Abstract
Vibrio cholerae, a Gram-negative bacterium, is the causative agent of cholera and colonizes the upper small intestine where sodium bicarbonate is present at a high concentration. Sodium bicarbonate is a potential inducer of virulence gene expression. Bacteria can increase cytosolic bicarbonate levels through the existence of transporter family proteins or through the action of metalloenzymes, called carbonic anhydrases (CAs, EC 4.2.1.1). Vibrio cholerae, lacking of transporter proteins in its genome, utilizes the CA system to accumulate bicarbonate into the cell suggesting a pivotal role of this metalloenzymes in the microbial virulence. Here, we report for the first time the characterization of the α-CA of V. cholerae (VchCA), which has been identified by translated genome inspection. The α-CA encoding gene was cloned and expressed in Escherichia coli and the recombinant protein purified to homogeneity. This investigation aimed to study the biochemical properties of VchCA and to provide preliminary insights in the field of this pathogen virulence. VchCA has a low esterase activity with 4-nitrophenyl acetate as substrate, and a high activity for the hydration of CO2 to bicarbonate.
Introduction
Cholerae is a human disease that is characterized by massive loss of water and electrolytes, which leads to severe dehydration and hypovolemic shock if the condition is not treated. The causative agent of cholera is Vibrio cholerae, a Gram-negative bacteriumCitation1. Cloning of the genomes of many bacterial pathogens offers the possibility to explore alternative pathways for inhibiting virulence factors or proteins essential for their life cycleCitation2–5.
Bacteria can increase cytosolic bicarbonate levels using at least two ways: (a) through the existence of transporters that can directly bind to and import bicarbonate, one example being the bicarbonate transport system of Synechococcus elongatus PCC 6301Citation6 and (b) through the action of enzymes, such as carbonic anhydrases (CAs, EC 4.2.1.1), that convert into bicarbonate the metabolic CO2 and/or atmospheric CO2 entered into the cell by simple diffusionCitation7.
Inspection of the V. cholerae genome using the FASTA programCitation8 revealed no sequences homologous to the transporter system. Our hypothesis was at the point that V. cholerae utilizes the CA system to accumulate bicarbonate into the cell. Actually, CAs catalyze a simple but physiologically relevant process in all life kingdoms, carbon dioxide hydration to bicarbonate and protonsCitation5,Citation9–14. CAs have been shown to be involved in many cellular processes (in all life kingdoms), such as photosynthesis, respiration, CO2 transport and cyanate metabolism in Escherichia coliCitation2,Citation4,Citation7. Five genetically distinct CA families are known to date, the α-, β-, γ-, δ- and ζ-CAs, and all of them are metalloenzymes, using Zn(II), Cd(II), or Fe(II) at their active sitesCitation2,Citation4,Citation5. Bacteria, the most successful organisms on earth, encode CAs belonging to the α-, β- and/or γ-CA familiesCitation2–5,Citation10,Citation11,Citation15. Many such enzymes were investigated in detail lately in pathogenic (as well as non-pathogenic) bacteria such as Brucella suisCitation16,Citation17, Mycobacterium tuberculosisCitation18,Citation19, Streptococcus pneumoniaeCitation20, Helicobacter pyloriCitation21, Salmonella entericaCitation22, Sulfurihydrogenibium spp.Citation14,Citation23, etc. in the search for antibiotics with a novel mechanism of action, since it has been demonstrated that in many of them, CAs are essential for the life cycle of the organismCitation2. The genome of V. cholerae encodes for putative CAs belonging to each bacterial class, i.e. an α-CA originated from the cah gene (VC0395_0957), a β-CA derived from the gene VC0395_A 0118 and a γ-CA encoded by the gene VC0395_A2463. Ethoxzolamide 1, a potent CA inhibitor (CAI), was shown to inhibit bicarbonate-mediated virulence induction, suggesting that conversion of CO2 into bicarbonate by one or more V. cholerae CAs plays a role in virulence inductionCitation24.
In this article, we report for the first time the characterization of the α-CA of V. cholerae (denominated from now on as VchCA), which has been identified by translated genome inspection. This investigation aimed to study the biochemical properties of VchCA and to provide preliminary insights in the field of this pathogen virulence. The α-CA encoding gene was cloned and expressed in E. coli and the recombinant protein purified to homogeneity.
