Abstract
Two series of 8-alkoxy-4,5-dihydrobenzo[b][1,2,4]triazolo[4,3-d][1,4]thiazepine derivatives (6a–q and 7a–q) were synthesized and evaluated for their anticonvulsant activity using the maximal electroshock (MES) method. All of the compounds prepared were effective in the MES screens. Among which, compound 7j was considered as the most promising one with an ED50 value of 26.3 mg/kg and a superior protective index value of 12.6. The potency of compound 7j against seizures induced by pentylenetetrazole, 3-mercaptopropionic acid and bicuculline suggested that two different mechanisms of action might potentially be involved in its anticonvulsant activity, including the inhibition of voltage-gated ion channels and the modulation of GABAergic activity. A computational study was also conducted to predict the pharmacokinetic properties of the compounds prepared, with the results supporting the use of these compounds as a group of promising antiepileptic agents.
Introduction
Epilepsy is one of the most common neurological disorders, and is characterized by excessive temporary neuronal discharge resulting in recurrent unprovoked seizuresCitation1,Citation2. It has been reported that about 1% of the world’s population (about 50 million people worldwide) are suffering with this neurological disorder at any one timeCitation2. In recent years, significant efforts have been invested in the development of novel therapeutics, resulting in the emergence of several novel drugs as promising anticonvulsant agentsCitation3,Citation4. The anticonvulsants currently available for the treatment of this disorder, however, can only reduce the severity and number of seizures in less than 70% of patients. Moreover, their usage is often associated with undesirable and numerous side effectsCitation5–7. The current antiepileptic drugs (AEDs) used widely, such as phenytoin, carbamazepine, ethosuximide, valproic acid and barbiturates, produce many adverse side effects, including ataxia, hepatotoxicity, gingival hyperplasia and megaloblastic anaemiaCitation8,Citation9. High levels of toxicity and intolerance, and a lack of efficacy also represent further limitations of the current AEDs. With all of this in mind, there is an urgent need for the development of novel AEDs with higher levels of potency and lower levels of toxicity.
The lack of information pertaining to the cellular mechanism of epilepsy in humans together with the complex mechanisms of the AEDs makes it difficult to apply rational methodologies in the field of AED discovery. Fortunately, a design method for the development of new antiepileptic agents based on the existence of different pharmacophores has been established through the analysis of different structural characteristics of clinically effective drugs as well as other antiepileptic compoundsCitation10–13. Several key pharmacophoric elements essential for good anticonvulsant activity have been identified and well documented in the literatureCitation13–15, including a hydrophobic unit (A), an electron donor group (D) and a hydrogen donor/acceptor unit (HAD) (). With the recent development of novel therapeutics based on the pharmacophore model, several new drugs (including tiagabine, lamotrigine, pregabalin, stiripentol, zonisamide, topiramate and levetiracetam) have already reached the market as promising antiepileptic agentsCitation16.
Figure 1. The vital structural features included in well-known antiepileptic agents and target compounds: hydrophobic unit (A), electron donor group (D), HAD unit.
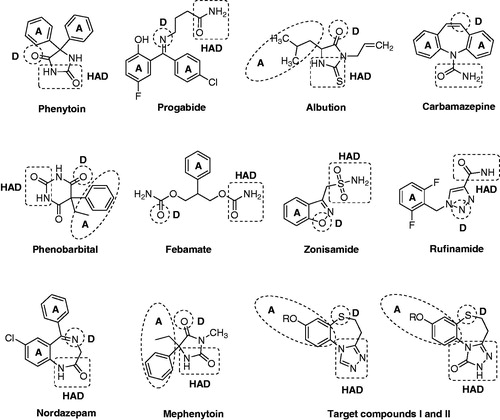
The 1,2,4-triazole nucleus has been incorporated into a wide variety of therapeutically important agents, such as antimicrobialCitation17–19, antiviral agentsCitation20, anti-HIVCitation21,Citation22 and anti-inflammatoryCitation23. Moreover, the chemistry of 1,2,4-triazoles and their fused heterocyclic derivatives has also received considerable attention owing to their synthetic and biological importance. In our previous work, we reported the synthesis and bioactivity evaluation of several series of compounds with a triazole ring. All of them exhibited a certain degree of anticonvulsant activityCitation24–30. Among which, 7-alkoxy-4H-[1,2,4]triazolo[4,3-d]benzo[b][1,4]thiazines (I) were found to exhibit the most remarkable levels of anticonvulsant activitiesCitation29. Herein, as part of our ongoing research towards the development of novel anticonvulsant agents, we described the design and synthesis of a series of 8-alkoxy-4,5-dihydrobenzo[b][1,2,4]triazolo[4,3-d][1,4]thiazepines (6a–q) using compounds I (, I) as the lead compound. Compounds 6a–q (, II) were the ring-expanded analogues of compounds I. Furthermore, our previous investigations suggested that the triazolone ring was relevant to the observed levels of activity. So, a series of 8-alkoxy-4,5-dihydrobenzo[b][1,2,4]triazolo[4,3-d][1,4]thiazepin-1(2H)-ones (7a–q) were also been synthesized (, III) with the aim of identifying promising compounds and establishing some structure–activity relationships (SARs). As shown in , all four of the essential pharmacophoric elements mentioned above were successfully incorporated into the compounds synthesized.
