Abstract
Objective. Preterm parturition is a syndrome caused by multiple etiologies. Although intra-amniotic infection is causally linked with intrauterine inflammation and the onset of preterm labor, other patients have preterm labor in the absence of demonstrable infection. It is now clear that inflammation may be elicited by activation of the Damage-Associated Molecular Patterns (DAMPs), which include pathogen-associated molecular patterns (PAMPs) as well as “alarmins” (endogenous molecules that signal tissue and cellular damage). A prototypic alarmin is high-mobility group box 1 (HMGB1) protein, capable of inducing inflammation and tissue repair when it reaches the extracellular environment. HMGB1 is a late mediator of sepsis, and blockade of HMGB1 activity reduces mortality in an animal model of endotoxemia, even if administered late during the course of the disorder. The objectives of this study were to: (1) determine whether intra-amniotic infection/inflammation (IAI) is associated with changes in amniotic fluid concentrations of HMGB1; and (2) localize immunoreactivity of HMGB1 in the fetal membranes and umbilical cord of patients with chorioamnionitis. Methods. Amniotic fluid samples were collected from the following groups: (1) preterm labor with intact membranes (PTL) with (n = 42) and without IAI (n = 84); and (2) preterm prelabor rupture of membranes (PROM) with (n = 38) and without IAI (n = 35). IAI was defined as either a positive amniotic fluid culture or amniotic fluid concentration of interleukin-6 (IL-6) ≥2.6 ng/mL. HMGB1 concentrations in amniotic fluid were determined by ELISA. Immunofluorescence staining for HMGB1 was performed in the fetal membranes and umbilical cord of pregnancies with acute chorioamnionitis. Results. (1) Amniotic fluid HMGB1 concentrations were higher in patients with IAI than in those without IAI in both the PTL and preterm PROM groups (PTL IAI: median 3.1 ng/mL vs. without IAI; median 0.98 ng/mL; p < 0.001; and preterm PROM with IAI median 7.3 ng/mL vs. without IAI median 2.6 ng/mL; p = 0.002); (2) patients with preterm PROM without IAI had a higher median amniotic fluid HMGB1 concentration than those with PTL and intact membranes without IAI (p < 0.001); and (3) HMGB1 was immunolocalized to amnion epithelial cells and stromal cells in the Wharton’s jelly (prominent in the nuclei and cytoplasm). Myofibroblasts and macrophages of the chorioamniotic connective tissue layer and infiltrating neutrophils showed diffuse cytoplasmic HMGB1 immunoreactivity. Conclusions. (1) intra-amniotic infection/inflammation is associated with elevated amniotic fluid HMGB1 concentrations regardless of membrane status; (2) preterm PROM was associated with a higher amniotic fluid HMGB1 concentration than PTL with intact membranes, suggesting that rupture of membranes is associated with an elevation of alarmins; (3) immunoreactive HMGB1 was localized to amnion epithelial cells, Wharton’s jelly and cells involved in the innate immune response; and (4) we propose that HMGB1 released from stress or injured cells into amniotic fluid may be responsible, in part, for intra-amniotic inflammation due to non-microbial insults.
Introduction
Preterm parturition is a syndrome [Citation1–3] clinically characterized by activation of the uterine components of the common pathway of parturition, including myometrial contractility [Citation4–13], cervical ripening [Citation14–19] and/or decidual membrane activation [Citation20–22] due to multiple etiologies [Citation1–3,Citation23–33]. Of the mechanisms of disease associated with the preterm parturition syndrome, only intra-amniotic infection/inflammation has been causally linked to preterm delivery [Citation34–40]. Accumulating evidence indicates that not all patients with intra-amniotic inflammation have demonstrable infection using cultivation and molecular techniques [Citation40–52]. This may be attributed either to infections which cannot be detected with molecular microbiologic techniques (cultivation or molecular), because they are caused by microorganisms that are non-culturable (bacteria [Citation41,Citation42], viruses [Citation53–57], etc.) or by microorganisms that have eluded detection with the currently available molecular microbiologic techniques. Another possibility to consider is that inflammation is of non-infection-related etiology. For example, we have proposed that “danger signals” can lead to the initiation of intra-amniotic inflammation and labor in the absence of infection. Recently, we have generated experimental evidence that allergy or hypersensitivity type I can cause preterm labor in animals [Citation58,Citation59], and case reports suggest that this may be the case in humans [Citation26,Citation60–62]. Similarly, we have recently demonstrated that “maternal anti-fetal rejection” is an important and frequent mechanism of disease in preterm labor [Citation29]. Such mechanism of disease is expressed by a unique pattern of chronic inflammation in the chorioamniotic membranes (chronic chorioamnionitis [Citation63,Citation64]) or the placental mass (villitis of unknown etiology [Citation65,Citation66]).
Inflammation is an ancient mechanism of host defense to control endogenous or exogenous damage and restore homeostasis in response to bacteria or tissue injury [Citation67]. To initiate an appropriate inflammatory response, organisms develop several means of cellular communication to sense “danger signals [Citation68]”. Exogenous signals are specific molecular patterns of pathogens including peptidoglycans, lipopolysaccharides, bacterial DNA, viral RNA, etc. and are collectively termed “pathogen-associated molecular patterns (PAMPs [Citation69–71])”. In contrast, endogenous danger signals are normal constituents of cells, which, when the cells sustain damage, leak outside the cells and are capable of eliciting an inflammatory response. Joost Oppenheim proposed the term “alarmins” to differentiate the endogenous molecules that signal tissue and cellular damage from PAMPs [Citation72–74]. Examples of alarmins include heat shock proteins [Citation75,Citation76], S100 proteins [Citation77,Citation78], uric acid [Citation79], hepatoma-derived growth factor [Citation80], interleukin-1α [Citation81], adenosine triphosphate [Citation74] and high-mobility group box-1 (HMGB1 [Citation72]).
HMGB1 has been considered a prototypic alarmin [Citation72]. The characteristics of alarmins are: (1) molecules are rapidly released after non-program cell death (i.e., necrosis), and also, by apoptotic cells; (2) immunocytes can produce and release alarmin without dying, using a specialized mechanism for secretion or the endoplasmic reticulum-Golgi secretion pathway; (Citation3) they can recruit and activate receptor-expressing cells of the innate immune system, including dendritic cells, and therefore, can activate the adaptive immune system; and (Citation4) these molecules should restore homeostasis by promoting healing of the tissue that was destroyed, either because of the direct insult or the secondary effects of the inflammatory process [Citation72].
Originally discovered as a nuclear protein [Citation82,Citation83], HMGB1 is the most widely studied member of the alarmin family. This protein can be released either passively by necrotic cells [Citation84,Citation85] or actively by stressed cells in response to injury [Citation86,Citation87]; it is capable of inducing inflammation [Citation88–97] and tissue repair [Citation98–105] when it reaches the extracellular environment. HMGB1 has been implicated as a late mediator of sepsis [Citation106–109]. Furthermore, blockade of HMGB1 activity reduces mortality in animal models of endotoxemia [Citation107] and sepsis [Citation108,Citation109], even if administered after the onset of the disease. These findings have generated considerable interest in HMGB1 as a potential therapeutic target for several infectious and inflammatory disorders [Citation106,Citation110].
HMGB1 mediates its biological activities through multiple receptors including toll-like receptors (TLRs [Citation89,Citation93,Citation94,Citation111]) and receptors for advanced glycation end products (RAGE [Citation112–117]). Binding of HMGB1 to RAGE can induce the production of pro-inflammatory cytokines and chemokines, as well as neutrophil chemotaxis, in a manner that may be suppressed or stimulated by soluble, truncated forms of RAGE including the soluble form of RAGE (sRAGE) and endogenous secretory RAGE (esRAGE [Citation112]). We have examined the changes of amniotic fluid concentrations of pro-inflammatory cytokines [Citation118–126], anti-inflammatory cytokines [Citation127], chemokines [Citation128–134], proteases/anti-proteases [Citation135], matrix-metalloproteinase [Citation136–143], pro- and anti-angiogenic factors [Citation144–146], coagulation factors [Citation147,Citation148], adipocytokines [Citation149–151], anti-microbial peptides [Citation152] and prostaglandins [Citation153–156] in patients with intra-amniotic infection and/or inflammation (IAI) both at term and preterm gestations. Amniotic fluid concentrations of sRAGE and esRAGE have been reported to be elevated in patients with IAI in preterm gestations and decreased in labor at term [Citation157], whereas the amniotic fluid concentration of HMGB1 in patients with IAI has not yet been examined.
The objectives of this study were to: (1) determine whether IAI in preterm gestation is associated with changes in the amniotic fluid concentration of HMGB1; and (2) immunolocalize HMGB1 in the fetal membranes and umbilical cord of patients with histologic chorioamnionitis.
Materials and methods
Study design and population
A retrospective cross-sectional study was conducted by searching our clinical database and bank of biological samples. Patients with spontaneous preterm labor (PTL) with intact membranes and those with preterm prelabor rupture of membranes (PROM) who had amniotic fluid samples retrieved by trans-abdominal amniocentesis were included. Patients were subdivided into the following groups: (1) PTL and intact membranes with (n = 42) and without IAI (n = 84); and (2) preterm PROM with (n = 38) and without IAI (n = 35).
All women provided written, informed consent before the collection of amniotic fluid samples. The collection and utilization of the samples were approved by the Human Investigation Committee of the participating institutions and the IRB of the Eunice Kennedy Shriver National Institute of Child Health and Human Development (NICHD/NIH/DHHS). Many of these samples have been used in previous studies of the biology of sRAGE, esRAGE and other cytokines and inflammatory mediators in IAI, pregnancy, preterm labor and preterm PROM.
Clinical definition:
The diagnosis of preterm labor was made in the presence of regular uterine contractions (at least 3 in 30 min) and documented cervical change in patients with a gestational age of 20 to 36 6/7 weeks. Preterm PROM was diagnosed with a sterile speculum examination with documentation of vaginal pooling and positive nitrazine and ferning tests. Intra-amniotic infection was defined as a positive culture for bacteria in amniotic fluid, and intra-amniotic inflammation as an amniotic fluid interleukin (IL)-6 concentration of 2.6 ng/mL or more [Citation43]. Intra-amniotic inflammation could be present in the absence of a positive amniotic fluid culture for microorganisms.
Sample collection
Amniotic fluid samples were obtained by trans-abdominal amniocentesis performed for evaluation of the microbial status of the amniotic cavity and/or assessment of fetal lung maturity. Samples of amniotic fluid were transported to the laboratory in a sterile capped syringe and cultured for aerobic/anaerobic bacteria and genital mycoplasmas. White blood cell (WBC) count [Citation158], glucose concentration [Citation159] and Gram stain [Citation160] were also performed shortly after collection as previously described [Citation158,Citation159]. The results of these tests were used for clinical management. Results of amniotic fluid IL-6 concentration were used only for research purposes. Amniotic fluid not required for clinical assessment was centrifuged at 1300g for 10 min at 4°C and the supernatant was stored at −70°C.