Materials and methods
Gene identification
The identification of V. cholerae CA (VchCA) was performed at the link http://www.ncbi.nlm.nih.gov/protein using in the keyword fields the word: “Vibrio cholerae and carbonic anhydrase”. The VchCA was identified running the “BLAST” program.
Construct preparation and protein expression
The GeneArt Company (Regensburg, Germany), specialized in gene synthesis, designed the synthetic V. cholerae gene encoding for the α-CA, lacking of the signal peptide (i.e. the first 20 amino acid residues of the peptide sequence) and containing a NdeI and XhoI site at the 5′ and 3′ end of the VchCA gene, respectively. The resulting plasmid was amplified into E. coli DH5 α-cells. The V. cholerae DNA fragments were separated on 1% agarose gel. The recovered V. cholerae gene and the linearized expression vector (pET15-b) were ligated by T4 DNA ligase to form the expression vector pET15-b/Vch. In order to confirm the integrity of the V. cholerae gene and the fact that no errors occurred at the ligation sites, the vector containing the fragment was sequenced. Competent E. coli BL21 (DE3) cells were transformed with pET15-b/Vch, grown at 37 °C, induced with 1 mM IPTG and grown for 5 h.
Purification
After additional growth for 5 h, cells were harvested and disrupted by sonication at 4 °C. Following centrifugation, the cell extract was placed in conical flasks, and an amount of ammonium sulfate gradually added to obtain saturations of 45%. After resting for 14 h at 4 °C, the sample was centrifuged at 1200 × g at 4 °C for 30 min. The precipitate was resuspended in 20 mM buffer phosphate, pH 8.0 and loaded onto a His-select HF nickel affinity gel. The protein was eluted with 250 mM imidazole. At this stage of purification the enzyme was at least 95% pure and the obtained recovery was of 30 mg of the recombinant bacterial CA.
Sodium dodecyl sulfate-polyacrylamide gel electrophoresis
Sodium dodecyl sulfate (SDS)-polyacrylamide gel electrophoresis (PAGE) was performed as described previouslyCitation25 using 12% gels.
Assay for CA with CO2 as substrate
CA activity assay was a modification of the procedure described by Chirica et al.Citation26. The assay was based on monitoring the pH variation due to the catalyzed conversion of CO2 to bicarbonate. Bromothymol blue was used as the indicator of pH variation. The assay was performed at 0 °C adding 1.0 mL ice-cold CO2-saturated water to 1.0 mL mixtures of 25 mM Tris-SO4 buffer containing different amounts of the enzyme. The CO2-satured solution was prepared by bubbling CO2 into 100 mL of distilled water for approximately 3 h. The CO2 solution was chilled in an ice water bath. To test the activity of the enzyme, 1 mL of 25 mM Tris, pH 8.3, containing bromothymol blue as a dye (to give a distinct and visible blue color) was added to two test tubes chilled in an ice bath. A total of 10–50 µL of the enzyme solution (e.g. cell extract or purified enzyme) were added to one tube, and an equivalent amount of buffer was added to the second tube as control. One milliliter of CO2 solution was added very quickly and simultaneously a stopwatch was started. The time required for the solution to change from blue to yellow was recorded (transition point of bromothymol blue is pH 6–7.6). The production of hydrogen ions during the CO2 hydration reaction lowers the pH of the solution until the color transition point of the dye is reached. The time required for the color change is inversely related to the quantity of CA present in the sample. Detecting the color change is somewhat subjective but the error for triple measurements was in the range 0–1 s difference for the catalyzed reaction. Wilbur-Anderson units (WAUs) were calculated according to the following definition: one WAU of activity is defined as (T0 − T)/T, where T0 (uncatalyzed reaction) and T (catalyzed reaction) are recorded as the time (in seconds) required for the pH to drop from 8.3 to the transition point of the dye in a control buffer and in the presence of enzyme, respectively.
Esterase activity
Activity for p-NpA hydrolysis was determined at 0 °C using a modification of the method proposed by Armstrong et al.Citation27. The reaction mixture contained 0.3 mL of freshly prepared 3 mM p-NpA and 0.7 mL of 15 mM Tris sulfate buffer, pH 7.6. A total of 10 µL of enzyme solution were added, and the catalyzed reaction was monitored by reading the increase in A348 nm for 5 min. The catalyzed reactions were corrected for the non-enzymatic reaction. One enzyme unit was defined as the amount capable of producing an OD348 nm = 0.03 in 5 min.