The newly synthesized compounds were characterized by IR, 1H and 13C NMR, mass spectral and elemental analyses. Their anticonvulsant activities and neurotoxicities were evaluated using the maximal electroshock (MES)-induced seizure model and the rotarod assay in mice, respectively. To effectively elucidate the possible mechanism of action of these compounds, the most active compound 7j was tested in pentylenetetrazole (PTZ), 3-mercaptopropionic acid (3-MP) and bicuculline (BIC)-induced seizure tests. Furthermore, a computational study was conducted for predicting the ADME properties, toxicity risks and druglikeness of all the synthesized compounds.
Experimental protocols
Chemistry
Melting points were determined in open capillary tubes and were uncorrected. IR spectra were recorded (in KBr) on IRPrestige-21. 1H-NMR and 13C-NMR spectra were measured on an AV-300 (Bruker, Fällanden, Switzerland), and all chemical shifts were given in ppm relative to tetramethysilane. Mass spectra were measured on an HP1100LC (Agilent Technologies, Santa Clara, CA). Elemental analyses were performed on a 204Q CHN (Perkin Elmer, Cambridge, MA). Microanalyses of C, N and H were performed using a Heraeus CHN Rapid Analyzer (Perkin Elmer, Waltham, MA). The major chemicals were purchased from Aldrich Chemical Corporation (St. Louis, MO).
Synthesis of 2-amino-5-methoxybenzenethiol (1)
A mixture of 6-methoxybenzo[d]thiazol-2-amine (6.00 g, 33 mmol) and 50% KOH solution (40 mL) was refluxed for 16 h. After cooling, the solution was diluted with water (50 mL) and filtered. The filtrated was neutralized with acetic acid to pH 6. The precipitate formed was filtered and washed with water. The crude product quickly dried in drying oven was subjected into the next reaction immediately. Yield 5.12 g (81%); m.p. 74–76 °C; 1H NMR (CDCl3, 300 MHz) δ 3.77 (s, 3 H, OCH3), 6.43 (dd, 1H, J = 2.6, 8.5 Hz, Ar-H), 6.57 (d, 1H, J = 8.5 Hz, Ar-H), 6.66 (d, 1H, J = 2.6 Hz, Ar-H).
Synthesis of 8-methoxy-2,3-dihydro-1,5-benzothiazepin-4(5H)-one (2)
A mixture of freshly prepared 6-methoxybenzo[d]thiazol-2-amine (1; 3.1 g, 20 mmol), acrylic acid (1.6 mL, 24 mmol) and toluene (40 mL) was heated to reflux for 6 h. After cooling, the crystal formed (black green) was filtered off, and washed with methanol. Yield 1.9 g (45%); m.p. 208–210 °C; 1H-NMR (CDCl3, 300 MHz), δ 2.40 (t, 2H, J = 6.8 Hz, COCH2), 3.35 (t, 2H, J = 6.8 Hz, SCH2), 3.74 (s, 3H, OCH3), 6.93–7.09 (m, 3H, Ar-H), 9.54 (s, 1H, CONH). IR (KBr) cm−1: 3219 (N-H), 1707 (C=O). MS m/z 210 (M + H).
Synthesis of 8-hydroxy-2,3-dihydro-1,5-benzothiazepin-4(5H)-one (3)
8-Methoxy-2,3-dihydro-1,5-benzothiazepin-4(5H)-one (2; 2.09 g, 10 mmol) was dissolved in 60 mL dichloromethane. BBr3 (2.78 mL, 30 mmol) was added dropwise to the solution and the mixture was stirred at room temperature. After 4 h, 20 mL ice water was added slowly into the mixture and allowed to stir for half an hour. After removing the dichloromethane under reduced pressure, the resulting white precipitate was obtained by filtration. Yield 1.85 g (95%); m.p. 247–249 °C; 1H-NMR (CDCl3, 300 MHz), δ 2.37 (t, 2H, J = 6.5 Hz, COCH2), 3.31 (t, 2H, J = 6.5 Hz, SCH2), 6.72–6.93 (m, 3H, Ar-H), 9.44 (s, 1H, Ar-OH). 9.59 (s, 1H, CONH). MS m/z 196 (M + H).
General procedure for the synthesis of 8-alkoxy-2,3-dihydro-1,5-benzothiazepin-4(5H)-one (4a–q)
K2CO3 (1.24 g, 10 mmol) and 8-hydroxy-2,3-dihydro-1,5-benzothiazepin-4(5H)-one (3; 5 mmol) were dissolved in acetonitrile (60 mL) and refluxed for 30 min, then the appropriate alkyl bromide or benzyl chloride (6 mmol) was added to the mixture. The reaction mixture was heated at reflux temperature for 4–24 h. After removing the most solvent, 100 mL of water was poured into the flask and the precipitate formed was filtered. Yield 79–88%. Characterization for 4a: Yield 81%; m.p. 156–158 °C; 1H-NMR (CDCl3, 300 MHz), δ 1.05 (t, 3H, J = 7.4 Hz, CH3), 1.76–1.88 (m, 2H, CH2CH3), 2.62 (t, 2H, J = 6.9 Hz, COCH2), 3.44 (t, 2H, J = 6.9 Hz, SCH2), 3.93 (t, 2H, J = 6.5 Hz, OCH2), 6.89 (dd, 1H, J1 = 2.8 Hz, J2 = 8.7 Hz, Ar-H), 7.04 (d, 1H, J = 8.7 Hz, Ar-H), 7.15 (d, 1H, J = 2.8 Hz, Ar-H), 8.03 (s, 1H, CONH). MS m/z 238 (M + H).
