Abstract
A new oscillatory device administers predetermined pressure oscillation sequences into the chest cavity over inhaled/exhaled air streams at low positive pressure. We assessed device safety and effect on 6MW performance, pulmonary function, and health-related quality-of-life (HRQOL) in moderate-to-very severe COPD in a randomized, double-blind, controlled, crossover study. Outcomes with an oscillatory device (PulsehalerTM, Respinova Ltd, Herzliya, Israel) and a “muted” sham device (control) of identical appearance that delivered continuous positive air pressure were compared in two groups receiving opposite treatment sequences: 2-week oscillatory device/control, 2-week washout, 2-week control/oscillatory device, 2-week washout. The clinical trial was registered (www.clinicaltrials.gov, NCT00821418) and approved by the Hadassah—Hebrew University Medical Center Institutional Review Board (08–608). All participants signed informed consent; 22 patients completed the study with no marked differences in COPD exacerbations or side effects. A total of 91% of patients treated with the oscillatory device had a clinically significant improvement (increase >40 m) in 6MW performance. The 6MW distance with the oscillatory device increased significantly after 1 week of treatment (51.6 ± 7.6 m, +13.5 ± 2.3%, p < 0.001), and more after 2 weeks (61.8 ± 9.0 m, 16.3 ± 2.7%, p < 0.001). This increase with the oscillatory device was significantly greater (p < 0.001) than the 15.4 ± 10.4 m increase (4.2 ± 2.6%, NS) with control. FVC and inspiratory capacity (IC) improved significantly (p = 0.03 for each) with the oscillatory device but not with control. HRQL improved markedly (≥1 point) for dyspnea and mastery with the oscillatory device (p = 0.02) but not control. Treatment with a new oscillatory device appears to be safe, and to improve 6MW performance, pulmonary function, and HRQL in COPD. Further evaluation is warranted.
Introduction
Chronic obstructive pulmonary disease (COPD) is characterized by persistent airway inflammation leading to impaired airway function, exercise capability, and quality of life (QOL), with substantial morbidity and mortality (Citation1). Over the last decade, new medications have led to an effective reduction in airway obstruction and frequency of COPD exacerbations, and to improved QOL (Citation2). Studies have documented the benefits of pulmonary rehabilitation in improving 6MW performance and QOL (Citation3, 4). Nevertheless, there is clearly a need for additional therapeutic modalities in COPD (Citation2).
Several studies have evaluated the effects of short term oscillations on physiological parameters of breathing. In normal subjects, oscillations synchronized to the respiratory cycle decreased the slope of breathlessness elicited by inspiratory load combined with hypercapnia (Citation5). Improved oxygenation and reduced end-tidal carbon dioxide pressure following short-term application of oscillations to the chest were found in normal subjects (Citation6) and COPD patients (Citation7). In COPD patients, oscillations significantly reduced the perception of dyspnea and reduced the respiratory rate (Citation8). It is possible, therefore, that a relatively brief daily exposure could affect significant outcome measures, in a manner similar to what has been described, for example, in the use of ultrasound for physical therapy (Citation9).
A new oscillatory airway pressure device (Pulsehaler™, Respinova LTD, Herzlyia, Israel) administers sequences of pressure oscillations into the chest cavity over the entire breathing cycle. The oscillations are controlled to have specific frequency, waveform, pressure amplitude, and duration according to a preprogrammed protocol. In contrast to previous use of oscillations, this oscillatory device and its protocol were designed specifically for the treatment of COPD. We compared outcomes from treatment with this novel oscillatory device, and treatment with a control sham device in which the oscillatory mechanism was muted, in patients with stable, moderate-to-severe COPD under optimal medical management.
As application of short-term oscillatory devices has improved dyspnea and lung functions in normal (Citation5, 6) and COPD patients (Citation7, 8), we hypothesized that a short intervention with this new device could reduce air trapping and reduce the perception of dyspnea, possibly leading to improved exercise capacity. The present study therefore aimed to assess the safety and potential efficacy of the oscillatory device in improving exercise capacity assessed by 6-minute walk (6MW) in patients with stable GOLD grades II-IV (moderate-to-very severe) COPD; and secondarily to evaluate its effects on pulmonary function and health-related quality of life (HRQL).