Effect of temperature on enzyme activity
The temperature dependence activities of VchCA and bCA II were measured using the p-NpA as substrate. Protein concentration used in the assay was 300 ng. The activity was measured in the temperature range from 25 °C to 100 °C. All the reactions were performed in triplicate.
Effect of temperature on the VchCA stability
To compare the stability of VchCA and bCA II at different temperatures, enzyme solutions at the concentration of 3 µg/mL in 10 mM Tris/HCl, pH 8.3 were incubated at 40, 50, 60, 70, 80, 90 and 100 °C for different times (30, 60, 120 and 180 min). Enzyme aliquots (30 ng) were withdrawn at appropriate times and the residual activity was measured at 0 °C using CO2 as substrate.
Results
Primary structure analysis
We aligned the amino acid sequence of VchCA and bCA II, to try to identify unique features of this bacterial enzyme (). It may be observed that similar to the other investigated α-CA, VchCA has the conserved three His ligands, which coordinate the Zn(II) ion crucial for catalysis (His94, 96 and 119, hCA I numbering system). The proton shuttle residue (His64) is also conserved in all these enzymes. This residue assists the rate-determining step of the catalytic cycle transferring a proton from the water coordinated to the Zn(II) ion to the environment, with formation of zinc hydroxide representing the nucleophilic species of the enzyme. VchCA also has the gate-keeping residues (Glu106 and Thr199), which orientate the substrate for catalysis, and are also involved in the binding of inhibitors. The only unique macro feature in the primary structure of the bacterial α-CA with respect to the mammalian enzyme was the absence of four amino acid loops indicated in ). This feature is typical of all α-CA of bacterial originCitation12–14. Deletion of the loops makes the protein smaller leading to a broader protein stability curveCitation28.
Figure 1. Alignment of the VchCA and bCA II amino acid sequences. The proton shuttle residue (His64), the zinc ligands (His94, 96 and 119) and the gate keeper residues (Glu106 and Thr199) are conserved in the bacterial and mammalian sequences. The hCA I numbering system was used. The bold letters (a, b, c and d) indicate the four loops absent in the bacterial enzyme. The asterisk (*) indicates identity at all aligned positions; the symbol (:) relates to conserved substitutions, while (.) means that semi-conserved substitutions are observed. Multialignment was performed with the program Clustal W, version 2.1. Legend: bCA II, Bos taurus, isoform II (accession number: NP_848667); VchCA, V. cholerae, α-CA (accession number: AFC59768.1).
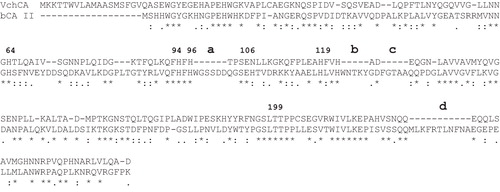
Enzyme purification
The recombinant VchCA was isolated and purified to homogeneity at room temperature from E. coli (DE3) cell extract (). Most of the CA activity was recovered in the soluble fraction of cell extract obtained after sonication and centrifugation. presents that the heterologously expressed VchCA enzyme was purified 1.4-fold with the ammonium sulfate precipitation step. Using the affinity column (His-select HF Nickel affinity gel), VchCA was purified 11-fold to apparent homogeneity, as indicated by a single protein band after SDS-PAGE (, Lane 4).
Figure 2. SDS-PAGE of the recombinant VchCA purified from E. coli. Lane Std, molecular markers, M.W. starting from the top: 250, 150, 100, 75, 50, 37, 25 and 20 k Da; Lane 1, cell extract protein from E. coli before induction with IPTG; Lane 2, cell extract protein after induction with IPTG; Lane 3, supernatant after 45% ammonium sulfate precipitation; and Lane 4, purified VchCA from His-tag affinity column.
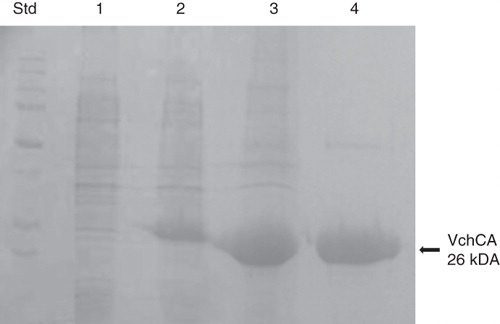
Table 1. Purification of recombinant VchCA produced in E. coli.