General procedure for the synthesis of 8-alkoxy-2,3-dihydro-1,5-benzothiazepin-4(5H)-thione (5a–q)
A mixture of crude 8-alkoxy-2,3-dihydro-1,5-benzothiazepin-4(5H)-one (4a–q; 4 mmol), Lawesson's reagent (0.97 g, 2.4 mmol) and toluene (40 mL) was heated to reflux for 2–4 h. The excess solvent was removed under reduced pressure, and the residue was purified by silica gel column chromatography with CH2Cl2–ether (2:1) to a white solid. Yield 65–74%. Characterization for 5a: Yield 69%; m.p. 134-136 °C; 1H-NMR (CDCl3, 300 MHz), δ 1.05 (t, 3H, J = 7.4 Hz, CH3), 1.77–1.89 (m, 2H, CH2CH3), 3.08 (t, 2H, J = 6.6 Hz, COCH2), 3.55 (t, 2H, J = 6.6 Hz, SCH2), 3.94 (t, 2H, J = 6.5 Hz, OCH2), 6.90 (dd, 1H, J1 = 2.7 Hz, J2 = 8.7 Hz, Ar-H), 7.08 (d, 1H, J = 8.7 Hz, Ar-H), 7.15 (d, 1H, J = 2.7 Hz, Ar-H), 9.86 (s, 1H, CONH). MS m/z 254 (M + H).
General procedure for the synthesis of 8-alkoxy-4,5-dihydrobenzo[b][1,2,4]triazolo[4,3-d][1,4]thiazepine (6a–q)
A solution of 5a–q (3 mmol) in appropriate n-butanol and formyl hydrazine (6 mmol) was refluxed for 2–3 d (TLC monitoring), the solvent was evaporated to dryness under reduced pressure, and the residue poured by water (50 mL) was extracted twice with dichloromethane (60 mL). The dichloromethane layer was washed three times with saturated aqueous NaCl (60 mL × 3) and dried over anhydrous MgSO4. After removing the solvents, products were purified by silica gel column chromatography with CH2Cl2–CH3OH (70:1). Characterization for 6a: m.p. 126–128 °C, yield 56%. 1H-NMR (CDCl3, 300 MHz) δ 1.05 (t, 3H, J = 7.4 Hz, CH3), 1.76–1.88 (m, 2H, CH2CH3), 3.15 (t, 2H, J = 6.9 Hz, CNCH2), 3.43 (t, 2H, J = 6.9 Hz, SCH2), 3.96 (t, J = 6.5 Hz, 2H, OCH2), 6.97–7.29 (m, 3H, Ar-H), 8.28 (s, 1H, triazole-H). IR (KBr) cm−1: 1601 1501 (N=C). MS m/z 262 (M + H). Anal Calcd for C13H15N3OS (%): C, 59.74; H, 5.79; N, 16.08. Found (%): C, 59.61; H, 5.88; N, 16.25. The characterizations of the other compounds (6b–q) are available in Supplementary material.
General procedure for the synthesis of 8-alkoxy-4,5-dihydrobenzo[b][1,2,4]triazolo[4,3-d][1,4]thiazepin-1(2H)-one (7a–q)
A solution of 5a–q (3 mmol) in appropriate n-butanol and methyl hydrazinecarboxylate (6 mmol) was refluxed for 2–3 d (TLC monitoring), the solvent was evaporated to dryness under reduced pressure, and the residue poured by water (50 mL) was extracted twice with dichloromethane (60 mL). The dichloromethane layer was washed three times with saturated aqueous NaCl (60 mL × 3) and dried over anhydrous MgSO4. After removing the solvents, products were purified by silica gel column chromatography with CH2Cl2–CH3OH (70:1). Characterization for 7a: m.p. 136–138 °C, yield 48%. 1H-NMR (CDCl3, 300 MHz) δ 1.03 (t, 3H, J = 7.4 Hz, CH3), 1.79–1.84 (m, 2H, CH2CH3), 2.86 (t, 2H, J = 6.8 Hz, CNCH2), 3.27 (t, 2H, J = 6.8 Hz, SCH2), 3.96 (t, J = 6.4 Hz, 2H, OCH2), 6.99–7.55 (m, 3H, Ar-H), 10.16 (s, 1H, CONH). IR (KBr) cm−1: 3291 (N-H), 1697 (C=O), 1601 (N=C). MS m/z 278 (M + H). Anal Calcd for C13H15N3O2S (%): C, 56.30; H, 5.45; N, 15.15. Found (%): C, 56.02; H, 5.69; N, 15.37. The characterizations of the other compounds (7b–q) seen in Supplementary material.
Pharmacology
MES seizure test
The MES test was carried out according to the methods described in the ADD of the National Institutes of Health (USA)Citation31,Citation32. Seizures were elicited with a 60 Hz alternating current of 50 mA intensity in mice. The current was applied via corneal electrodes for 0.2 s. Protection against the spread of MES-induced seizures was defined as the absence of tonic extension of the hind leg a. After 0.5 and 4.0 h of drug administration, the activities were evaluated in MES test. In phase-I screening, each compound was administered at the dose levels of 30, 100 and 300 mg/kg for evaluating the preliminary anticonvulsant activity. For determination of the median effective dose (ED50) the median toxic dose (TD50), the phase-II screening was prepared. Groups of 10 mice were given a range of intraperitoneal doses of the tested compound until at least three points were established in the range of 10–90% seizure protection (or neurotoxicity, NT). From these data, the respective ED50, TD50 values, and 95% confidence intervals were calculated by probit analysis.
NT screening
The NT of the compounds was measured in mice by the rotarod testCitation31,Citation32. The mice were trained to stay on an accelerating rotarod of diameter 3.2 cm that rotates at 10 rpm. Trained animals were given an intraperitoneal injection of the test compounds. NT was indicated by the inability of the animal to maintain equilibrium on the rod for at least 1 min in each of the trials.
