Cu(II) complexes were found to possess higher antifungal activity than antibacterial activity. The antifungal activity of synthesized Schiff bases and some of their complexes was close to that of the standard drug fluconazole (). It has been reported in the past that metal complexes with Schiff bases of formyl coumarin derivatives possess high antimicrobial activityCitation17,Citation18,Citation23,Citation42–44.
The minimum inhibitory concentration (MIC) of some selected compounds, which showed significant activity against selected bacterial and fungi species, was also determined, and results are presented in . The MIC of these compounds indicated that they were most active in inhibiting the growth of the tested organisms at a 10 μg/mL concentration.
Table 5. Minimum inhibitory concentration (μg/mL) of selected compounds against selected bacteria.
Electrophoretic analysis
Representative Co(C24H18N2O6).2H2O and Cu(C24H18N2O6).2H2O complexes were studied for their DNA cleavage activity by the agarose gel electrophoresis method, and results are presented in .
Figure 1. DNA cleavage study of Co(II) (2) and Cu(II) (6) complexes on genomic DNA. M, standard molecular weight marker; C- E.coli, control DNA of E. coli; lane 1, E. coli DNA treated with Co(C24H18N2O6).2H2O; lane 2, E. coli DNA treated with Cu(C24H18N2O6).2H2O; lane 3, A. niger DNA treated with Co(C24H18N2O6).2H2O; lane 4, A. niger DNA treated with Cu(C24H18N2O6).2H2O; C- niger: control DNA of A. niger.
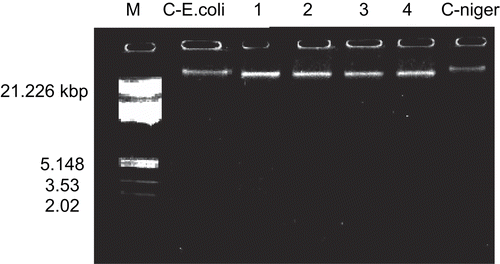
The gel after electrophoresis clearly revealed that both complexes acted on DNA, as there was a molecular weight difference between the control and the treated DNA samples. A difference was observed in the bands of the complexes (lanes 1–4) compared to the control DNA of E.coli and A. niger. This indicated that the control DNA alone did not show any apparent cleavage, unlike the complexes. However, the nature of the reactive intermediates involved in DNA cleavage by the complexes is not clear. The results indicate the important role of metal in these isolated DNA cleavage reactions. As the compounds were observed to cleave DNA, it can be concluded that the compounds inhibited growth of the pathogenic organism by cleaving the genome.
Conclusions
The newly synthesized Schiff bases act as tetradentate ligands. The metal ion is coordinated through the azomethine nitrogen and phenolic oxygen atoms. The bonding of ligand to metal ion is confirmed by the analytical, spectral, magnetic, thermal, and fluorescence studies.
Biological study reveals that the Schiff bases and some metal complexes are highly active against E. coli and S. typhi. In antifungal studies, both Schiff bases and some of their complexes are found to be highly active. Co(II) (2) and Cu(II) (6) complexes show non-specific cleavage of DNA. The electrochemical properties of the metal complexes, investigated in DMF, show that the most significant feature of the Cu(II) complex is the Cu(II)/Cu(I) couple.
All these observations put together lead us to propose the following structures ( and ) in which the complexes have stoichiometry of the type ML.2H2O (M = Co(II), Ni(II), and Cu(II)).
Ligand field parameters of Ni(II) complexes with Schiff bases.
Download GIF Image (96.4 KB)Ligand field parameters of Ni(II) complexes with Schiff bases.
Download GIF Image (172.8 KB)Ligand field parameters of Ni(II) complexes with Schiff bases.
Download GIF Image (83 KB)Ligand field parameters of Ni(II) complexes with Schiff bases.
Download GIF Image (114.1 KB)Ligand field parameters of Ni(II) complexes with Schiff bases.
Download GIF Image (53.6 KB)Ligand field parameters of Ni(II) complexes with Schiff bases.
Download GIF Image (71 KB)Acknowledgments
Declaration of interest: The authors report no conflicts of interest.
References
- Egan D, O’Kennedy R, Moran E, Cox D, Prosser E, Thornes RD. Drug Metab Rev 1990;22:503–29.
- Hoult Paya M. Gen Pharmacol 1996;27:713–22.
- Laurin P, Ferroud D, Klich M, Dupuis-Hamelin C, Mauvais P, Lassaigne P, et al. Bioorg Med Chem Lett 1999;9:2079–84.
- Connor DT. US Patent. 1981;126:287.
- Emmanuel-Giota AA, Fylaktakidou KC, Hadjipavlou-Litina DJ, Litinas KE, Nicolaides DN. J.Heterocyclic Chem 2001;38:717–22.