Methods
This study was a double-blind, controlled, crossover, randomized, safety and efficacy, prospective trial. All participating patients had been diagnosed with COPD, and were under medical treatment in the Institute of Pulmonary Medicine at the Hadassah-Hebrew University Medical Center, Jerusalem. The study was approved by Hadassah's Institutional Review Board (approval 08–608); all participants signed informed consent. The clinical trial was registered with www.clinicaltrials.gov (NCT 00821418).
Patients
Patients who had been diagnosed with COPD (post-bronchodilator FEV1/FVC < 0.7), according to GOLD criteria (Citation1) were evaluated for the study. Inclusion criteria were age >40 years, post-bronchodilator FEV1 <70% of predicted value, a smoking history ≥10 pack-years, and stable COPD with no upper respiratory infections, no exacerbation, and no change in therapy for 30 days prior to study enrollment. Patients with bullous emphysema on CT or with other significant pulmonary, cardiac, or systemic disease were excluded. Occurrence of a COPD exacerbation halted the study protocol for 4 weeks in the affected patient, who then returned to the beginning of the current stage in the study protocol. Only data from patients who completed both arms of treatment were used for the analysis of efficacy.
Device
The oscillatory airway pressure device used in this study administers preprogrammed sequences of pressure oscillations into the chest cavity using air within the airways as the carrier medium. The device is equipped with a mouthpiece that is held between the patient's lips, and a software-controlled mechanism that generates the low-pressure (3.5–5.5 cmH2O) pulsatile airflow according to protocol. The current model uses a commercially available CPAP generator (DeVilbiss 9000D, by DeVilbiss Healthcare, Somerset, PA) to create a constant pressure airstream, modulated to carry the oscillations. Oscillations are generated according to a preprogrammed protocol that defines their frequency (3.5 Hz–55 Hz), waveform, pressure amplitude, and duration of oscillation (30 seconds to several minutes). The oscillation protocol is administered throughout the breathing cycle, both inspiration and expiration. A schemata of the device used is shown in .
Figure 1. A schemata of the pulse device used in the study. A: Constant airflow inlet; B: Oscillating airflow outlet into patient's mouth.
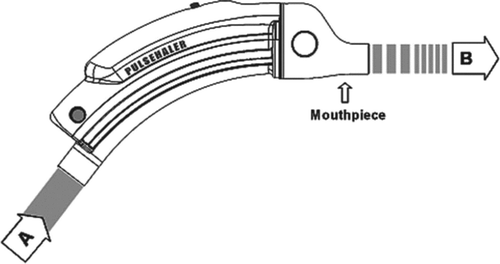
Each patient was treated by an oscillatory device and by a sham version of the device in which the frequency protocol was disabled and only a low-level continuous-pressure airstream was delivered (control device). Although patients could have perceived some difference between the devices, they received no information that could enable them to determine which was the therapeutic or the sham device.
Study design
To assess treatment safety and efficacy of the oscillatory device, a double-blind crossover trial was designed (, 3). The study coordinator enrolled patients and randomly assigned them to a group. Patients, as well as all physicians, nurses, and technicians providing direct care or interacting with patients, were blinded to group assignments. Patients in Group 1 underwent treatment with the oscillatory device in Period I and the oscillation-disabled control device in Period II. Patients in Group 2 were treated in reverse order, with control treatment during Period I and the oscillatory device during Period II. Patients were instructed on the use of the devices without specifying whether the treatment received was oscillatory (functional) or sham. There was a 2-week washout interval after each period. During each study period, patients treated themselves with the devices when seated and at rest, for 20 minutes, 3 times daily, for 2 weeks. Treatments were provided as an add-on to existing COPD therapy.
Compliance
Patients reported the number of treatments they actually performed. Adherence to therapy was confirmed by the study coordinator, who called patients daily by telephone and visited them at home, documenting protocol adherence.
Outcome Measures
Safety
To evaluate device safety, patients were monitored for COPD exacerbations and all other medical events throughout the study.
Efficacy
For each period, exercise capacity during 6MW and lung function were evaluated before the first treatment in each study period, after 1 and 2 weeks of treatment, and 2 weeks after completion of each period (). HRQL assessment was performed before and at the end of each period.
Exercise capacity
Physical capacity was assessed using the 6MW test, performed according to American Thoracic Society (ATS) guidelines (Citation10).
Pulmonary function
Spirometry was performed using a pneumotachograph-based system (Medgraphics, St. Paul, MN), based on reference equations and corrections published by the European Community for Coal and Steel (ECCS) (Citation11). No ethnic correction factors were used. Forced expiratory volume (FEV1), forced vital capacity (FVC), DLCO, inspirational capacity (IC), and residual volume to total lung capacity ratio (RV/TLC) were assessed at rest. Oxygen saturation was measured at rest and after 6MW at each assessment interval.