Chemical induced seizure tests
In chemically induced seizures (Table 4, Supplementary material), mice were given doses of convulsants that could induce seizures at least 97% of animals. The doses used were: PTZ, 85 mg/kg; 3-MP acid, 40 mg/kg and BIC, 5.4 mg/kg. The test compounds and standard AEDs were administered as an intraperitoneal (i.p.) injection in a volume of 30 mg/kg to groups of ten mice 30 min before injection of PTZ (subcutaneously), 3-MP acid (i.p.) and BIC (subcutaneously). The mice were placed in individual cages and observed for 30 min, the number of clonic seizures, tonic seizures and the lethality were recordedCitation33,Citation34.
Computational study
Prediction of ADME properties
A computational study of titled compounds was performed for the prediction of ADME properties. Polar surface area (PSA), miLog P, number of rotatable bonds, molecular volume, number of hydrogen donor and acceptor atoms and violations of Lipinski’s rule of fiveCitation35 were calculated using Molinspiration online property calculation toolkitCitation36. Absorption (%ABS) was calculated by: % ABS = 109 – (0.345 × PSA)Citation37.
Toxicity risks prediction and drug-score
The toxicity prediction and drug-score of the compounds were calculated using Osiris property calculatorCitation38.
Results and discussion
Chemistry
All of the target compounds were synthesized according to the route depicted in , with 6-methoxybenzo[d]thiazol-2-amine being used as the starting material. Briefly, the starting material was hydrolyzed in a 50% aqueous KOH solution to afford 2-amino-5-methoxybenzenethiol (1). It is noteworthy that compound 1 was unstable and was therefore immediately progressed into the subsequent reactionCitation39. Compound 1 was treated with acrylic acid in toluene for 6 h to provide 8-methoxy-2,3-dihydro-1,5-benzothiazepin-4(5H)-one (2). The structure of compound 2 was confirmed by its 1H NMR spectrum, which clearly revealed the resonance signals of the amide (9.54 ppm) and methylene groups (2.40 and 3.35 ppm), in accordance with the proposed structure. Unfortunately, however, the yield for this reaction was poor (the best is 45% in our lab), because of the occurrence of several competing side-reactions (). Compound 2 was then demethyled with boron tribromide to give 8-hydroxy-2,3-dihydro-1,5-benzothiazepin-4(5H)-one (3), which was subsequently alkylated with a variety of different alkylating agents to afford the corresponding 8-alkoxy-2,3-dihydro-1,5-benzothiazepin-4(5H)-one (4a–q). Compounds 4a–q were then treated with Lawesson's reagent to provide the key 8-alkoxy-2,3-dihydro-1,5-benzothiazepin-4(5H)-thione intermediates (5a–q). Finally, the target compounds 6a–q and 7a–q were obtained following the treatment of the key intermediates 5a–q with formyl hydrazine and methyl hydrazinecarboxylate, respectively.
Their chemical structures were characterized by IR, 1H and 13C NMR, mass spectral and elemental analyses. The detailed physical and analytical data are listed in experimental protocols.
Biological evaluation
Phase I evaluation of anticonvulsant activity
The choice of an appropriate animal model for the initial compound screening process represents a very important step in AED discovery. There are currently three models available for the in vivo evaluation of the anticonvulsant activity of a drug candidate, including the MES, subcutaneous PTZ (scPTZ) and kindling models, which are routinely used by most AED discovery programs. Among these tests, the MES and scPTZ seizure models represent the two animal seizure models most widely used in the search for new AEDsCitation40,Citation41. In the present study, the MES seizure model was selected to screen the anticonvulsant activities of target compounds.
In the preliminary screening process (MES and rotarod tests), each compound was administered (i.p.) at three different dose levels, including 30, 100 and 300 mg/kg. Anticonvulsant and neurotoxic effects were assessed at 0.5 and 4 h intervals after administration in mice. The results obtained are listed in .
Table 1. Phase-I preliminary evaluation of anticonvulsant activity in mice (i.p.)
As shown in , all the compounds synthesized were active when administered at the dose of 300 mg/kg in the MES test. At the dose of 100 mg/kg, most of the compounds, with the exception of compounds 6h, 6i, 6n, 6p, 6q and 7n, showed some degree of protection at time period 0.5 h. At the dose of 30 mg/kg, compounds 6j, 6m, 7b, 7f and 7i exhibited protection in only 1/3 of the mice, whereas compounds 6d, 6g, 7c–g and 7j exhibited protection in 2/3 at time period 0.5 h. None of the compounds showed protection at the 4 h period, indicating that they all had a rapid onset and short duration of anticonvulsant activity.
In the NT screen, all of the synthesized compounds exhibited neurotoxic effects at the high dose of 300 mg/kg at 0.5 h. With the exception of compounds 6g, 6m, 6o, 7b–e, 7k, 7o and 7q, the majority of the compounds did not show any NT at the dose of 100 mg/kg at 0.5 h. At the 4 h period, no NT could be detected.