- Nofal ZM, El-Zahar M, Abd El-Karim S. Molecules 2000;5:99–113.
- Manolov I, Danchev ND. Eur J Med Chem Chim Ther 1995;30:531–6.
- Kirkiacharian S, Thuy DT, Sicsic S, Bakhchinian R, Kurkjian R, Tonnaire T. Farmaco 2002;57:703–8.
- Yu D, Suzuki M, Xie L, Morris-Natschke SL, Lee KH. Med Res Rev 2003;23:322–45.
- Marshall EM, Ryles M, Butler K, Weiss L. J Cancer Res Clin Oncol 1994;120:535–8.
- Yanping Lia, Yanbo Wua, Jing Zhaoa, Yang Pin. J Inorg Biochem 2007;101:283–90.
- Xiang-Li Wang, Hui Chao, Hong Li, Xian-Lan Hong, Liang-Nian Ji, Xiao-Yuan Li. J Inorg Biochem 2004;98:423–9.
- Cowan JA. Curr Opin Chem Biol 2001;5:634–42.
- Liu J, Zhang T, Lu T, Qu L, Zhou H, Zhang Q, et al. J Inorg Biochem 2002;91:269–76.
- Vaidyanathen VG, Nair BU. J Inorg Biochem 2003;93:271–6.
- Reddy PR, Rao KS, Satyanarayana B, Tetrahedron Lett. 2006;47:7311–7315.
- Bagihalli GB, Avaji PG, Patil SA, Badami PS. J. Coord. chem. 2008;61:2793–2806.
- Bagihalli GB, Avaji PG, Patil SA, Badami PS. Eur J Med Chem 2008;43:2639–49.
- Ahluwalia VK, Bhagat P, Aggarwal R, Chandra R. Intermediates for Organic Synthesis. Delhi: I.K. International Pvt. Ltd., 2005.
- Brown TA, Molecular biology- A practical approach, Oxford University Press, USA, Volume 1, 51–52, (1990).
- Sadana AK, Mirza Y, Aneja KR, Prakash O. Eur J Med Chem 2003;38:533–6.
- Patil SA, Kulkarni VH. Polyhedron. 1984;3(1):21–24.
- Rehman SU, Chohan ZH, Gulnaz1 F, Supuran CT. J Enzyme Inhib Med Chem 2005;20:333–40.
- Rupini B, Mamatha K, Mogili R, Ravinder M, Srihari S. J. Indian Chem. Soc. 2007;84:629–631.
- El-Sharief AMS, Gharib EAA, Ammar YA. J. Indian Chem. Soc. 1983;LX:1017–1019.
- Singh K, Barwa MS, Tyagi P. Eur J Med Chem 2006;41:147–53.
- Dholakiya PP, Patel MN. Synth. React. Inorg., Metal-Org., and Nano-Met. Chem. 2002;32(4):819–829.
- Nakamoto K. Infrared Spectra of Inorganic and Coordination Compounds. New York: Wiley-Interscience, 1970.
- Emara Adel AA, Adly Omima MI. Trans Met Chem 2007;32:889–901.
- Ayman K, El-Sawaf, Douglas X, West A, Fathy El-Saied, El-Bahnasawy MR Trans Met Chem 1998;23:649–55.
- Huiyan Liu, Haiying Wang, Feng Gao, Dezhong Niu, Zaisheng Lu. J Coord Chem 2007;60:2671–8.
- Rao TR, Prasad Archana. Synth React Inorg Met Org Nano Met Chem 2005;35:299–304.
- Hankare PP, Naravane SR, Bhuse VM, Delekar SD, Jagtap AH. Indian J. Chem. 2004;43A:1464–1467.
- Singh DP, Ramesh Kumar, Malik V, Tyagi P. Trans Met Chem 2007;32:1051–5.
- McGregor KT, Halfield WE. J Chem Soc Dalton Trans 1974:2448–51.
- .
- Bard A-J, Izatt L-R, eds. Electrochemical Methods: Fundamentals and Applications, 2nd ed. New York: Wiley, 2001.
- Mukherjee S. Indian J. Chem. 1987;26:1002–1005.
- Avaji PG, Patil SA, Badami PS. Trans Met Chem 2008;33:275–83.
- Chohan ZH, Supuran CT, Scozzafava A. J Enzyme Inhib Med Chem 2004;19:79–84.
- Chohan ZH, Praveen M. Appl Organomet Chem 2001;15:617–25.
- Kulkarni A, Avaji PG, Bagihalli GB, Patil SA, Badami PS. J Coord Chem 2009;62:481–92.
- Bagihalli GB, Patil SA, Badami PS. J Enzyme Inhib Med Chem 2009;24:381–94.
- Bagihalli GB, Patil SA, Badami PS. J. Enzym. Med. Chem. 2009;24(03): 730–741..