Determination of HMGB1 in amniotic fluid
Concentrations of HMGB1 and IL-6 in amniotic fluid were determined by sensitive and specific enzyme immunoassays obtained from IBL International (Toronto, Canada) and from R&D Systems (Minneapolis, MN), respectively. The initial assay validation was performed in our laboratory prior to the conduction of this study. Briefly, the immunoassay utilized the quantitative sandwich enzyme immunoassay technique and the concentrations were determined by interpolation from the standard curves. The inter- and intra-assay coefficients of variation for HMGB1 were 3.1 and 4.4%, respectively, and for IL-6, 8.7 and 4.6%, respectively. The sensitivity of the assay for HMGB1 was 0.2 ng/mL and for IL-6, 0.09 pg/mL. The results of amniotic fluid sRAGE and esRAGE concentrations in several samples have been previously reported [Citation157] but were included in this manuscript in order to provide a comprehensive picture of the relationship between HMGB1 concentrations and that of its soluble receptors.
Immunofluorescence staining of HMGB1 in the fetal membranes and umbilical cord
Immunofluorescence staining was performed to determine whether HMGB1 protein expression could be detected and the localization of the protein in the chorioamniotic membranes and umbilical cord. The placentas of women at term not in labor, at term in labor, and patients who delivered preterm with histologic chorioamnionitis were studied (n = 6). The staining was performed using a mouse monoclonal anti-HMGB1 antibody (1:50; AbCam plc, Cambridge, UK). Five-micrometer-thick sections of formalin-fixed, paraffin-embedded tissues were placed on silanized slides and stained using a Ventana Discovery automatic staining system (Ventana Medical Systems, Tucson, AZ). Sections were incubated with primary antibodies in 1% (w/v) BSA in PBS for 1 h, followed by incubation with Alexa 488 goat anti-mouse IgG and Alexa 594 donkey anti-rabbit IgG (Invitrogen, Carlsbad, CA) in 1% (w/v) BSA for 30 min. Sections were mounted in ProLong® Gold antifade reagent with 4′-6-Diamidino-2-phenylindole (DAPI) (Invitrogen).
Statistical analysis
The Kolmogorov-Smirnov test and Shapiro-Wilk test were used to determine whether the data were normally distributed. A two-tailed Mann–Whitney U test was used to compare continuous non-parametric variables. Comparisons between proportions were performed using Chi-square or Fisher’s exact tests. Spearman rank correlation was utilized to assess correlations between two continuous variables. A p-value < 0.05 was considered statistically significant. Analysis was performed with SPSS, version 15 (SPSS Inc., Chicago, IL).
Results
Demographics and clinical characteristics of the study population
Demographics and clinical characteristics of patients with spontaneous PTL with intact membranes and those with preterm PROM are displayed in and , respectively. There was no significant difference in the median gestational age at amniocentesis between patients with and without IAI (p = 0.3 and p = 0.6, respectively). Immunoreactive HMGB1 in amniotic fluid was detected in 91% (186/205) of the samples. Nineteen samples had HMGB1 concentrations below the detection limit of the immunoassay. Those samples were from patients in the following groups: sixteen from the PTL with intact membranes group (12 without IAI, 4 with IAI), and 3 from the preterm PROM group (1 without IAI, 2 with IAI).
Table I. Demographics and clinical characteristics of patients with preterm labor with intact membranes (PTL).
Table II. Demographics and clinical characteristics of patients with preterm prelabor rupture of membranes (PROM).
Intra-amniotic infection/inflammation was associated with an elevation of amniotic fluid HMGB1 concentration
Patients with PTL and intact membranes with IAI had a significantly higher median amniotic fluid concentration of HMGB1 than those without IAI (PTL with IAI: median 3.1 ng/mL; range: 0–31.2 ng/mL vs. PTL without IAI: median 0.98 ng/mL; range: 0–7.3 ng/mL; p < 0.001; ). Similarly, the median amniotic fluid concentration of HMGB1 in patients with preterm PROM with IAI was significantly higher than those without IAI (preterm PROM with IAI: median 7.3 ng/mL; range: 0–65.4 ng/mL vs. preterm PROM without IAI: median 2.6 ng/mL; range: 0–9.7 ng/mL; p = 0.002; ). As previously reported, IAI was associated with a higher median amniotic fluid concentration of sRAGE and esRAGE in both patients with PTL and those with preterm PROM ( and ).
Figure 1. Amniotic fluid concentrations of HMGB1 in patients with preterm labor and intact membranes (PTL) with and without intra-amniotic infection/inflammation (IAI). Patients with PTL and intact membranes with IAI had a significantly higher median amniotic fluid concentration of HMGB1 than those without IAI (PTL with IAI: median 3.1 ng/mL; range: 0–31.2 ng/mL vs. PTL without IAI: median 0.98 ng/mL; range: 0–7.3 ng/mL; p < 0.001).
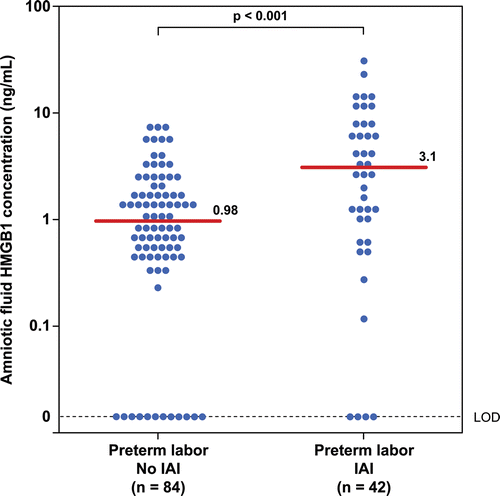
Figure 2. Amniotic fluid concentrations of HMGB1 in patients with preterm prelabor rupture of membranes (PROM) with and without intra-amniotic infection/inflammation (IAI). The median amniotic fluid concentration of HMGB1 in patients with preterm PROM with IAI was significantly higher than those without IAI (preterm PROM with IAI: median 7.3 ng/mL; range: 0–65.4 ng/mL vs. preterm PROM without IAI: median 2.6 ng/mL; range: 0–9.7 ng/mL; p = 0.002).
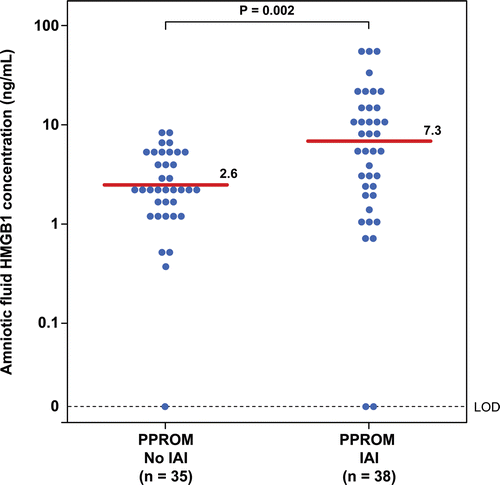
There was no significant difference in the median amniotic fluid concentration of HMGB1 in patients with PTL without IAI between those who delivered at term and preterm (term delivery, n = 56, median 1.2 ng/mL; range: 0–6.7 ng/mL vs. preterm delivery, n = 28, median 0.7 ng/mL; range: 0–7.3 ng/mL; p = 0.2). Among patients with PTL without IAI who delivered at term, there was a significant relationship between amniotic fluid concentrations of HMGB1 and IL-6 (Spearman Rho 0.4; p = 0.007). In contrast, among patients with PTL with IAI, there was no significant relationship between amniotic fluid concentrations of HMGB1 and markers of inflammation (IL-6, WBC, glucose) or its soluble receptors (sRAGE and esRAGE) (each p > 0.05).
There were 28 patients with PTL who delivered preterm without IAI, six of which delivered within 3 days after amniocentesis. The median amniotic fluid HMGB1 concentrations of patients without IAI who delivered within 3 days were not significantly different from patients with PTL who delivered at term without IAI (median 1.3 ng/mL range 0.8–3.1 ng/mL vs. median 1.2 ng/mL range 0–6.7 ng/mL; p = 0.6).
Amniotic fluid HMGB1 concentrations changed with membrane status
Patients with preterm PROM without IAI had a higher median amniotic fluid HMGB1 concentration than patients with PTL and intact membranes without IAI (p < 0.001; ). Although the median gestational age at the time of amniocentesis was slightly lower in patients with preterm PROM without IAI than those with PTL and intact membranes without IAI (preterm PROM without IAI: median 29.6 weeks, range: 23–34.3 weeks vs. PTL without IAI: median 31.3 weeks, range: 23.1–34.5 weeks; p = 0.003), amniotic fluid concentration of HMGB1 did not change as a function of gestational age in patients with PTL without IAI who delivered at term (Spearman Rho = −0.1; p = 0.3).
Figure 3. Amniotic fluid concentrations of HMGB1 in patients with preterm labor and intact membranes (PTL) and preterm prelabor rupture of membranes (PROM) without intra-amniotic infection/inflammation (IAI). Patients with preterm PROM without IAI had a higher median amniotic fluid HMGB1 concentration than patients with PTL and intact membranes without IAI (preterm PROM without IAI: median 2.6 ng/mL; range: 0–9.7 ng/mL vs. PTL without IAI: median 0.98 ng/mL; range: 0–7.3 ng/mL; p < 0.001).
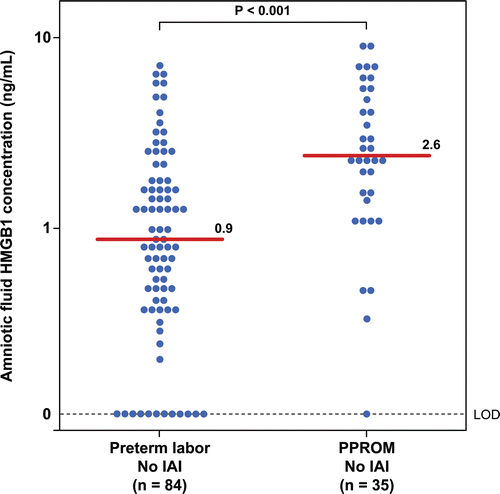
Among all patients with intra-amniotic infection/inflammation (n = 80), those who had a positive culture for microorganisms (n = 43) had a significantly higher (6-fold) median amniotic IL-6 concentration than those with sterile amniotic fluid (n = 37) (median 49 ng/mL range 0.03–388 ng/mL vs. median 7.8 ng/mL range 2.6–923; p < 0.001), whereas there was no significant difference in HMGB1 concentration between those with and without a positive amniotic fluid culture (median 5.8 ng/mL range 0–65 ng/mL vs. median 3.1 range (0–31.2; p = 0.09).
Three patients had intra-amniotic infection without a high amniotic fluid IL-6 concentration (<2.6 ng/mL). The first patient was diagnosed with PTL and underwent amniocentesis at 33.5 weeks. She delivered spontaneously 4 days later. This patient had a positive amniotic fluid culture for Mycoplasma hominis with an IL-6 concentration of 0.64 ng/mL and HMGB1 of 2.6 ng/mL. The other two patients presented with preterm PROM and underwent amniocentesis at 28.9 and 34.4 weeks, respectively. In both cases, amniotic fluid culture revealed Ureaplasma urealyticum. These patients had IL-6 concentrations of 0.02 and 0.53 ng/mL with HMGB1 concentrations of 10.9 and 3.3 ng/mL, respectively.