Health-related quality-of-life
HRQL was assessed using the Chronic Respiratory Questionnaire–Self Administered Standardized format (CRQ-SAS, McMaster University) for patients with chronic lung diseases (Citation12), spanning the domains of dyspnea, fatigue, emotional function, and mastery. The CRQ is designed to detect and quantify clinically significant changes that are perceived by the patient as important in daily life. An average improvement of 0.5 points per question in a domain is the minimal level of change that is clinically important. Changes between 0.75 and 1.25 represent important changes of moderate magnitude, and changes greater than 1.5 represent important changes of large magnitude (Citation13, 14).
Data analysis
For each patient, the differences between baseline measurements of 6MW performance, lung function, HRQL, and safety assessment findings were compared with measurements after 1 and 2 weeks of treatment with the oscillatory-and sham devices (Table 3). Mean change following treatment with the oscillatory device, and mean change after sham device treatments were compared. The study population was not designed to enable subgroup analysis.
Statistical methods
The two-sample t-test was applied for testing the statistical significance of changes between the study groups from baseline to each of the continuous assessments. An analysis of variance (ANOVA) model with repeated measurements was applied for analyzing changes over time in continuous parameters between treatment periods. All tests applied were two-tailed, and a p-value of 5% or less was considered statistically significant. The data was analyzed by MediStat Ltd. (Tel Aviv, Israel) using the SAS® version 9.1 for Windows (SAS Institute, Cary, NC).
Results
Study population
Among 34 patients who were assessed, 29 met inclusion and exclusion criteria and gave informed consent (). Seven did not complete the study; three in Group 1 and four in Group 2, as detailed in . Analysis of efficacy thus included data from 22 patients who completed both treatment cycles. Baseline characteristics of these patients are presented in . No patient was lost to follow-up.
Table 1. Baseline characteristics of patients
Compliance
Patients treated themselves 2.8 ± 0.3 times daily with the oscillatory device, and 2.6 ± 0.5 times daily with the control device.
Air pressure
Positive air pressure was set to the maximal value that was comfortable for each patient just prior to treatment one in Period I. Air pressure was maintained at the same level for each patient for the second (crossover) period unless modification was required due to patient discomfort. Mean pressure settings during treatment with the oscillatory device were 4.9 ± 0.2 in Group 1 and 4.2 ± 0.3 in Group 2; mean pressure settings for control treatment were 4.6 ± 0.2 and 4.5 ± 0.2, respectively. There was no significant difference in therapeutic air pressure settings for treatment versus control, whether treatment with the oscillatory device preceded or followed sham control.
Safety
Medical events during the trial are presented in . Six of 8 patients who experienced COPD exacerbations returned to the study following a 4-week break and completed the full crossover protocol.
Table 2. Medical events during the trial
Exercise capacity
After 1 week of treatment with the oscillatory device, exercise capacity, as assessed by the 6MW test, improved by 51.6±7.6 m (13.5 ± 2.3% change, p < 0.001), whereas there was no change with the control treatment (1 ± 7.8, 1 ± 2.1% change, NS). At the end of the second week of treatment, 6MW with the oscillatory device improved by 61.8 ± 9.0 m compared to baseline (16.3 ± 2.7% change, p < 0.001), whereas with the control device there was only a mild non-significant change (15.4 ± 10.4 m compared to baseline, 4.2 ± 2.6% change, p = 0.16). The difference between the change with the oscillatory device and the change with the sham device was strongly significant at both time points (p < 0.001). When the maximal value of 6MW was compared between the 2 groups, both the oscillatory (80 ± 7.7 m, 21% ± 2.6 change, p < 0.001) and the control device (21 ± 9.4 m, 5% ± 2.6 change, p = 0.037) improved 6MW performance. However, the improvement in 6MW with the test device was significantly greater than improvement with the control device (p < 0.001) (). A change of 25–35 m (10–14%) in the 6MW is considered to be clinically important (Citation15, 16). The change produced by the test device greatly surpassed this threshold, yet the mean change following control treatment fell below the threshold. During treatment with the oscillatory device, 91% of patients (20/22) increased their 6 MW distance more than 40 m (, top bar). shows the individual maximal changes in the 6 MW test compared to baseline for each patient.