Biochemical characterization
Determination of molecular weight by SDS-PAGE
The molecular weight estimated by SDS-PAGE was 26.0 kDa. A subunit molecular mass of 26.4 kDa was calculated on the basis of the amino acid sequence translated from the gene.
CO2-hydrase and esterase activities
Using CO2 as substrate, the specific activities for VchCA and bCA II were determined. As indicated in , the specific activity of VchCA was 5.4 times greater than that of the mammalian enzyme. CAs (α-type) from mammalian sources catalyze the reversible hydrolysis of esters. With p-NpA as substrate, the presence of esterase activity was investigated in the bacterial enzyme. VchCA showed a specific activity of 19 U/mg of protein that is 60 times smaller than that of the commercially available bovine bCA II ().
Table 2. Comparison of the hydratase and esterase activity for VchCA and bCA II.
Effect of temperature on the enzyme activity
The temperature dependent activity of VchCA was assayed using the p-NpA as substrate, and measurements were performed in parallel with bCA II using 300 ng of each enzyme (). The optimum temperature ranges for VchCA and bCA II were determined at 25, 40, 50, 60, 70, 80, 90 and 100 °C. The reaction was monitored following the absorbance at 348 nm for 5 min. The optimum temperature for bovine enzyme activity was found to be 60 °C, while the VchCA showed an optimum at 70 °C ().
Temperature effect on the stabilities of VchCA and bCA II
Stabilities of VchCA and bCA II were compared at the temperatures indicated on the X-axis (). After 30, 120 and 180 min of incubation, the activities of VchCA and bCA II were determined using CO2 as substrate. The bovine enzyme was inactivated at temperature higher than 60 °C () for all incubation times. The bacterial enzyme retained its activity up to 70 °C (). Our hypothesis is that the bacterial enzyme was more stable than the bovine enzyme. This major stability might be attributed to the absence of for loops in the amino acid sequence of the bacterial α-CA ().
Discussion and conclusion
The potential microbial virulence depends on the ability to enter a host, to evade host defenses, to grow in a host environment, to counteract host immune responses, to assimilate iron and nutrients from the environment and to sense environmental change. All these abilities implicate the action of numerous enzymes. Enzymes considered as virulence factors are generally active against host components and contribute to virulence by damaging host tissues. Tissue damage makes the host permissive for microbial infection. Enzyme virulence factors that damage tissue include proteases, neurominidases and phospholipases. Other enzymes, such as urease, contribute to virulence by facilitating survival inside phagocytic cellsCitation29. CAs are enzymes present in several pathogenic species, suggesting a pivotal role in the microbial virulenceCitation7,Citation30. Investigations of CA in bacteria domains will undoubtedly reveal novel aspects of microbial virulence.
It is reported in the literature that the expression of the β-CA of Salmonella typhimurium, a Gram-negative facultative intracellular pathogen, is induced 24-fold upon infection in host macrophages, and it is necessary for bacterial survival within the hostCitation31. Vibrio cholerae is a Gram-negative bacterium that is the causative agent of cholera. A potential inducer of virulence gene expression is sodium bicarbonate, which is present at a high concentration in the upper small intestine, colonized by V. choleraeCitation24. The bicarbonate ion has been shown to promote virulence gene expression in other bacteria, such as, Streptococcus pyogenesCitation32, enterohemorrhagic E. coliCitation33, and the murine pathogen Citrobacter rodentiumCitation34,Citation35. Addition of a CAI caused a significant reduction in virulence gene expressionCitation24 suggesting that the potential CAs of V. cholerae (α, β and γ-CAs) could be implicated in the microbial virulence.
With the help of the recombinant DNA technology, the VchCA identified in the genome of this mesophilic bacterium was heterologously expressed in E. coli. The enzyme shared significant similarity with bCA II belonging to the α-CA class. A comparison of the biochemical properties of the bacterial and the mammalian enzyme showed: (a) the CO2-hydrase activity of VchCA was 5.4 times higher than that of bCA II; (b) VchCA displayed a rather low specific activity toward p-NpA, with an esterase activity 60 times lower than that of the mammalian enzyme; (c) VchCA activity curve against temperature was shifted toward higher temperatures (i.e. 70 °C) with respect to the bCA II and (d) the influence of temperature on the protein stability is more pronounced for the mammalian enzyme than for the bacterial enzyme. This major stability might be attributed to the absence of loops in the amino acid sequence of the bacterial α-CA.