Immunofluorescence staining for HMGB1 in the fetal membranes and umbilical cord
In the chorioamniotic membranes, immunoreactivity of HMGB1 was prominent in the nuclei or cytoplasm of amniotic epithelial cells. Myofibroblasts and macrophages of the chorioamniotic connective tissue layer and infiltrating neutrophils showed diffuse cytoplasmic HMGB1 immunoreactivity (). In the umbilical cord, stromal cells in the Wharton’s jelly were strongly immunoreactive for HMGB1, both in the nuclei and cytoplasm (. In contrast, immunostaining of HMGB1 on umbilical vascular endothelial cells was negative (.
Figure 4. Immunofluorescence images of HMGB1 immunoreactivity in the chorioamniotic membranes. (a) Chorioamniotic membranes obtained from an uncomplicated pregnant woman who delivered at term. HMGB1 immunoreactivity (green) is evident in the amnion epithelial cells (arrows) and mesenchymal cells of the chorioamniotic connective tissue (arrowheads). ×400. (b) Chorioamniotic membranes obtained from a different case of normal term delivery. Scattered HMGB immunoreactivity (green) is observed in the chorionic trophoblasts (arrows) and decidual stromal cells (arrowheads). ×400. (c) Chorioamniotic membranes obtained from a patient who delivered preterm with acute chorioamnionitis and funisitis. Distinct immunoreactivity (green) is detected in the amnion epithelial cells (arrows), myofibroblasts of chorioamniotic connective tissue (arrowheads), and infiltrating neutrophils (circle). ×400. DAPI (blue) was used to stain nuclei.
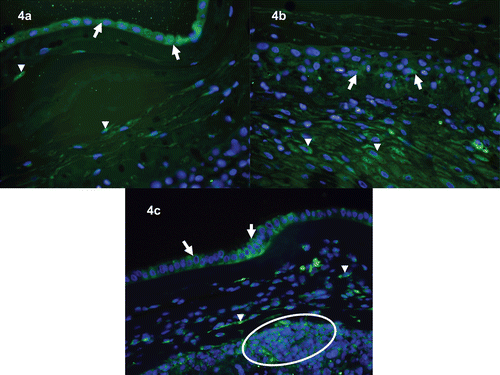
Figure 5. Immunofluorescence images of HMGB1 immunoreactivity in the umbilical cord Wharton’s jelly and umbilical vein. (a) Umbilical cord Wharton’s jelly obtained from a patient who delivered preterm with acute chorioamnionitis and funisitis. Distinct immunoreactivity of HMGB1 (green) is detected in the stromal cells of the Wharton’s jelly. ×400. (b) Umbilical vein obtained from an uncomplicated pregnant woman who delivered at term. HMGB immunoreactivity (green) is absent in umbilical vein endothelial cells (arrows). Autofluorescence from RBCs are prominent (circle). ×400. DAPI (blue) was used to stain nuclei.
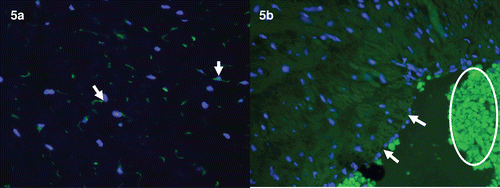
Discussion
Principal findings of the study
(1) Amniotic fluid concentrations of HMGB1 were higher in patients with intra-amniotic infection/inflammation regardless of their membrane status (intact or ruptured); (2) in the absence of intra-amniotic inflammation, patients with preterm PROM had higher amniotic fluid concentrations of HMGB1 than patients with preterm labor with intact membranes; and (3) HMGB1 protein was immunolocalized to amnion epithelial cells, myofibroblasts and infiltrating macrophages of chorioamniotic membranes as well as stromal cells in Wharton’s jelly of the umbilical cord.
Biology of HMGB1
HMGB1 is an evolutionarily conserved DNA-binding protein that stabilizes nucleosomes and facilitates gene transcription [Citation88]. This protein is expressed in almost every cell type (except those without a nucleus such as erythrocytes and skin epithelial cells [Citation161]) and can be actively released from cells in response to bacterial products (e.g., peptidoglycan, lipopolysaccharide (LPS), CpG-DNA [Citation162–165]), cytokines [e.g., tumor necrosis factor (TNF)-α, interferon-γ (iFN-γ) and transforming growth factor-β [Citation165–167]]or tissue injury (i.e. ischemic/reperfusion, nerve crush injury [Citation86,Citation87,Citation168]). Due to the lack of a leader signal sequence, HMGB1 can not be actively secreted through the classic endoplasmic reticulum-Golgi, but requires a specialized pathway to translocate the protein from the nucleus to the cytoplasm and load it into secretory lysosomes [Citation72,Citation169]. Eventually, this protein can be secreted into the extracellular environment by either exocytosis [Citation169] or released as exosomes [Citation170]. The release of HMGB1 from the nucleus of activated macrophages and dendritic cells is controlled by a crucial acetylation step. Alternatively, HMGB1 can be passively released by necrotic cells [Citation84,Citation85] or cells infected with viruses (e.g., West Nile, influenza, etc. [Citation171,Citation172]) or mycobacteria [Citation173,Citation174]. It is noteworthy that, in granulocytes, HMGB1 is tightly sequestered in a detergent-insoluble form, which limits its release even under conditions of necrotic cell death [Citation84,Citation161].
Several types of receptors have been implicated in HMGB1 signaling, including RAGE [Citation112–117] and members of the toll-like receptors (TLR-2, -4, and -9 [Citation89,Citation93,Citation94,Citation111]). Upon binding to its receptors, HMGB1 activates immune cells or endothelial cells to produce pro-inflammatory cytokines (TNF-α, IL-6 and IFN-γ), chemokines and adhesion molecules [Citation88–97] as well as maturation and migration of immune cells [Citation92,Citation97,Citation114,Citation115]. Moreover, HMGB1 can stimulate the migration of stem cells, which is important for tissue repair and regeneration after inflammation [Citation98–105].
Since HMGB1 can elicit sustained inflammatory response in the extracellular environment, a few physiologic regulators are present to control biological activity of HMGB1. These molecules include thrombomodulin [Citation175–177], CD24 and Siglec-10 [Citation178,Citation179], as well as heat shock protein-72 [Citation180]. Vagal nerve stimulation has also been shown to inhibit TNF-α release and reduce systemic HMGB1 concentrations, as well as improve survival in animal models of sepsis through an α-7 nicotinic acetylycholine receptor [Citation181,Citation182].
Recently, Rouhiainen et al. demonstrated that ultra-purified HMGB1 has weak pro-inflammatory activity in mononuclear cells [Citation183]. Since HMGB1 has a natural propensity to bind negatively charged molecules such as DNA, RNA, nucleosomes, PAMP or immune activating cytokines such as IL-1, it is possible that HMGB1 may form a complex with TLR agonists (i.e. LPS, IL-1) that triggers the respective TLR or cytokine receptors and sends an “alarm signal” [Citation16].
HMGB1, a late mediator of sepsis
HMGB1 was proposed to be a late mediator of sepsis [Citation107]. Wang et al. identified this 30-kDa protein in the conditioned media of macrophage culture after stimulation with endotoxin. In an animal model of endotoxemia [Citation107] and sepsis [Citation184], HMGB1 was first detected in the circulation late during the course of the disorder. This profile of HMGB1 parallels the occurrence of death induced by endotoxemia [Citation107] and sepsis [Citation184], and distinguishes itself from TNF-α and other pro-inflammatory cytokines which are increased early after the administration of endotoxin (IL-1β [Citation108,Citation109]). The administration of HMGB1-neutralizing antibodies conferred a dose-dependent protective effect even when administered 24 h after the onset of sepsis [Citation184]. These observations indicate that HMGB1 is a potential therapeutic target for sepsis and other inflammatory disorders [Citation106,Citation109,Citation161].
Intra-amniotic infection/inflammation was associated with an elevation of HMGB1
The finding that intra-amniotic infection/inflammation in preterm gestations was associated with an approximate 3-fold increase in the amniotic fluid concentration of HMGB1 suggests that this alarmin may participate in the host response to intra-amniotic infection or cellular injury in spontaneous preterm delivery. We have proposed that intra-amniotic inflammation is induced by microorganisms which may be detectable by standard cultivation or molecular techniques [Citation41,Citation43,Citation45]. However, the possibility that inflammation caused by endogenous alarmins released from stressed or injured cells due to non-microbial insults should also be considered. It is now clear that inflammation can be caused by multiple insults unrelated to microorganisms [Citation72,Citation74,Citation185].
Microbial products such as LPS and endogenous mediators (alarmins) such as HMGB1 can stimulate IL-6 and other pro-inflammatory cytokines [Citation186]. Yet, in the current study, there was no relationship between IL-6 and HMGB1 in patients with intra-amniotic inflammation. It is noteworthy that, among patients with intra-amniotic inflammation, the median HMGB1 concentration in amniotic fluid was not significantly different between patients with and without a positive amniotic fluid culture for microorganisms, while the median amniotic fluid IL-6 concentration was 6-fold higher in those with a microbiologically proven infection than in those with sterile amniotic fluid. One interpretation of these findings is that microorganisms and microbial products are stronger inducer of IL-6 than of HMGB1 in cases of intra-amniotic infection. Evidence in support of this interpretation includes: (1) LPS and HMGB1 induce a distinct pattern of cytokine release from neutrophils based on microarray experiments [Citation92,Citation187,Citation188]; and (2) the administration of an HMGB1-neutralizing antibody to mice with LPS-induced lung injury does not significantly reduce the concentration of several pro-inflammatory cytokines (TNF-α, IL-1α, etc. [Citation108]), suggesting that HMGB1 acts at more distal point in the endotoxin-induced pro-inflammatory cascade, which is different from the effects of LPS (specifically, some effects of LPS may be due to early mediators). Alternatively, it can be argued that the magnitude of the HMGB1 elevation in amniotic fluid is similar in patients with intra-amniotic inflammation, regardless of whether the stimuli are microbial.
Of note, we also observed that, under certain circumstances, patients with a microbiologically-proven intra-amniotic infection had an elevation of HMGB1 concentration, despite no elevation of IL-6 (<2.6 ng/mL). The reasons for this dissociation between IL-6 and HMGB1 are not known at this time, but we believe that this is an important observation. It suggests that the action of microorganisms may be mediated through the conventional pathway of chemokines and cytokines, such as IL-1β, TNFα and IL-6 in some cases, but in others, it may be mediated by the action of what we now call alarmins. If this interpretation is correct, there may be an overlap in the mechanisms of host response induced by bacteria. In some cases, the biological effects are mediated to the classical PAMPs, whereas in others, the effect of bacteria on cells may lead to the release of alarmins such as HMGB1. These alarmins could then trigger the inflammatory process. It is understandable why the term “DAMPs [Citation189]” has been somewhat confusing in the literature—some authors use this term to refer to endogenous molecules released in response to cell injury not caused by bacteria, while others use the term DAMPs as an umbrella to include PAMPs as well as endogenous mediators, or alarmins.