Phase II evaluation of anticonvulsant activity
On the basis of the results recorded in the phase I test, compounds 6d, 6g, 7c–g and 7j were subjected to phase II trials for quantification of their anticonvulsant activities (indicated by ED50) and neurotoxicities (indicated by TD50) in mice. The results of the quantitative tests for the selected compounds, together with the corresponding data for the currently marketed AEDs, including carbamazepine, phenobarbital and valproate, are shown in . Compound 6g was found to be the most active of this group of compounds with an ED50 value of 20.7 mg/kg. Unfortunately, compound 6g also showed a high level of NT with a TD50 value of 163.7 mg/kg, which consequently resulted in a protective index (PI) value of 7.9. Considering all these factors (i.e. efficacy, toxicity, and safety), compound 7j was considered to be the most promising compound in this study owing to its favorable efficacy and low NT (ED50 = 26.3 mg/kg and TD50 = 331.3 mg/kg, resulting in a PI value of 12.6). It is noteworthy that all of the selected compounds showed a higher PI than the standard drugs, except the compound 7c (PI value of 4.3).
Table 2. Phase-II quantitative anticonvulsant evaluation in Mice (i.p.).
Structure activity relationships
In the present work, we synthesized two series of compounds including 8-alkoxy-4,5-dihydrobenzo[b][1,2,4]triazolo[4,3-d][1,4]thiazepines (6a–q) and 8-alkoxy-4,5-dihydrobenzo[b][1,2,4]triazolo[4,3-d][1,4]thiazepin-1(2H)-ones (7a–q). Analyzing the activities of the synthesized compounds, the following SARs were obtained. Among compounds 6a–q, compounds 6a–f possessed different alkoxyl group attached to the core triazolobenzodiazepine fragment, ranging from a propoxy group to an octyloxy group. The length of the alkyl chain appeared to have an impact on the anticonvulsant activity of the derivatives. For example, as the alkyl chain length increased from 6a to 6d, the anticonvulsant activity gradually increased with compound 6d (n-hexane chain) being the most active. From compound 6d–f, although the alkyl chain length increased further, the anticonvulsant activity decreased, suggesting that the n-hexane chain was optimal. Compound 6d was the most active compound with an ED50 value of 23.7 mg/kg, indicating the optimal partition coefficient associated with the easiest crossing of the biological membranes in the congeners. Compound 6g was substituted with a benzyloxy group at the 8-position of the triazolobenzodiazepine core and F, Cl, Br and CH3 groups were subsequently added on to the benzene ring of the benzyloxy group of 6g at different positions providing compounds 6h–q. Unfortunately, the introduction of different halogen or methyl groups to the phenyl ring led to a reduction in the anticonvulsant activity in comparison with derivative 6g. For the three F-substituted derivatives (6h–j), a potency order of p-F > m-F = o-F was observed. Of the four Cl-substituted derivatives (6k–n), a potency order of p-Cl > o-Cl > m-Cl > 2,4-Cl2 was observed. Between the two Br-substituted derivatives (6o, 6p), the potency order was o-Br > p-Br. The SARs of compounds 7a–q were on the whole similar to those of 6a–q. For the alkoxyl group substituted compounds (7a–f), the trend in activity was the same as that observed in compounds 6a–q, with compound 7d (with an n-hexane alkyl chain) being identified as the most active compound in the series. For compounds 7g–q, substituted with a benzyloxy groups that were either halogenated or non-halogenated on their phenyl ring, the p-F substituted compound 7j was found to be the most active with an ED50 value of 26.3 mg/kg, and also exhibited a higher level of activity than the non-substituted compound 7g (ED50 = 36.7 mg/kg). The other compounds (7h–i and 7k–q) all led to a reduction in anticonvulsant activity when compared to derivative 7g. The influence of the position of the halogens on the phenyl ring was also similar to that of series 6a–q. For the three F-substituted derivatives (7h–j), the potency order was p-F > m-F > o-F. For the four Cl-substituted derivatives (7k–n), the potency order was p-Cl = o-Cl > m-Cl > 2,4-Cl2. The potency order of the two Br-substituted derivatives (6o, 6p) was o-Br > p-Br. Comparing the compounds 6a–q and 7a–q, no significant difference was found with regard of anticonvultant activity and toxicity. The additional C=O of compounds 7a–q seems not to influence the anticonvulsant activity.
Chemical-induced seizure tests
In this study, the majority of synthesized compounds were highly potent in the MES test. As the MES test is known to be sensitive to sodium channel inhibitors (e.g. phenytoin, carbamazepine), the tested compounds may inhibit voltage-gated ion channels (particularly sodium channels). To further investigate the effects of the anticonvulsant activity in several different models and speculate about the possible mechanism action of anticonvulsant, compound 7j was tested against convulsions induced by chemical substances, including PTZ, 3-MP and BIC. The reasons for the chose of compound 7j are its higher PI value than others and its good profile in toxicity and druglikeness prediction. Compound 7j was administered as an intraperitoneal injection to mice at a dose of 50 mg/kg, which was higher than its ED50 value and far below its TD50 value. The reference drug carbamazepine was also administered at the dose of 50 mg/kg.
In the sc-PTZ model, carbamazepine inhibited the clonic seizures, tonic seizures and death at the rates of 0%, 100% and 90%, respectively. While compound 7j inhibited clonic seizures, tonic seizures and lethality at the rates of 10%, 80% and 90% induced by sc-PTZ, respectively (). The data showed that compound 7j and carbamazepine can protect the mice from tonic seizures and death overwhelmingly, which revealed that compound 7j possessed excellent activity in sc-PTZ model. PTZ has been reported to produce seizures by inhibiting γ-aminobutyric acid (GABA) neurotransmissionCitation42,Citation43. GABA is the main inhibitory neurotransmitter in the brain, and is widely implicated in epilepsy. Inhibition of GABAergic neurotransmission or activity has been shown to promote and facilitate seizuresCitation44, while enhancement of GABAergic neurotransmission is known to inhibit or attenuate seizures. As such, our newly synthesized compound 7j might inhibit or attenuate PTZ-induced seizures in mice by enhancing GABAergic neurotransmission.