Here, we described a preliminary work concerning some biochemical properties of VchCA. Our group is preparing a detailed study of the inhibitory profile of the most important classes of CAIs (sulfonamides, sulfamates and sulfamide)Citation17,Citation22,Citation36,Citation37 against the VchCA in the search of drugs with a novel mechanism of action, since it has been demonstrated that in many such bacteria, CAs are essential for their life cycle.
In conclusion, prokaryotic CAs can have medical importance because many of these enzymes are druggable targets, and therefore provide potential for the development of pharmacological agents.
Declaration of interest
This study was financed in part by an FP7 EU project (Metoxia, to Claudiu T. Supuran and Andrea Scozzafava). The authors report no conflicts of interest.
References
- Cash RA, Music SI, Libonati JP, et al. Response of man to infection with Vibrio cholerae. I. Clinical, serologic, and bacteriologic responses to a known inoculum. J Infect Dis 1974;129:45–52
- Supuran CT. Bacterial carbonic anhydrases as drug targets: toward novel antibiotics? Front Pharmacol 2011;2:34
- Supuran CT. Carbonic anhydrase inhibitors and activators for novel therapeutic applications. Future Med Chem 2011;3:1165–80
- Supuran CT. Carbonic anhydrase inhibitors. Bioorg Med Chem Lett 2010;20:3467–74
- Supuran CT. Carbonic anhydrases: novel therapeutic applications for inhibitors and activators. Nat Rev Drug Discov 2008;7:168–81
- Maeda S, Price GD, Badger MR, et al. Bicarbonate binding activity of the CmpA protein of the cyanobacterium Synechococcus sp. strain PCC 7942 involved in active transport of bicarbonate. J Biol Chem 2000;275:20551–5
- Smith KS, Ferry JG. Prokaryotic carbonic anhydrases. FEMS Microbiol Rev 2000;24:335–66
- Pearson WR. Using the FASTA program to search protein and DNA sequence databases. Methods Mol Biol 1994;24:307–31
- Supuran CT, Di Fiore A, Alterio V, et al. Recent advances in structural studies of the carbonic anhydrase family: the crystal structure of human CA IX and CA XIII. Curr Pharm Des 2010;16:3246–54
- Supuran CT. Enzyme Inhibition and more – a tribute to John Smith. J Enzyme Inhib Med Chem 2011;26:301–2
- Supuran CT. Carbonic anhydrase inhibition with natural products: novel chemotypes and inhibition mechanisms. Mol Divers 2011;15:305–16
- Vullo D, Luca VD, Scozzafava A, et al. The alpha-carbonic anhydrase from the thermophilic bacterium Sulfurihydrogenibium yellowstonense YO3AOP1 is highly susceptible to inhibition by sulfonamides. Bioorg Med Chem 2012; doi: 10.1016/j.bmcl.2012.08.088 [Epub ahead of print]
- De Luca V, Vullo D, Scozzafava A, et al. Anion inhibition studies of an alpha-carbonic anhydrase from the thermophilic bacterium Sulfurihydrogenibium yellowstonense YO3AOP1. Bioorg Med Chem Lett 2012;22:5630–4
- Capasso C, De Luca V, Carginale V, et al. Biochemical properties of a novel and highly thermostable bacterial alpha-carbonic anhydrase from Sulfurihydrogenibium yellowstonense YO3AOP1. J Enzyme Inhib Med Chem 2012;27:892–7
- Supuran CT. Therapeutic compounds: patent evaluation of WO2011011652A1. Expert Opin Ther Pat 2011;21:1491–5
- Maresca A, Scozzafava A, Kohler S, et al. Inhibition of beta-carbonic anhydrases from the bacterial pathogen Brucella suis with inorganic anions. J Inorg Biochem 2012;110:36–9
- Joseph P, Ouahrani-Bettache S, Montero JL, et al. A new beta-carbonic anhydrase from Brucella suis, its cloning, characterization, and inhibition with sulfonamides and sulfamates, leading to impaired pathogen growth. Bioorg Med Chem 2011;19:1172–8