Clearly, it is possible that infection can elicit different cytokine responses. For example, the duration of microbial invasion of the amniotic cavity, the gestational age at which infection occurs (very early pregnancy or close to term), the clinical presentation in preterm labor or PROM and the timing of the retrieval of amniotic fluid can all contribute to explaining the differences in the profile of chemokines, cytokines, inflammatory mediators and alarmins at a given point in the natural history of the inflammatory process. Some patients present in preterm labor in the early phase of the inflammatory response in which HMGB1 is low—this is likely to represent the majority of patients (IL-6 will be elevated in such patients). In contrast, in patients whose intra-amniotic infection is characterized by a prolonged exposure to microbial products and microorganisms, the host response may have evolved so that HMGB1 is elevated. This is the equivalent of what happens in some patients with sepsis, in which HMGB1 is typically a late mediator of septic shock and death [Citation108].
Amniotic fluid HMGB1 concentrations did not correlate with sRAGE or esRAGE
RAGE, a member of the immunoglobulin receptor superfamily, is one of the main receptors for HMGB1 [Citation117] and is expressed in several cell types including amnion epithelium, extravillous trophoblasts, decidua, endothelial cells, macrophages, smooth muscle cells and neurons. The soluble truncated form of RAGE has been proposed to act as a regulatory negative feedback mechanism to modulate the activity of RAGE or HMGB1 [Citation112]. However, this soluble form (sRAGE) has also been shown to induce the production of pro-inflammatory cytokines and chemokines by monocytes [Citation190] and spleen cells [Citation191]. Both the soluble and endogenous secretory forms of RAGE (sRAGE and esRAGE) were elevated in patients with IAI at preterm gestations regardless of the membrane status [Citation157]. However, in the current study, neither sRAGE nor esRAGE concentrations correlated with HMGB1. These findings could be explained by the nature of RAGE which can be induced by several pro-inflammatory molecules (other than HMGB1), including S100/calgranulins, amyloid and beta sheet fibrils [Citation112]. Indeed, intra-amniotic infection has been associated with a significant increase in amniotic fluid concentrations of S-100B [Citation78], calprotectin (heterocomplex of S100A8 or calgranulin-A and S100A9 or calgranulin-B [Citation152]).
Amniotic fluid HMGB1 in patients with and without intra-amniotic infection of membranes
In the absence of intra-amniotic infection/inflammation, there was a significant correlation between the amniotic fluid concentrations of HMGB1 and IL-6. In such instances, mild cellular injury or stress may be responsible for the presence of extracellular HMGB1 and IL-6 in the amniotic fluid, and these proteins may be beneficial for tissue repair and resolution of a minor cellular injury (e.g., preterm labor without infection that eventually results in delivery at term because the episode of preterm labor resolves without delivery).
The finding that there was no significant difference in HMGB1 concentrations in the amniotic fluid of patients without intra-amniotic inflammation who delivered preterm compared to those who delivered at term suggests that HMGB1 does not appear to be involved in the mechanism of preterm labor/delivery in the absence of an elevation of IL-6.
An interesting question is whether spontaneous preterm labor can be induced by danger signals, which may elicit an intra-amniotic inflammatory response in the absence of demonstrable microorganisms and their products. This question has not been addressed in this study; however, preliminary observations suggest that there is a subgroup of patients with these characteristics (an elevated IL-6, negative amniotic fluid cultures and PCR for microbial genomes and an elevated HMGB1). We propose that such patients have a different mechanism for inflammation-induced preterm labor. Insults unrelated to demonstrable infection at the time of amniocentesis may elicit damage of host cells leading to an inflammatory response characterized by elevations of both IL-6 and HMGB1. The placental pathology of such cases remains to be established (Romero R, Chaiworapongsa T, unpublished observations, 2011).
HMGB1 may be involved in the mechanisms of rupture of membranes
A major finding of the current study is that patients with preterm PROM had higher concentrations of HMGB1 in amniotic fluid than those with intact membranes. This observation is consistent with the findings from a previous study that HMGB1 is capable of stimulating matrix metalloproteinase (MMP)-9 in neuronal and glial cell cultures [Citation192]. This group of proteins has been implicated in the mechanisms of membrane rupture [Citation120,Citation121,Citation136–143,Citation193,Citation194]. In contrast, our findings for HMGB1 are different than the behavior of a large number of inflammatory mediators including prostaglandins [Citation153–156], cytokines [Citation118–121] and chemokines [Citation128–130] in women with preterm labor and preterm PROM. In the overwhelming majority of cases, the concentrations of inflammatory mediators are higher in patients with preterm labor and intact membranes than in those with preterm PROM. The exception to this rule is the concentration and/or activity of matrix-degrading enzymes, which are implicated in the mechanisms of membrane rupture [Citation120,Citation121,Citation136–143,Citation193,Citation194]. In such cases, the concentrations of MMP1, MMP8 and MMP9 are significantly higher in both the amniotic fluid [Citation136,Citation140,Citation141] and fetal blood [Citation121] in patients with preterm PROM than in patients with preterm labor and intact membranes, regardless of microbial status.
It remains to be determined whether HMGB1 in amniotic fluid can stimulate MMP expression in the fetal membranes or by immune cells. It is also possible that higher concentrations of extracellular HMGB1 in amniotic fluid observed in patients with membrane rupture may reflect more cellular damage in this condition than in patients with preterm labor and intact membranes. Furthermore, a recent study observed a lower HMGB1 mRNA expression in cervical biopsy specimens of patients with preterm PROM compared to those of patients with preterm labor and intact membranes [Citation195].
Immunolocalization of HMGB1 in fetal membranes and umbilical cord
The sources of HMGB1 in the amniotic fluid of patients with intra-amniotic inflammation were unknown. In the current study, however, HMGB1 was immunolocalized to the cytoplasm of amnion epithelium cells, myofibroblasts and macrophages of chorioamniotic membranes, and infiltrating neutrophils in patients with histologic chorioamnionitis, indicating that HMGB1 may be translocated from the nucleus to the cytoplasm in these cells and eventually released into amniotic fluid. These findings are consistent with those reported in normal pregnancy at term, which showed strong nuclear and cytoplasmic expression of HMGB1 in amnion epithelial cells using immunohistochemistry and in situ hybridization [Citation196].
Strengths and limitations of this study
This is the first report of HMGB1 concentration in the amniotic fluid of patients with preterm labor and preterm PROM. It is tempting to propose that this protein may reflect a “danger signal” responsible for the mechanisms of membrane rupture. However, due to the cross-sectional nature of the study, a temporal relationship between an elevation of HMGB1 in amniotic fluid and membrane rupture could not be established.
Conclusion
The observation that amniotic fluid concentrations of HMGB1 were increased in intra-amniotic infection/inflammation suggests that this alarmin participates in the host response to intra-amniotic infection or cellular injury during intra-amniotic inflammation in preterm gestation. The possibility that intra-amniotic inflammation and preterm labor can be caused by endogenous alarmins released from stress or injured cells due to non-microbial insults should be considered.
Acknowledgment
This research was supported, in part, by the Perinatology Research Branch, Division of Intramural Research, Eunice Kennedy Shriver National Institute of Child Health and Human Development, NIH, DHHS.
Declaration of interest: The authors declare no conflicts of interest exist.
References
- Romero R, Mazor M, Munoz H, Gomez R, Galasso M, Sherer DM. The preterm labor syndrome. Ann N Y Acad Sci 1994;734:414–429.
- Romero R, Sepulveda W, Baumann P, Yoon B, Brandt F, Gomez R, Mazor M et al. The preterm labor syndrome: Biochemical, cytologic, immunologic, pathologic, microbiologic and clinical evidence that preterm labor is a heterogenous disease. Am J Obstet Gynecol 1993;168:288
- Romero R, Espinoza J, Kusanovic JP, Gotsch F, Hassan S, Erez O, Chaiworapongsa T, Mazor M. The preterm parturition syndrome. BJOG 2006;113 Suppl 3:17–42.
- Mittal P, Romero R, Tarca AL, Gonzalez J, Draghici S, Xu Y, Dong Z, et al. Characterization of the myometrial transcriptome and biological pathways of spontaneous human labor at term. J Perinat Med 2010;38:617–643.
- Havelock JC, Keller P, Muleba N, Mayhew BA, Casey BM, Rainey WE, Word RA. Human myometrial gene expression before and during parturition. Biol Reprod 2005;72:707–719.
- Garfield RE, Saade G, Buhimschi C, Buhimschi I, Shi L, Shi SQ, Chwalisz K. Control and assessment of the uterus and cervix during pregnancy and labour. Hum Reprod Update 1998;4:673–695.
- Granström L, Ekman G, Ulmsten U, Malmström A. Changes in the connective tissue of corpus and cervix uteri during ripening and labour in term pregnancy. Br J Obstet Gynaecol 1989;96:1198–1202.
- Chwalisz K, Garfield RE. Regulation of the uterus and cervix during pregnancy and labor. Role of progesterone and nitric oxide. Ann N Y Acad Sci 1997;828:238–253.
- Garfield RE, Hayashi RH. Appearance of gap junctions in the myometrium of women during labor. Am J Obstet Gynecol 1981;140:254–260.
- Miller SM, Garfield RE, Daniel EE. Improved propagation in myometrium associated with gap junctions during parturition. Am J Physiol 1989;256:C130–C141.
- Wu WX, Derks JB, Zhang Q, Nathanielsz PW. Changes in heat shock protein-90 and -70 messenger ribonucleic acid in uterine tissues of the ewe in relation to parturition and regulation by estradiol and progesterone. Endocrinology 1996;137:5685–5693.
- Wu WX, Ma XH, Zhang Q, Nathanielsz PW. Characterization of topology-, gestation- and labor-related changes of a cassette of myometrial contraction-associated protein mRNA in the pregnant baboon myometrium. J Endocrinol 2001;171:445–453.
- Bievenue AM, Jenkins SL, Nathanielsz PW. The effects of photoperiod on the switching of myometrial contractility patterns of pregnant baboons: Relationship to surgery and parturition. J Soc Gynecol Investig 2002;9:27–31.
- Hassan SS, Romero R, Tarca AL, Nhan-Chang CL, Vaisbuch E, Erez O, Mittal P, et al. The transcriptome of cervical ripening in human pregnancy before the onset of labor at term: Identification of novel molecular functions involved in this process. J Matern Fetal Neonatal Med 2009;22:1183–1193.
- Timmons BC, Mitchell SM, Gilpin C, Mahendroo MS. Dynamic changes in the cervical epithelial tight junction complex and differentiation occur during cervical ripening and parturition. Endocrinology 2007;148:1278–1287.
- Andersson S, Minjarez D, Yost NP, Word RA. Estrogen and progesterone metabolism in the cervix during pregnancy and parturition. J Clin Endocrinol Metab 2008;93:2366–2374.
- Word RA, Li XH, Hnat M, Carrick K. Dynamics of cervical remodeling during pregnancy and parturition: Mechanisms and current concepts. Semin Reprod Med 2007;25:69–79.
- Maul H, Mackay L, Garfield RE. Cervical ripening: Biochemical, molecular, and clinical considerations. Clin Obstet Gynecol 2006;49:551–563.