Table 3. Effects of compound 7j on chemical-induced seizures in mice (i.p.).
In the 3-MP induced seizure model, carbamazepine inhibited the clonic seizures, tonic seizures and death at the rates of 0%, 80% and 90%, respectively. In comparison, compounds 7j showed an anticonvulsant effect similar to that of carbamazepine in inhibiting the clonic and tonic seizures; however, its effect on death rates induced by 3-MP was less, with the inhibition rate of 30% (). 3-MP is competitive inhibitor of the GABA synthesis enzyme glutamate decarboxylase (GAD), and it inhibits the synthesis of GABA resulting in decrease of GABA levels in the brainCitation45. Compound 7j showed antagonism to 3-MP induced seizures suggesting that it might activate GAD or inhibit aminotransferase (GABA-T) in the brain.
In the BIC induced seizure model, both carbamazepine and 7j inhibited tonic seizures and death, but did not inhibit clonic seizures. Carbamazepine showed inhibition of clonic and tonic seizures and death at rates of 0%, 100% and 40%, respectively. Compound 7j showed inhibition of clonic and tonic seizures and death at rates of 10%, 90% and 50%, respectively (). BIC is a competitive antagonist of GABAA receptor, which produces convulsions through its interference of the GABAA receptorCitation46. As compound 7j inhibited the seizures induced by BIC, it likely exerts anticonvulsant activity, at least partially, through GABAA-mediated mechanisms.
Computational study
Prediction of ADME properties
A computational study was conducted to predict the ADME properties of all of the synthesized compounds (Table 4, Supplementary material). Two significant barriers exist in the human body, with one of these being the intestinal epithelium, which modulate drug absorption following oral administration. With the exception of medications interrupting status epilepticus, most of the AEDs are administered orally, and newly synthesized antiepileptic substances should therefore be efficiently absorbed in the digestive system. It has been demonstrated experimentally that the intestinal absorption of drugs is significantly correlated with their PSA. The PSA of a molecule effectively represent the portion of its surface belonging to polar atoms, such as oxygen, nitrogen and attached hydrogen atoms, and is a descriptor related to the passive molecular transport of a molecule through membranes. With this in mind, PSA could be used to predict the transport properties of drugs in the intestinesCitation47. Palm et al.Citation48 have proven, based on Caco-2 cell studies, that drugs with a PSA value below 60 were completely absorbed in the intestine. For the target compounds 6a–q and 7a–q, the PSA values were ranged from 39.9 to 59.9 Å2 and the level of intestinal absorption (%ABS) according to the algorithm described by Zhao et al.Citation37 was in the range of 88.3–95.2%. These findings indicated that these compounds (6a–q and 7a–q) would possess good transport properties in the intestines.
The blood-brain barrier (BBB) represents the second of the two significant barriers in the human body, and is responsible for regulating the influx of different chemical compounds into the brain. The permeability (or lack thereof) of the BBB affects drug efficiency in the central nervous system. Chemical substances may be transported to the brain by means of passive and facilitated diffusion processes or active transportCitation49. According to Kaliszan & MarkuszewskiCitation50, the lipophilicity (LogP) and molecular weight (MW) of a compound are the main factors affecting the delivery of a drug across the BBB. Lipophilicity affects the absorption of a drug, hydrophobic drug-receptor interactions, drug metabolism and drug toxicity. It has been reported that a LogP value of 2.0 represent the optimum value for drugs acting on the central nervous systemCitation51. Drugs possessing this value will be inhibited to a lesser extent in their movement through the aqueous and lipophilic phases of living tissues. From the calculated LogP parameters, it is clear that all of the synthesized compounds exhibited LogP values in the range of 2.0–4.9, which were close to the optimum LogP value of 2.0. These investigations suggested that the compounds reported here would possess good pharmacokinetic properties. The “Rule of 5” was established as a set of simple molecular descriptors by Lipinski based on the observation that most drugs are relatively small and lipophilic moleculesCitation35. This rule states that most “drug-like” molecules have common parameters, including LogP ≤ 5, MW ≤ 500, number of hydrogen bond acceptors ≤10, and number of hydrogen bond donors ≤5. Molecules violating more than one of these rules may have problems with bioavailability. Compounds 6a–q and 7a–q obeyed all of Lipinski’s parameters.
Toxicity risks prediction and drug-score
The risk of the occurrence of toxic effects following the administration of the eight superior derivatives (6d, 6g, 7c–g, 7j) was estimated using the Osiris software. A series of so-called drug-score parameters, which measure the probability of the application of an active compound as a potential drug, were also calculated (Table 5, Supplementary material). The toxicity risk assessment revealed that all of the compounds investigated were non-tumorigenic, non-mutagenic and showed no adverse reproductive effects. The results suggested, however, that compounds 6d, 7c and 7d possessed a high risk of producing an irritation effect, whereas compound 7e only possessed a moderate risk of producing an irritation effect. Of course, computationally predicted toxicity is not completely reliable and is just another tool for identifying potential promising drug candidates with greater ease and speed. In turn, the drug-score is estimated on the basis of a combination of several different factors, including drug-likeness, toxicity risks, LogS, cLogP and MW, to provide one handy value that may be used to judge the potential of any molecule to qualify as a drug. Based on the data presented in Table 5, compound 7j (with the higher score of 0.57 compared to others) was identified as the most promising antiepileptic agent of the current series, which was also in good agreement with the previous results for the evaluation of its anticonvulsant activity and NT.