- Maresca A, Vullo D, Scozzafava A, et al. Inhibition of the beta-class carbonic anhydrases from Mycobacterium tuberculosis with carboxylic acids. J Enzyme Inhib Med Chem 2012; doi: 10.3109/14756366.2011.650168 [Epub ahead of print]
- Nishimori I, Minakuchi T, Maresca A, et al. The beta-carbonic anhydrases from Mycobacterium tuberculosis as drug targets. Curr Pharm Des 2010;16:3300–9
- Burghout P, Vullo D, Scozzafava A, et al. Inhibition of the beta-carbonic anhydrase from Streptococcus pneumoniae by inorganic anions and small molecules: toward innovative drug design of antiinfectives? Bioorg Med Chem 2011;19:243–8
- Nishimori I, Onishi S, Takeuchi H, Supuran CT. The alpha and beta classes carbonic anhydrases from Helicobacter pylori as novel drug targets. Curr Pharm Des 2008;14:622–30
- Nishimori I, Minakuchi T, Vullo D, et al. Inhibition studies of the beta-carbonic anhydrases from the bacterial pathogen Salmonella enterica serovar Typhimurium with sulfonamides and sulfamates. Bioorg Med Chem 2011;19:5023–30
- Luca VD, Vullo D, Scozzafava A, et al. An α-carbonic anhydrase from the thermophilic bacterium Sulphurihydrogenibium azorense is the fastest enzyme known for the CO(2) hydration reaction. Bioorg Med Chem 2012; doi: 10.1016/j.bmc.2012.09.047 [Epub ahead of print]
- Abuaita BH, Withey JH. Bicarbonate induces Vibrio cholerae virulence gene expression by enhancing ToxT activity. Infect Immun 2009;77:4111–20
- Laemmli UK, Johnson RA. Maturation of the head of bacteriophage T4. II. Head-related, aberrant tau-particles. J Mol Biol 1973;80:601–11
- Chirica LC, Elleby B, Jonsson BH, Lindskog S. The complete sequence, expression in Escherichia coli, purification and some properties of carbonic anhydrase from Neisseria gonorrhoeae. Eur J Biochem 1997;244:755–60
- Armstrong JM, Myers DV, Verpoorte JA, Edsall JT. Purification and properties of human erythrocyte carbonic anhydrases. J Biol Chem 1966;241:5137–49
- Myers JK, Pace CN, Scholtz JM. Denaturant m values and heat capacity changes: relation to changes in accessible surface areas of protein unfolding. Protein Sci 1995;4:2138–48
- Cox GM, Mukherjee J, Cole GT, et al. Urease as a virulence factor in experimental cryptococcosis. Infect Immun 2000;68:443–8
- Smith KS, Jakubzick C, Whittam TS, Ferry JG. Carbonic anhydrase is an ancient enzyme widespread in prokaryotes. Proc Natl Acad Sci USA 1999;96:15184–9
- Valdivia RH, Falkow S. Fluorescence-based isolation of bacterial genes expressed within host cells. Science 1997;277:2007–11
- Caparon MG, Geist RT, Perez-Casal J, Scott JR. Environmental regulation of virulence in group A streptococci: transcription of the gene encoding M protein is stimulated by carbon dioxide. J Bacteriol 1992;174:5693–701
- Abe H, Tatsuno I, Tobe T, et al. Bicarbonate ion stimulates the expression of locus of enterocyte effacement-encoded genes in enterohemorrhagic Escherichia coli O157:H7. Infect Immun 2002;70:3500–9
- Yang J, Dogovski C, Hocking D, et al. Bicarbonate-mediated stimulation of RegA, the global virulence regulator from Citrobacter rodentium. J Mol Biol 2009;394:591–9
- Yang J, Hart E, Tauschek M, et al. Bicarbonate-mediated transcriptional activation of divergent operons by the virulence regulatory protein, RegA, from Citrobacter rodentium. Mol Microbiol 2008;68:314–27
- Abbate F, Casini A, Scozzafava A, Supuran CT. Carbonic anhydrase inhibitors: X-ray crystallographic structure of the adduct of human isozyme II with a topically acting antiglaucoma sulfonamide. Bioorg Med Chem Lett 2004;14:2357–61
- Carta F, Scozzafava A, Supuran CT. Sulfonamides: a patent review (2008–2012). Expert Opin Ther Pat 2012;22:747–58