- Schlembach D, Mackay L, Shi L, Maner WL, Garfield RE, Maul H. Cervical ripening and insufficiency: From biochemical and molecular studies to in vivo clinical examination. Eur J Obstet Gynecol Reprod Biol 2009;144 Suppl 1:S70–S76.
- Nhan-Chang CL, Romero R, Tarca AL, Mittal P, Kusanovic JP, Erez O, Mazaki-Tovi S, et al. Characterization of the transcriptome of chorioamniotic membranes at the site of rupture in spontaneous labor at term. Am J Obstet Gynecol 2010;202:462.e1–462.41.
- Haddad R, Tromp G, Kuivaniemi H, Chaiworapongsa T, Kim YM, Mazor M, Romero R. Human spontaneous labor without histologic chorioamnionitis is characterized by an acute inflammation gene expression signature. Am J Obstet Gynecol 2006;195:394.e1–394.24.
- Bollapragada S, Bollopragada S, Youssef R, Jordan F, Greer I, Norman J, Nelson S. Term labor is associated with a core inflammatory response in human fetal membranes, myometrium, and cervix. Am J Obstet Gynecol 2009;200:104.e1–104.11.
- Liggins GC. Etiology of premature labor. Mead Johnson Symp Perinat Dev Med 1980;7.
- Iams JD, Romero R, Culhane JF, Goldenberg RL. Primary, secondary, and tertiary interventions to reduce the morbidity and mortality of preterm birth. Lancet 2008;371:164–175.
- Gotsch F, Gotsch F, Romero R, Erez O, Vaisbuch E, Kusanovic JP, Mazaki-Tovi S, et al. The preterm parturition syndrome and its implications for understanding the biology, risk assessment, diagnosis, treatment and prevention of preterm birth. J Matern Fetal Neonatal Med 2009;22 (Suppl. 2):5–23.
- Romero R, Kusanovic JP, Muñoz H, Gomez R, Lamont RF, Yeo L. Allergy-induced preterm labor after the ingestion of shellfish. J Matern Fetal Neonatal Med 2010;23:351–359.
- Romero R, Chaiworapongsa T, Kuivaniemi H, Tromp G. Bacterial vaginosis, the inflammatory response and the risk of preterm birth: A role for genetic epidemiology in the prevention of preterm birth. Am J Obstet Gynecol 2004;190:1509–1519.
- Romero R. Prenatal medicine: The child is the father of the man. 1996. J Matern Fetal Neonatal Med 2009;22:636–639.
- Lee J, Romero R, Xu Y, Kim JS, Topping V, Yoo W, Kusanovic JP, et al. A signature of maternal anti-fetal rejection in spontaneous preterm birth: Chronic chorioamnionitis, anti-human leukocyte antigen antibodies, and C4d. PLoS ONE 2011;6:e16806.
- Arias F, Victoria A, Cho K, Kraus F. Placental histology and clinical characteristics of patients with preterm premature rupture of membranes. Obstet Gynecol 1997;89:265–271.
- Arias F, Rodriquez L, Rayne SC, Kraus FT. Maternal placental vasculopathy and infection: Two distinct subgroups among patients with preterm labor and preterm ruptured membranes. Am J Obstet Gynecol 1993;168:585–591.
- Gomez R, Galasso M, Romero R, Mazor M, Sorokin Y, Gonçalves L, Treadwell M. Ultrasonographic examination of the uterine cervix is better than cervical digital examination as a predictor of the likelihood of premature delivery in patients with preterm labor and intact membranes. Am J Obstet Gynecol 1994;171:956–964.
- Huszar G, Naftolin F. The myometrium and uterine cervix in normal and preterm labor. N Engl J Med 1984;311:571–581.
- Romero R, Mazor M, Wu YK, Sirtori M, Oyarzun E, Mitchell MD, Hobbins JC. Infection in the pathogenesis of preterm labor. Semin Perinatol 1988;12:262–279.
- Romero R, Sirtori M, Oyarzun E, Avila C, Mazor M, Callahan R, Sabo V, et al. Infection and labor. V. Prevalence, microbiology, and clinical significance of intraamniotic infection in women with preterm labor and intact membranes. Am J Obstet Gynecol 1989;161:817–824.
- Romero R, Espinoza J, Gonçalves LF, Kusanovic JP, Friel L, Hassan S. The role of inflammation and infection in preterm birth. Semin Reprod Med 2007;25:21–39.
- Romero R, Mazor M. Infection and preterm labor. Clin Obstet Gynecol 1988;31:553–584.
- Romero R, Avila C, Brekus CA, Morotti R. The role of systemic and intrauterine infection in preterm parturition. Ann N Y Acad Sci 1991;622:355–375.
- Romero R, Espinoza J, Gonçalves LF, Kusanovic JP, Friel LA, Nien JK. Inflammation in preterm and term labour and delivery. Semin Fetal Neonatal Med 2006;11:317–326.
- Gibbs RS, Romero R, Hillier SL, Eschenbach DA, Sweet RL. A review of premature birth and subclinical infection. Am J Obstet Gynecol 1992;166:1515–1528.
- DiGiulio DB, Romero R, Amogan HP, Kusanovic JP, Bik EM, Gotsch F, Kim CJ, et al. Microbial prevalence, diversity and abundance in amniotic fluid during preterm labor: A molecular and culture-based investigation. PLoS ONE 2008;3:e3056.
- DiGiulio DB, Romero R, Kusanovic JP, Gómez R, Kim CJ, Seok KS, Gotsch F, et al. Prevalence and diversity of microbes in the amniotic fluid, the fetal inflammatory response, and pregnancy outcome in women with preterm pre-labor rupture of membranes. Am J Reprod Immunol 2010;64:38–57.
- Yoon BH, Romero R, Moon JB, Shim SS, Kim M, Kim G, Jun JK. Clinical significance of intra-amniotic inflammation in patients with preterm labor and intact membranes. Am J Obstet Gynecol 2001;185:1130–1136.
- Yoon BH, Romero R, Kim M, Kim EC, Kim T, Park JS, Jun JK. Clinical implications of detection of Ureaplasma urealyticum in the amniotic cavity with the polymerase chain reaction. Am J Obstet Gynecol 2000;183:1130–1137.
- Yoon BH, Romero R, Lim JH, Shim SS, Hong JS, Shim JY, Jun JK. The clinical significance of detecting Ureaplasma urealyticum by the polymerase chain reaction in the amniotic fluid of patients with preterm labor. Am J Obstet Gynecol 2003;189:919–924.
- Oh KJ, Lee SE, Jung H, Kim G, Romero R, Yoon BH. Detection of ureaplasmas by the polymerase chain reaction in the amniotic fluid of patients with cervical insufficiency. J Perinat Med 2010;38:261–268.
- Kim M, Kim G, Romero R, Shim SS, Kim EC, Yoon BH. Biovar diversity of Ureaplasma urealyticum in amniotic fluid: Distribution, intrauterine inflammatory response and pregnancy outcomes. J Perinat Med 2003;31:146–152.
- Hitti J, Riley DE, Krohn MA, Hillier SL, Agnew KJ, Krieger JN, Eschenbach DA. Broad-spectrum bacterial rDNA polymerase chain reaction assay for detecting amniotic fluid infection among women in premature labor. Clin Infect Dis 1997;24:1228–1232.
- Gardella C, Riley DE, Hitti J, Agnew K, Krieger JN, Eschenbach D. Identification and sequencing of bacterial rDNAs in culture-negative amniotic fluid from women in premature labor. Am J Perinatol 2004;21:319–323.
- Jalava J, Mäntymaa ML, Ekblad U, Toivanen P, Skurnik M, Lassila O, Alanen A. Bacterial 16S rDNA polymerase chain reaction in the detection of intra-amniotic infection. Br J Obstet Gynaecol 1996;103:664–669.
- Jacobsson B, Aaltonen R, Rantakokko-Jalava K, Morken NH, Alanen A. Quantification of Ureaplasma urealyticum DNA in the amniotic fluid from patients in PTL and pPROM and its relation to inflammatory cytokine levels. Acta Obstet Gynecol Scand 2009;88:63–70.
- Oyarzún E, Yamamoto M, Kato S, Gómez R, Lizama L, Moenne A. Specific detection of 16 micro-organisms in amniotic fluid by polymerase chain reaction and its correlation with preterm delivery occurrence. Am J Obstet Gynecol 1998;179:1115–1119.
- Tsekoura EA, Konstantinidou A, Papadopoulou S, Athanasiou S, Spanakis N, Kafetzis D, Antsaklis A, Tsakris A. Adenovirus genome in the placenta: Association with histological chorioamnionitis and preterm birth. J Med Virol 2010;82:1379–1383.
- Srinivas SK, Ma Y, Sammel MD, Chou D, McGrath C, Parry S, Elovitz MA. Placental inflammation and viral infection are implicated in second trimester pregnancy loss. Am J Obstet Gynecol 2006;195:797–802.
- Baschat AA, Towbin J, Bowles NE, Harman CR, Weiner CP. Is adenovirus a fetal pathogen? Am J Obstet Gynecol 2003;189:758–763.
- Miller JL, Harman C, Weiner C, Baschat AA. Perinatal outcomes after second trimester detection of amniotic fluid viral genome in asymptomatic patients. J Perinat Med 2009;37:140–143.
- Baschat AA, Towbin J, Bowles NE, Harman CR, Weiner CP. Prevalence of viral DNA in amniotic fluid of low-risk pregnancies in the second trimester. J Matern Fetal Neonatal Med 2003;13:381–384.
- Bytautiene E, Romero R, Vedernikov YP, El-Zeky F, Saade GR, Garfield RE. Induction of premature labor and delivery by allergic reaction and prevention by histamine H1 receptor antagonist. Am J Obstet Gynecol 2004;191:1356–1361.
- Bytautiene E, Vedernikov YP, Maner WL, Saade GR, Romero R, Garfield RE. Challenge with ovalbumin antigen increases uterine and cervical contractile activity in sensitized guinea pigs. Am J Obstet Gynecol 2008;199:658.e1–658.e6.
- Klein VR, Harris AP, Abraham RA, Niebyl JR. Fetal distress during a maternal systemic allergic reaction. Obstet Gynecol 1984;64:15S–17S.
- Habek D, Cerkez-Habek J, Jalsovec D. Anaphylactic shock in response to wasp sting in pregnancy. Zentralbl Gynakol 2000;122:393–394.
- Shingai Y, Nakagawa K, Kato T, Fujioka T, Matsumoto T, Kihana T, Noda K, Mori T. Severe allergy in a pregnant woman after vaginal examination with a latex glove. Gynecol Obstet Invest 2002;54:183–184.
- Oggé G, Romero R, Lee DC, Gotsch F, Than NG, Lee J, Chaiworapongsa T, et al. Chronic chorioamnionitis displays distinct alterations of the amniotic fluid proteome. J Pathol 2011;223:553–565.
- Kim CJ, Romero R, Kusanovic JP, Yoo W, Dong Z, Topping V, Gotsch F, et al. The frequency, clinical significance, and pathological features of chronic chorioamnionitis: A lesion associated with spontaneous preterm birth. Mod Pathol 2010;23:1000–1011.