Conclusion
Based on our previous work, we synthesized two novel series of 8-alkoxy-4,5-dihydrobenzo[b][1,2,4]triazolo[4,3-d][1,4]thiazepine derivatives (6a–q and 7a–q) and evaluated their anticonvulsant activities. The compounds tested in the MES screens were effective at various doses, with the most promising compound being identified as 8-(4-fluorobenzyloxy)-4,5-dihydrobenzo[b][1,2,4]triazolo[4,3-d][1,4]thiazepin-1(2H)-one (7j), which showed an ED50 value of 26.3 mg/kg and a superior PI value of 12.6 when compared to standard drugs in the MES test in mice. Moreover, the potencies of compound 7j against seizures induced by PTZ, 3-MP acid and BIC were also established, with the results suggesting that several different mechanisms of action were involved in its anticonvulsant activity, including the inhibition of voltage-gated ion channels and the modulation of GABAergic activity. A computational study was also carried out to predict the pharmacokinetic properties of the synthesized compounds, with the results effectively supporting the potential of these compounds as a group of promising antiepileptic agents.
Declaration of Interest
We declare no conflict of interest.
This work was supported by the National Natural Science Foundation of China (No. 81160382) and National Science and Technology Major Project of China (No. 2011ZX09102-003-03).
Supplementary Material
Download PDF (75.3 KB)References
- Strine TW, Kobau R, Chapman DP, et al. Psychological distress, comorbidities, and health behaviors among U.S. adults with seizures: results from the 2002 National Health Interview Survey. Epilepsia 2005;46:1133–9
- Mc Namara OJ, Brunton LL, Lazo JS, Parker KL (Eds.). The pharmacological basis of therapeutics. New York: McGraw-Hill; 2006:501–26
- Sirven JI, Noe K, Hoerth M, Drazkowski J. Antiepileptic drugs 2012: recent advances and trends. Mayo Clin Proc 2012;87:879–89
- Donner EJ, Snead OC. New generation anticonvulsants for the treatment of epilepsy in children. NeuroRX 2006;3:170–80
- Bootsma HP, Ricker L, Hekster YA, et al. The impact of side effects on long-term retention in three new antiepileptic drugs. Seizure 2009;18:327–31
- Kennedy GM, Lhatoo SD. CNS adverse events associated with antiepileptic drugs. CNS Drugs 2008;22:739–60
- Penovich PE, James Willmore L. Use of a new antiepileptic drug or an old one as first drug for treatment of absence epilepsy. Epilepsia 2009;50:37–41
- Bialer M, Johannessen SI, Kupferberg HJ, et al. Progress report on new antiepileptic drugs: a summary of the Eigth Eilat conference (EILAT VIII). Epilepsy Res 2007;73:1–52
- Bialer M, Yagen B. Valproic acid -- second generation. Neurotherapeutics 2007;4:130–7
- Alam O, Mullick P, Verma SP, et al. Synthesis, anticonvulsant and toxicity screening of newer pyrimidine semicarbazone derivatives. Eur J Med Chem 2010;45:2467–72
- Karakurt A, Ozalp M, Isik S, et al. Synthesis, anticonvulsant and antimicrobial activities of some new 2-acetylnaphthalene derivatives. Bioorg Med Chem 2010;18:2902–11
- Tasso SM. Pharmacophore model for antiepileptic drugs acting on sodium channels. J Mol Model 2001;7:231–9
- Unverferth K, Engel J, Hofgen N, et al. Synthesis, anticonvulsant activity, and structureeactivity relationships of sodium channel blocking 3-aminopyrroles. J Med Chem 1998;41:63–73
- Bruno-Blanch L, Galvez J, Garcia-Domenach R. Topological virtual screening: a way to find new anticonvulsant drugs from chemical diversity. Bioorg Med Chem Lett 2003;13:2749–54
- Estrada E, Pena A. In silico studies for the rational discovery of anticonvulsant compounds. Bioorg Med Chem 2000;8:2755–70
- Stefan H, Feuerstein TJ. Novel anticonvulsant drugs. Pharmacol Ther 2007;113:165–83
- Eswaran S, Adhikari AV, Shetty NS. Synthesis and antimicrobial activities of novel quinoline derivatives carrying 1,2,4-triazole moiety. Eur J Med Chem 2009;44:4637–47
- Bayrak H, Demirbas A, Demirbas N, Karaoglu SA. Synthesis of some new 1,2,4-triazoles starting from isonicotinic acid hydrazide and evaluation of their antimicrobial activities. Eur J Med Chem 2009;44:4362–6
- Patel NB, Khan IH, Rajani SD. Antimycobacterial and antimicrobial study of new 1,2,4-triazoles with benzothiazoles. Arch Pharm (Weinheim) 2010;343:692–9
- Bahadur S, Singh SP, Shukla MK. Synthesis of some new thiosemicarbazides, thiadiazoles, triazoles and their derivatives as potential antiviral agents. Arch Pharm (Weinheim) 1982;315:312–7
- Al-Soud YA, Al-Masoudi NA, Loddo R, Colla PL. In-vitro anti-HIV and antitumor activity of new 3,6-disubstituted [1,2,4]triazolo[3,4-b][1,3,4]thiadiazoles and thiadiazine analogues. Arch Pharm (Weinheim) 2008;341:365–9