- Kim MJ, Romero R, Kim CJ, Tarca AL, Chhauy S, LaJeunesse C, Lee DC, et al. Villitis of unknown etiology is associated with a distinct pattern of chemokine up-regulation in the feto-maternal and placental compartments: Implications for conjoint maternal allograft rejection and maternal anti-fetal graft-versus-host disease. J Immunol 2009;182:3919–3927.
- Kim JS, Romero R, Kim MR, Kim YM, Friel L, Espinoza J, Kim CJ. Involvement of Hofbauer cells and maternal T cells in villitis of unknown aetiology. Histopathology 2008;52:457–464.
- Medzhitov R. Inflammation 2010: New adventures of an old flame. Cell 2010;140:771–776.
- Matzinger P. The danger model: A renewed sense of self. Science 2002;296:301–305.
- Medzhitov R, Janeway CA Jr..Innate immunity: The virtues of a nonclonal system of recognition. Cell 1997;91:295–298.
- Medzhitov R. Toll-like receptors and innate immunity. Nat Rev Immunol 2001;1:135–145.
- Medzhitov R, Janeway CA Jr. Decoding the patterns of self and nonself by the innate immune system. Science 2002;296:298–300.
- Bianchi ME. DAMPs, PAMPs and alarmins: All we need to know about danger. J Leukoc Biol 2007;81:1–5.
- Harris HE, Raucci A. Alarmin(g) news about danger: Workshop on innate danger signals and HMGB1. EMBO Rep 2006;7:774–778.
- Oppenheim JJ, Tewary P, de la Rosa G, Yang D. Alarmins initiate host defense. Adv Exp Med Biol 2007;601:185–194.
- Schmitt E, Gehrmann M, Brunet M, Multhoff G, Garrido C. Intracellular and extracellular functions of heat shock proteins: Repercussions in cancer therapy. J Leukoc Biol 2007;81:15–27.
- Chaiworapongsa T, Erez O, Kusanovic JP, Vaisbuch E, Mazaki-Tovi S, Gotsch F, Than NG, et al. Amniotic fluid heat shock protein 70 concentration in histologic chorioamnionitis, term and preterm parturition. J Matern Fetal Neonatal Med 2008;21:449–461.
- Bianchi R, Adami C, Giambanco I, Donato R. S100B binding to RAGE in microglia stimulates COX-2 expression. J Leukoc Biol 2007;81:108–118.
- Friel LA, Romero R, Edwin S, Nien JK, Gomez R, Chaiworapongsa T, Kusanovic JP, et al. The calcium binding protein, S100B, is increased in the amniotic fluid of women with intra-amniotic infection/inflammation and preterm labor with intact or ruptured membranes. J Perinat Med 2007;35:385–393.
- Shi Y, Evans JE, Rock KL. Molecular identification of a danger signal that alerts the immune system to dying cells. Nature 2003;425:516–521.
- Zhou Z, Yamamoto Y, Sugai F, Yoshida K, Kishima Y, Sumi H, Nakamura H, Sakoda S. Hepatoma-derived growth factor is a neurotrophic factor harbored in the nucleus. J Biol Chem 2004;279:27320–27326.
- Romero R, Mazor M, Brandt F, Sepulveda W, Avila C, Cotton DB, Dinarello CA. Interleukin-1 α and interleukin-1 β in preterm and term human parturition. Am J Reprod Immunol 1992;27:117–123.
- Goodwin GH, Sanders C, Johns EW. A new group of chromatin-associated proteins with a high content of acidic and basic amino acids. Eur J Biochem 1973;38:14–19.
- Müller S, Scaffidi P, Degryse B, Bonaldi T, Ronfani L, Agresti A, Beltrame M, Bianchi ME. New EMBO members’ review: The double life of HMGB1 chromatin protein: Architectural factor and extracellular signal. EMBO J 2001;20:4337–4340.
- Rovere-Querini P, Capobianco A, Scaffidi P, Valentinis B, Catalanotti F, Giazzon M, Dumitriu IE, et al. HMGB1 is an endogenous immune adjuvant released by necrotic cells. EMBO Rep 2004;5:825–830.
- Raucci A, Palumbo R, Bianchi ME. HMGB1: A signal of necrosis. Autoimmunity 2007;40:285–289.
- Qiu J, Nishimura M, Wang Y, Sims JR, Qiu S, Savitz SI, Salomone S, Moskowitz MA. Early release of HMGB-1 from neurons after the onset of brain ischemia. J Cereb Blood Flow Metab 2008;28:927–938.
- Tsung A, Klune JR, Zhang X, Jeyabalan G, Cao Z, Peng X, Stolz DB, et al. HMGB1 release induced by liver ischemia involves Toll-like receptor 4 dependent reactive oxygen species production and calcium-mediated signaling. J Exp Med 2007;204:2913–2923.
- Castiglioni A, Canti V, Rovere-Querini P, Manfredi AA. High-mobility group box 1 (HMGB1) as a master regulator of innate immunity. Cell Tissue Res 2011;343:189–199.
- Ivanov S, Dragoi AM, Wang X, Dallacosta C, Louten J, Musco G, Sitia G, et al. A novel role for HMGB1 in TLR9-mediated inflammatory responses to CpG-DNA. Blood 2007;110:1970–1981.
- Luan ZG, Zhang H, Yang PT, Ma XC, Zhang C, Guo RX. HMGB1 activates nuclear factor-?B signaling by RAGE and increases the production of TNF-a in human umbilical vein endothelial cells. Immunobiology 2010;215:956–962.
- Orlova VV, Choi EY, Xie C, Chavakis E, Bierhaus A, Ihanus E, Ballantyne CM, et al. A novel pathway of HMGB1-mediated inflammatory cell recruitment that requires Mac-1-integrin. EMBO J 2007;26:1129–1139.
- Park JS, Arcaroli J, Yum HK, Yang H, Wang H, Yang KY, Choe KH, et al. Activation of gene expression in human neutrophils by high mobility group box 1 protein. Am J Physiol, Cell Physiol 2003;284:C870–C879.
- Park JS, Svetkauskaite D, He Q, Kim JY, Strassheim D, Ishizaka A, Abraham E. Involvement of toll-like receptors 2 and 4 in cellular activation by high mobility group box 1 protein. J Biol Chem 2004;279:7370–7377.
- Park JS, Gamboni-Robertson F, He Q, Svetkauskaite D, Kim JY, Strassheim D, Sohn JW, et al. High mobility group box 1 protein interacts with multiple Toll-like receptors. Am J Physiol, Cell Physiol 2006;290:C917–C924.
- Qin YH, Dai SM, Tang GS, Zhang J, Ren D, Wang ZW, Shen Q. HMGB1 enhances the proinflammatory activity of lipopolysaccharide by promoting the phosphorylation of MAPK p38 through receptor for advanced glycation end products. J Immunol 2009;183:6244–6250.
- Rauvala H, Rouhiainen A. Physiological and pathophysiological outcomes of the interactions of HMGB1 with cell surface receptors. Biochim Biophys Acta 2010;1799:164–170.
- Yang D, Chen Q, Yang H, Tracey KJ, Bustin M, Oppenheim JJ. High mobility group box-1 protein induces the migration and activation of human dendritic cells and acts as an alarmin. J Leukoc Biol 2007;81:59–66.
- De Mori R, Straino S, Di Carlo A, Mangoni A, Pompilio G, Palumbo R, Bianchi ME, et al. Multiple effects of high mobility group box protein 1 in skeletal muscle regeneration. Arterioscler Thromb Vasc Biol 2007;27:2377–2383.
- Palumbo R, Bianchi ME. High mobility group box 1 protein, a cue for stem cell recruitment. Biochem Pharmacol 2004;68:1165–1170.
- Palumbo R, Sampaolesi M, De Marchis F, Tonlorenzi R, Colombetti S, Mondino A, Cossu G, Bianchi ME. Extracellular HMGB1, a signal of tissue damage, induces mesoangioblast migration and proliferation. J Cell Biol 2004;164:441–449.
- Mitola S, Belleri M, Urbinati C, Coltrini D, Sparatore B, Pedrazzi M, Melloni E, Presta M. Cutting edge: Extracellular high mobility group box-1 protein is a proangiogenic cytokine. J Immunol 2006;176:12–15.
- Sorci G, Riuzzi F, Arcuri C, Giambanco I, Donato R. Amphoterin stimulates myogenesis and counteracts the antimyogenic factors basic fibroblast growth factor and S100B via RAGE binding. Mol Cell Biol 2004;24:4880–4894.
- Degryse B, Bonaldi T, Scaffidi P, Müller S, Resnati M, Sanvito F, Arrigoni G, Bianchi ME. The high mobility group (HMG) boxes of the nuclear protein HMG1 induce chemotaxis and cytoskeleton reorganization in rat smooth muscle cells. J Cell Biol 2001;152:1197–1206.
- Limana F, Germani A, Zacheo A, Kajstura J, Di Carlo A, Borsellino G, Leoni O, et al. Exogenous high-mobility group box 1 protein induces myocardial regeneration after infarction via enhanced cardiac C-kit+ cell proliferation and differentiation. Circ Res 2005;97:e73–e83.
- Chavakis E, Hain A, Vinci M, Carmona G, Bianchi ME, Vajkoczy P, Zeiher AM, et al. High-mobility group box 1 activates integrin-dependent homing of endothelial progenitor cells. Circ Res 2007;100:204–212.
- Czura CJ, Tracey KJ. Targeting high mobility group box 1 as a late-acting mediator of inflammation. Crit Care Med 2003;31:S46–S50.
- Wang H, Bloom O, Zhang M, Vishnubhakat JM, Ombrellino M, Che J, Frazier A, et al. HMG-1 as a late mediator of endotoxin lethality in mice. Science 1999;285:248–251.
- Wang H, Yang H, Czura CJ, Sama AE, Tracey KJ. HMGB1 as a late mediator of lethal systemic inflammation. Am J Respir Crit Care Med 2001;164:1768–1773.
- Wang H, Ward MF, Sama AE. Novel HMGB1-inhibiting therapeutic agents for experimental sepsis. Shock 2009;32:348–357.
- Mantell LL, Parrish WR, Ulloa L. Hmgb-1 as a therapeutic target for infectious and inflammatory disorders. Shock 2006;25:4–11.
- Tian J, Avalos AM, Mao SY, Chen B, Senthil K, Wu H, Parroche P, et al. Toll-like receptor 9-dependent activation by DNA-containing immune complexes is mediated by HMGB1 and RAGE. Nat Immunol 2007;8:487–496.
- Bierhaus A, Humpert PM, Morcos M, Wendt T, Chavakis T, Arnold B, Stern DM, Nawroth PP. Understanding RAGE, the receptor for advanced glycation end products. J Mol Med 2005;83:876–886.
- Sims GP, Rowe DC, Rietdijk ST, Herbst R, Coyle AJ. HMGB1 and RAGE in inflammation and cancer. Annu Rev Immunol 2010;28:367–388.
- Dumitriu IE, Baruah P, Valentinis B, Voll RE, Herrmann M, Nawroth PP, Arnold B, et al. Release of high mobility group box 1 by dendritic cells controls T cell activation via the receptor for advanced glycation end products. J Immunol 2005;174:7506–7515.
- Dumitriu IE, Baruah P, Bianchi ME, Manfredi AA, Rovere-Querini P. Requirement of HMGB1 and RAGE for the maturation of human plasmacytoid dendritic cells. Eur J Immunol 2005;35:2184–2190.
- Hori O, Brett J, Slattery T, Cao R, Zhang J, Chen JX, Nagashima M, et al. The receptor for advanced glycation end products (RAGE) is a cellular binding site for amphoterin. Mediation of neurite outgrowth and co-expression of RAGE and amphoterin in the developing nervous system. J Biol Chem 1995;270:25752–25761.
- Kokkola R, Andersson A, Mullins G, Ostberg T, Treutiger CJ, Arnold B, Nawroth P, et al. RAGE is the major receptor for the proinflammatory activity of HMGB1 in rodent macrophages. Scand J Immunol 2005;61:1–9.
- Romero R, Manogue KR, Mitchell MD, Wu YK, Oyarzun E, Hobbins JC, Cerami A. Infection and labor. IV. Cachectin-tumor necrosis factor in the amniotic fluid of women with intraamniotic infection and preterm labor. Am J Obstet Gynecol 1989;161:336–341.
- Romero R, Brody DT, Oyarzun E, Mazor M, Wu YK, Hobbins JC, Durum SK. Infection and labor. III. Interleukin-1: A signal for the onset of parturition. Am J Obstet Gynecol 1989;160:1117–1123.
- Fortunato SJ, Menon R. Distinct molecular events suggest different pathways for preterm labor and premature rupture of membranes. Am J Obstet Gynecol 2001;184:1399–405; discussion 1405.
- Romero R, Chaiworapongsa T, Espinoza J, Gomez R, Yoon BH, Edwin S, Mazor M, et al. Fetal plasma MMP-9 concentrations are elevated in preterm premature rupture of the membranes. Am J Obstet Gynecol 2002;187:1125–1130.
- Kusanovic JP, Romero R, Chaiworapongsa T, Mittal P, Mazaki-Tovi S, Vaisbuch E, Erez O, et al. Amniotic fluid sTREM-1 in normal pregnancy, spontaneous parturition at term and preterm, and intra-amniotic infection/inflammation. J Matern Fetal Neonatal Med 2010;23:34–47.
- Cruciani L, Romero R, Vaisbuch E, Kusanovic JP, Chaiworapongsa T, Mazaki-Tovi S, Mittal P, et al. Pentraxin 3 in amniotic fluid: A novel association with intra-amniotic infection and inflammation. J Perinat Med 2010;38:161–171.
- Maymon E, Ghezzi F, Edwin SS, Mazor M, Yoon BH, Gomez R, Romero R. The tumor necrosis factor α and its soluble receptor profile in term and preterm parturition. Am J Obstet Gynecol 1999;181:1142–1148.
- Romero R, Nores J, Mazor M, Sepulveda W, Oyarzun E, Parra M, Insunza A, et al. Microbial invasion of the amniotic cavity during term labor. Prevalence and clinical significance. J Reprod Med 1993;38:543–548.
- Romero R, Avila C, Santhanam U, Sehgal PB. Amniotic fluid interleukin 6 in preterm labor. Association with infection. J Clin Invest 1990;85:1392–1400.
- Gotsch F, Romero R, Kusanovic JP, Erez O, Espinoza J, Kim CJ, Vaisbuch E, et al. The anti-inflammatory limb of the immune response in preterm labor, intra-amniotic infection/inflammation, and spontaneous parturition at term: A role for interleukin-10. J Matern Fetal Neonatal Med 2008;21:529–547.
- Esplin MS, Romero R, Chaiworapongsa T, Kim YM, Edwin S, Gomez R, Mazor M, Adashi EY. Monocyte chemotactic protein-1 is increased in the amniotic fluid of women who deliver preterm in the presence or absence of intra-amniotic infection. J Matern Fetal Neonatal Med 2005;17:365–373.
- Ghezzi F, Gomez R, Romero R, Yoon BH, Edwin SS, David C, Janisse J, Mazor M. Elevated interleukin-8 concentrations in amniotic fluid of mothers whose neonates subsequently develop bronchopulmonary dysplasia. Eur J Obstet Gynecol Reprod Biol 1998;78:5–10.
- Romero R, Ceska M, Avila C, Mazor M, Behnke E, Lindley I. Neutrophil attractant/activating peptide-1/interleukin-8 in term and preterm parturition. Am J Obstet Gynecol 1991;165:813–820.
- Nhan-Chang CL, Romero R, Kusanovic JP, Gotsch F, Edwin SS, Erez O, Mittal P, et al. A role for CXCL13 (BCA-1) in pregnancy and intra-amniotic infection/inflammation. J Matern Fetal Neonatal Med 2008;21:763–775.
- Mittal P, Romero R, Kusanovic JP, Edwin SS, Gotsch F, Mazaki-Tovi S, Espinoza J, et al. CXCL6 (granulocyte chemotactic protein-2): A novel chemokine involved in the innate immune response of the amniotic cavity. Am J Reprod Immunol 2008;60:246–257.
- Hamill N, Romero R, Gotsch F, Kusanovic JP, Edwin S, Erez O, Than NG, et al. Exodus-1 (CCL20): Evidence for the participation of this chemokine in spontaneous labor at term, preterm labor, and intrauterine infection. J Perinat Med 2008;36:217–227.
- Chaiworapongsa T, Romero R, Espinoza J, Kim YM, Edwin S, Bujold E, Gomez R, Kuivaniemi H. Macrophage migration inhibitory factor in patients with preterm parturition and microbial invasion of the amniotic cavity. J Matern Fetal Neonatal Med 2005;18:405–416.
- Helmig BR, Romero R, Espinoza J, Chaiworapongsa T, Bujold E, Gomez R, Ohlsson K, Uldbjerg N. Neutrophil elastase and secretory leukocyte protease inhibitor in prelabor rupture of membranes, parturition and intra-amniotic infection. J Matern Fetal Neonatal Med 2002;12:237–246.
- Athayde N, Edwin SS, Romero R, Gomez R, Maymon E, Pacora P, Menon R. A role for matrix metalloproteinase-9 in spontaneous rupture of the fetal membranes. Am J Obstet Gynecol 1998;179:1248–1253.
- Athayde N, Romero R, Gomez R, Maymon E, Pacora P, Mazor M, Yoon BH, et al. Matrix metalloproteinases-9 in preterm and term human parturition. J Matern Fetal Med 1999;8:213–219.
- Maymon E, Romero R, Pacora P, Gervasi MT, Edwin SS, Gomez R, Seubert DE. Matrilysin (matrix metalloproteinase 7) in parturition, premature rupture of membranes, and intrauterine infection. Am J Obstet Gynecol 2000;182:1545–1553.
- Maymon E, Romero R, Pacora P, Gervasi MT, Gomez R, Edwin SS, Yoon BH. Evidence of in vivo differential bioavailability of the active forms of matrix metalloproteinases 9 and 2 in parturition, spontaneous rupture of membranes, and intra-amniotic infection. Am J Obstet Gynecol 2000;183:887–894.
- Maymon E, Romero R, Pacora P, Gervasi MT, Bianco K, Ghezzi F, Yoon BH. Evidence for the participation of interstitial collagenase (matrix metalloproteinase 1) in preterm premature rupture of membranes. Am J Obstet Gynecol 2000;183:914–920.
- Maymon E, Romero R, Pacora P, Gomez R, Athayde N, Edwin S, Yoon BH. Human neutrophil collagenase (matrix metalloproteinase 8) in parturition, premature rupture of the membranes, and intrauterine infection. Am J Obstet Gynecol 2000;183:94–99.
- Maymon E, Romero R, Chaiworapongsa T, Kim JC, Berman S, Gomez R, Edwin S. Value of amniotic fluid neutrophil collagenase concentrations in preterm premature rupture of membranes. Am J Obstet Gynecol 2001;185:1143–1148.
- Maymon E, Romero R, Pacora P, Gomez R, Mazor M, Edwin S, Chaiworapongsa T, et al. A role for the 72 kDa gelatinase (MMP-2) and its inhibitor (TIMP-2) in human parturition, premature rupture of membranes and intraamniotic infection. J Perinat Med 2001;29:308–316.
- Alpay Savasan Z, Romero R, Chaiworapongsa T, Kusanovic JP, Kim SK, Mazaki-Tovi S, Vaisbuch E, et al. Evidence in support of a role for anti-angiogenic factors in preterm prelabor rupture of membranes. J Matern Fetal Neonatal Med 2010;23:828–841.
- Seubert DE, Maymon E, Pacora P, Gervasi MT, Berry SM, Torry DS, Romero R. A study of the relationship between placenta growth factor and gestational age, parturition, rupture of membranes, and intrauterine infection. Am J Obstet Gynecol 2000;182:1633–1637.
- Pacora P, Romero R, Chaiworapongsa T, Kusanovic JP, Erez O, Vaisbuch E, Mazaki-Tovi S, et al. Amniotic fluid angiopoietin-2 in term and preterm parturition, and intra-amniotic infection/inflammation. J Perinat Med 2009;37:503–511.
- Erez O, Romer R, Vaisbuch E, Chaiworapongsa T, Kusanovic JP, Mazaki-Tovi S, Gotsch F, et al. Changes in amniotic fluid concentration of thrombin-antithrombin III complexes in patients with preterm labor: Evidence of an increased thrombin generation. J Matern Fetal Neonatal Med 2009;22:971–982.
- Erez O, Romero R, Vaisbuch E, Kusanovic JP, Mazaki-Tovi S, Chaiworapongsa T, Gotsch F, et al. High tissue factor activity and low tissue factor pathway inhibitor concentrations in patients with preterm labor. J Matern Fetal Neonatal Med 2010;23:23–33.
- Mazaki-Tovi S, Romero R, Kusanovic JP, Erez O, Gotsch F, Mittal P, Than NG, et al.Visfatin/Pre-B cell colony-enhancing factor in amniotic fluid in normal pregnancy, spontaneous labor at term, preterm labor and prelabor rupture of membranes: An association with subclinical intrauterine infection in preterm parturition. J Perinat Med 2008;36:485–496.
- Mazaki-Tovi S, Romero R, Vaisbuch E, Kusanovic JP, Erez O, Mittal P, Gotsch F, et al. Adiponectin in amniotic fluid in normal pregnancy, spontaneous labor at term, and preterm labor: A novel association with intra-amniotic infection/inflammation. J Matern Fetal Neonatal Med 2010;23:120–130.
- Vaisbuch E, Mazaki-Tovi S, Kusanovic JP, Erez O, Than NG, Kim SK, Dong Z, et al. Retinol binding protein 4: An adipokine associated with intra-amniotic infection/inflammation. J Matern Fetal Neonatal Med 2010;23:111–119.
- Espinoza J, Chaiworapongsa T, Romero R, Edwin S, Rathnasabapathy C, Gomez R, Bujold E, et al.Antimicrobial peptides in amniotic fluid: Defensins, calprotectin and bacterial/permeability-increasing protein in patients with microbial invasion of the amniotic cavity, intra-amniotic inflammation, preterm labor and premature rupture of membranes. J Matern Fetal Neonatal Med 2003;13:2–21.