- Al-Soud YA. Synthesis and anti-HIV activity of substituted 1,2,4-triazolo-thiophene derivatives. Heteroatom Chem 2007;18:443–8
- Mathew V, Giles D, Keshavayya J, Vaidya VP. Studies on synthesis and pharmacological activities of 1,2,4-triazolo[3,4-b]1,3,4-thiadiazoles and their dihydro analogues. Arch Pharm (Weinheim) 2009;342:210–22
- Deng XQ, Dong ZQ, Song MX, et al. Synthesis and anticonvulsant activities of some triazolothiadiazole derivatives. Arch Pharm (Weinheim) 2012;345:565–73
- Xie ZF, Chai KY, Piao HR, et al. Synthesis and anticonvulsant activity of 7-alkoxyl-4,5-dihydro-[1,2,4]triazolo[4,3-a]quinolines. Bioorg Med Chem Lett 2005;15:4803–5
- Guo LJ, Wei CX, Jia JH, et al. Design and synthesis of 5-alkoxy-[1,2,4]triazolo[4,3-a]quinoline derivatives with anticonvulsant activity. Eur J Med Chem 2009;44:954–8
- Zhang L, Guan LP, Sun XY, et al. Synthesis and anticonvulsant activity of 6-alkoxy-[1,2,4]triazolo[3,4-a]phthalazines. Chem Biol Drug Des 2009;73:313–19
- Deng XQ, Wei CX, Li FN, et al. Design and synthesis of 10-alkoxy-5,6-dihydro-triazolo[4,3-d]benzo[f][1,4]oxazepine derivatives with anticonvulsant activity. Eur J Med Chem 2010;45:3080–6
- Zhang LQ, Guan LP, Wei CX, et al. Synthesis and anticonvulsant activity of some 7-alkoxy-2H-1,4-benzothiazin-3(4H)-ones and 7-alkoxy-4H-[1,2,4]triazolo[4,3-d]benzo[b][1,4]thiazines. Chem Pharm Bull 2010;58:326–31
- Deng XQ, Song MX, Wei CX, et al. Synthesis and anticonvulsant activity of 7-alkoxy-triazolo-[3,4-b]benzo[d]thiazoles. Med Chem 2010;6:313–20
- Krall RJ, Penry JK, White BG, Kupferberg HJ. Antiepileptic drug development: II. Anticonvulsant drug screening. Epilepsia 1978;19:409–28
- Poter RJ, Cereghino JJ, Gladding GD, et al. Antiepileptic drug development program. Cleve Clin Q 1984;51:293–305
- Arnoldi A, Bonsignori A, Melloni P, et al. Synthesis and anticonvulsant and sedative-hypnotic activity of 4-(alkylimino)-2,3-dihydro-4H-1-benzopyrans and -benzothiopyrans. J Med Chem 1990;33:2865–9
- Löscher W, Schmidt D. Which animal models should be used in the search for new antiepileptic drugs? A proposal based on experimental and clinical considerations. Epilepsy Res 1988;2:145–81
- Lipinski CA, Lombardo L, Dominy BW, Feeney PJ. Experimental and computational approaches to estimate solubility and permeability in drug discovery and development settings. Adv Drug Deliv Rev 2001;46:3–26
- Molinspiration Cheminformatics. Available from: http://www.molinspiration.com/ [last accessed 6 June 2012]
- Zhao Y, Abraham MH, Lee J, et al. Rate-limited steps of human oral absorption and QSAR studies. Pharm Res 2002;19:1446–57
- Osiris property calculator. Available from: http://www.organic-chemistry.org/prog/peo [last accessed 8 June 2012]
- Rutkauskas K, Kantminiene K, Beresnevieius ZJ. Syn the sis and cyclization of N-{2-[(2-carboxyethyl)sulfanylphenyl]}-b-alanines. Polish J Chem 2008;82:2341–7
- White HS. Preclinical development of antiepileptic drugs: past, present, and future directions. Epilepsia 2003;44:2–8
- Levy RH, Mattson RH, Meldrum BS (Eds.). Antiepileptic drugs. New York: Raven Press; 1995:99–110
- Okada R, Negishi N, Nagaya H. The role of the nigrotegmental GABAergic pathway in the propagation of pentylenetetrazol-induced seizures. Brain Res 1989;480:383–7
- Olsen RW. GABA-benzodiazepine-barbiturate receptor interactions. J. Neurochem 1981;37:1–13
- Gale K. GABA and epilepsy: basic concepts from preclinical research. Epilepsia 1992;33:S3–12
- Loscher W. 3-Mercaptopropionic acid: convulsant properties, effects on enzymes of the gamma-aminobutyrate system in mouse brain and antagonism by certain anticonvulsant drugs, aminooxyacetic acid and gabaculine. Biochem Pharmacol 1979;28:1397–407
- Macdonald RL, Barker JL. Specific antagonism of GABA-mediated postsynaptic inhibition in cultured mammalian spinal cord neurons: a common mode of convulsant action. Neurology 1978;28:325–30
- Ertl P, Rohde B, Selzer P. Fast calculation of molecular polar surface area as a sum of fragment based contributions and its application to the prediction of drug transport properties. J Med Chem 2000;43:3714–17
- Palm K, Luthman K, Ungell AL, et al. Correlation of drug absorption with molecular surface properties. J Pharm Sci 1996;85:32–9
- Schneeberger EE, Lynch RD. Structure, function and regulation of cellular tight junctions. Am J Physiol 1992;262:647–61
- Kaliszan R, Markuszewski M. Brain/blood distribution described by a combination of partition coefficient and molecular mass. Int J Pharm 1996;145:9–16
- Lien EJ, Liao RC, Shinouda HG. Quantitative structure-activity relationships and dipole moments of anticonvulsants and CNS depressants. J Pharm Sci 1979;68:463–5