- Romero R, Wu YK, Sirtori M, Oyarzun E, Mazor M, Hobbins JC, Mitchell MD. Amniotic fluid concentrations of prostaglandin F2 α, 13,14-dihydro-15-keto-prostaglandin F2 α (PGFM) and 11-deoxy-13,14-dihydro-15-keto-11, 16-cyclo-prostaglandin E2 (PGEM-LL) in preterm labor. Prostaglandins 1989;37:149–161.
- Romero R, Quintero R, Emamian M, Wan M, Grzyboski C, Hobbins JC, Mitchell MD. Arachidonate lipoxygenase metabolites in amniotic fluid of women with intra-amniotic infection and preterm labor. Am J Obstet Gynecol 1987;157:1454–1460.
- Romero R, Emamian M, Wan M, Quintero R, Hobbins JC, Mitchell MD. Prostaglandin concentrations in amniotic fluid of women with intra-amniotic infection and preterm labor. Am J Obstet Gynecol 1987;157:1461–1467.
- Mazor M, Wiznitzer A, Maymon E, Leiberman JR, Cohen A. Changes in amniotic fluid concentrations of prostaglandins E2 and F2 α in women with preterm labor. Isr J Med Sci 1990;26:425–428.
- Romero R, Espinoza J, Hassan S, Gotsch F, Kusanovic JP, Avila C, Erez O, et al.Soluble receptor for advanced glycation end products (sRAGE) and endogenous secretory RAGE (esRAGE) in amniotic fluid: Modulation by infection and inflammation. J Perinat Med 2008;36:388–398.
- Romero R, Quintero R, Nores J, Avila C, Mazor M, Hanaoka S, Hagay Z, et al.Amniotic fluid white blood cell count: A rapid and simple test to diagnose microbial invasion of the amniotic cavity and predict preterm delivery. Am J Obstet Gynecol 1991;165:821–830.
- Romero R, Jimenez C, Lohda AK, Nores J, Hanaoka S, Avila C, Callahan R, et al. Amniotic fluid glucose concentration: A rapid and simple method for the detection of intraamniotic infection in preterm labor. Am J Obstet Gynecol 1990;163:968–974.
- Romero R, Emamian M, Quintero R, Wan M, Hobbins JC, Mazor M, Edberg S. The value and limitations of the Gram stain examination in the diagnosis of intraamniotic infection. Am J Obstet Gynecol 1988;159:114–119.
- Lotze MT, Tracey KJ. High-mobility group box 1 protein (HMGB1): Nuclear weapon in the immune arsenal. Nat Rev Immunol 2005;5:331–342.
- Jiang W, Li J, Gallowitsch-Puerta M, Tracey KJ, Pisetsky DS. The effects of CpG DNA on HMGB1 release by murine macrophage cell lines. J Leukoc Biol 2005;78:930–936.
- Chen G, Li J, Ochani M, Rendon-Mitchell B, Qiang X, Susarla S, Ulloa L, et al. Bacterial endotoxin stimulates macrophages to release HMGB1 partly through CD14- and TNF-dependent mechanisms. J Leukoc Biol 2004;76:994–1001.
- Mullins GE, Sunden-Cullberg J, Johansson AS, Rouhiainen A, Erlandsson-Harris H, Yang H, Tracey KJ, et al. Activation of human umbilical vein endothelial cells leads to relocation and release of high-mobility group box chromosomal protein 1. Scand J Immunol 2004;60:566–573.
- Wähämaa H, Vallerskog T, Qin S, Lunderius C, LaRosa G, Andersson U, Harris HE. HMGB1-secreting capacity of multiple cell lineages revealed by a novel HMGB1 ELISPOT assay. J Leukoc Biol 2007;81:129–136.
- Jiang W, Pisetsky DS. The role of IFN-α and nitric oxide in the release of HMGB1 by RAW 264.7 cells stimulated with polyinosinic-polycytidylic acid or lipopolysaccharide. J Immunol 2006;177:3337–3343.
- Rendon-Mitchell B, Ochani M, Li J, Han J, Wang H, Yang H, Susarla S, et al. IFN-γ induces high mobility group box 1 protein release partly through a TNF-dependent mechanism. J Immunol 2003;170:3890–3897.
- Faraco G, Fossati S, Bianchi ME, Patrone M, Pedrazzi M, Sparatore B, Moroni F, Chiarugi A. High mobility group box 1 protein is released by neural cells upon different stresses and worsens ischemic neurodegeneration in vitro and in vivo. J Neurochem 2007;103:590–603.
- Gardella S, Andrei C, Ferrera D, Lotti LV, Torrisi MR, Bianchi ME, Rubartelli A. The nuclear protein HMGB1 is secreted by monocytes via a non-classical, vesicle-mediated secretory pathway. EMBO Rep 2002;3:995–1001.
- Liu S, Stolz DB, Sappington PL, Macias CA, Killeen ME, Tenhunen JJ, Delude RL, Fink MP. HMGB1 is secreted by immunostimulated enterocytes and contributes to cytomix-induced hyperpermeability of Caco-2 monolayers. Am J Physiol, Cell Physiol 2006;290:C990–C999.
- Chu JJ, Ng ML. The mechanism of cell death during West Nile virus infection is dependent on initial infectious dose. J Gen Virol 2003;84:3305–3314.
- Alleva LM, Budd AC, Clark IA. Systemic release of high mobility group box 1 protein during severe murine influenza. J Immunol 2008;181:1454–1459.
- Hofner P, Seprényi G, Miczák A, Buzás K, Gyulai Z, Medzihradszky KF, Rouhiainen A, et al. High mobility group box 1 protein induction by Mycobacterium bovis BCG. Mediators Inflamm 2007;2007:53805.
- Grover A, Taylor J, Troudt J, Keyser A, Sommersted K, Schenkel A, Izzo AA. Mycobacterial infection induces the secretion of high-mobility group box 1 protein. Cell Microbiol 2008;10:1390–1404.
- Crikis S, Zhang XM, Dezfouli S, Dwyer KM, Murray-Segal LM, Salvaris E, Selan C, et al. Anti-inflammatory and anticoagulant effects of transgenic expression of human thrombomodulin in mice. Am J Transplant 2010;10:242–250.
- Nagato M, Okamoto K, Abe Y, Higure A, Yamaguchi K. Recombinant human soluble thrombomodulin decreases the plasma high-mobility group box-1 protein levels, whereas improving the acute liver injury and survival rates in experimental endotoxemia. Crit Care Med 2009;37:2181–2186.
- Ito T, Kawahara K, Okamoto K, Yamada S, Yasuda M, Imaizumi H, Nawa Y, et al. Proteolytic cleavage of high mobility group box 1 protein by thrombin-thrombomodulin complexes. Arterioscler Thromb Vasc Biol 2008;28:1825–1830.
- Chen GY, Tang J, Zheng P, Liu Y. CD24 and Siglec-10 selectively repress tissue damage-induced immune responses. Science 2009;323:1722–1725.
- Liu Y, Chen GY, Zheng P. CD24-Siglec G/10 discriminates danger- from pathogen-associated molecular patterns. Trends Immunol 2009;30:557–561.
- Hasegawa A, Iwasaka H, Hagiwara S, Noguchi T. Relationship Between HMGB1 and Tissue Protective Effects of HSP72 in a LPS-Induced Systemic Inflammation Model. J Surg Res 2011;169:85–91.
- Huston JM, Gallowitsch-Puerta M, Ochani M, Ochani K, Yuan R, Rosas-Ballina M, Ashok M, et al. Transcutaneous vagus nerve stimulation reduces serum high mobility group box 1 levels and improves survival in murine sepsis. Crit Care Med 2007;35:2762–2768.
- Pavlov VA, Ochani M, Yang LH, Gallowitsch-Puerta M, Ochani K, Lin X, Levi J, et al. Selective α7-nicotinic acetylcholine receptor agonist GTS-21 improves survival in murine endotoxemia and severe sepsis. Crit Care Med 2007;35:1139–1144.
- Rouhiainen A, Tumova S, Valmu L, Kalkkinen N, Rauvala H. Pivotal advance: Analysis of proinflammatory activity of highly purified eukaryotic recombinant HMGB1 (amphoterin). J Leukoc Biol 2007;81:49–58.
- Yang H, Ochani M, Li J, Qiang X, Tanovic M, Harris HE, Susarla SM, et al. Reversing established sepsis with antagonists of endogenous high-mobility group box 1. Proc Natl Acad Sci USA 2004;101:296–301.
- Bianchi ME, Manfredi AA. Immunology. Dangers in and out. Science 2009;323:1683–1684.
- Erlandsson Harris H, Andersson U. Mini-review: The nuclear protein HMGB1 as a proinflammatory mediator. Eur J Immunol 2004;34:1503–1512.
- Silva E, Arcaroli J, He Q, Svetkauskaite D, Coldren C, Nick JA, Poch K, et al.HMGB1 and LPS induce distinct patterns of gene expression and activation in neutrophils from patients with sepsis-induced acute lung injury. Intensive Care Med 2007;33:1829–1839.
- Klune JR, Dhupar R, Cardinal J, Billiar TR, Tsung A. HMGB1: Endogenous danger signaling. Mol Med 2008;14:476–484.
- Lotze MT, Deisseroth A, Rubartelli A. Damage associated molecular pattern molecules. Clin Immunol 2007;124:1–4.
- Wang Y, Wang H, Piper MG, McMaken S, Mo X, Opalek J, Schmidt AM, Marsh CB. sRAGE induces human monocyte survival and differentiation. J Immunol 2010;185:1822–1835.
- Pullerits R, Brisslert M, Jonsson IM, Tarkowski A. Soluble receptor for advanced glycation end products triggers a proinflammatory cytokine cascade via β2 integrin Mac-1. Arthritis Rheum 2006;54:3898–3907.
- Qiu J, Xu J, Zheng Y, Wei Y, Zhu X, Lo EH, Moskowitz MA, Sims JR. High-mobility group box 1 promotes metalloproteinase-9 upregulation through Toll-like receptor 4 after cerebral ischemia. Stroke 2010;41:2077–2082.
- Ferrand PE, Parry S, Sammel M, Macones GA, Kuivaniemi H, Romero R, Strauss JF 3rd . A polymorphism in the matrix metalloproteinase-9 promoter is associated with increased risk of preterm premature rupture of membranes in African Americans. Mol Hum Reprod 2002;8:494–501.
- Fortunato SJ, Menon R, Lombardi SJ. MMP/TIMP imbalance in amniotic fluid during PROM: An indirect support for endogenous pathway to membrane rupture. J Perinat Med 1999;27:362–368.
- Dubicke A, Andersson P, Fransson E, Andersson E, Sioutas A, Malmström A, Sverremark-Ekström E, Ekman-Ordeberg G. High-mobility group box protein 1 and its signalling receptors in human preterm and term cervix. J Reprod Immunol 2010;84:86–94.
- Holmlund U, Wähämaa H, Bachmayer N, Bremme K, Sverremark-Ekström E, Palmblad K. The novel inflammatory cytokine high mobility group box protein 1 (HMGB1) is expressed by human term placenta. Immunology 2007;122:430–437.