Figure 4. Percentage of patients responding (responders) to treatment with the oscillatory device. Percentage of patients with a clinically significant improvement (responders) following treatment with the oscillatory device. The following improvements were defined as clinically significant: >40 m in 6MW; >12% in FVC; >12% in FEV1; <0.05 in RV/TLC (abs); >1 in dyspnea score; >1 in mastery score.
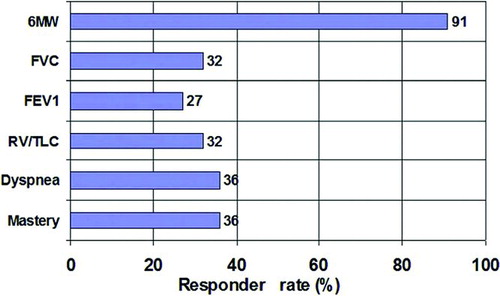
Figure 5. Changes in the 6-minute walk (6MW) test. Maximal change in the 6MW test compared to baseline for each patient with the sham device (left) and the oscillatory device (right).
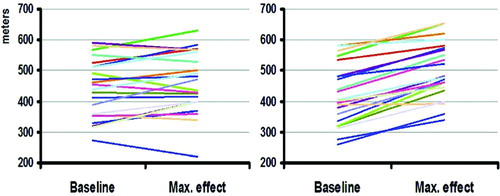
Table 3. Changes induced by oscillatory and control device treatments measured 1 and 2 weeks after the beginning of each round of treatment
Pulmonary function
Pulmonary function test results are presented in . No parameter improved significantly during control treatment. With the oscillatory device there was significant improvement in FVC (201 ± 89 ml, p = 0.03) and IC (122 ± 53 ml, p = 0.03) after 2 weeks of treatment. The improvement in FVC with the oscillatory device at 2 weeks was significantly better (p = 0.01) than with the sham device (–141 ± 93 ml). There was no significant improvement in FEV1, RV/TLC or DLCO with either the oscillatory-or control device (). Resting-and post-exercise oxygen saturation improved slightly (1–2%) with both the oscillatory device and control treatment. The percentage of responders to the oscillatory device for each performance measure throughout the study is shown in .
Quality of life
Both dyspnea and mastery scores changed significantly during treatment with the oscillatory device; 36% were responders for each score (p < 0.05, and ), with an average change >1, reaching the second level of clinical significance, which is considered a change of moderate magnitude. Treatment with the control device did not improve any of the four HRQOL categories ().
Analysis of data collected from the HRQOL questionnaires revealed a period effect, referring to the situation in which the results obtained from an intervention applied in Period I differ from those obtained from the same intervention applied in Period II. This is a known phenomenon of the crossover methodology (Citation17, 18). Analysis of data from all patients, ignoring the period effect, showed no significant differences between groups (data not shown). Data presented above was analyzed using a correction commonly used in this situation (Citation18, 19), which is analysis of only the first period of the study.
Discussion
In this randomized, controlled, double-blind, crossover study we found the oscillatory device to be safe. Treatment with this new device significantly improved 6MW performance, FVC, IC, and aspects of HRQOL. Treatment with the control device did not significantly improve any of the evaluated measures.
The exact mechanism of action by which this oscillatory device improved these parameters has not been elucidated, and requires further research. However, it seems possible that significant mechanical improvement in vital capacity and inspiratory capacity made important contributions to the improvement in exercise capacity.
Clearly, mechanical and functional improvements in pulmonary function are associated with improved QOL. Exercise capacity may have been further improved by effects on the cardiovascular system, which may be assessed in future studies. For example, van Gestel et al. have recently shown that resting parasympathetic tone, as measured by heart rate variability (HRV), is independently associated with QOL and dyspnea in COPD patients (Citation20).
Oscillatory phenomena have been shown to have biological effects on cells and tissues in normal subjects (Citation6, Citation21), as well as in CF (Citation22–25) and COPD patients (Citation7). One important effect of oscillatory phenomena, demonstrated mainly in CF patients, is the effect on mucus clearance. Forced expiration using vibration techniques at a relatively nonspecific frequency range of 5–30 Hz was shown to elicit cough and expectoration (Citation22–24, Citation26–29). In the present study, COPD was not predominated by chronic bronchitis. Mucus expectoration was not quantified; thus no assessment of the relationship between expectoration and the benefit from the vibrations could be made.
Oscillations have been also shown to affect pulmonary function. High frequency chest wall oscillation was shown to affect total lung capacity (TLC), functional residual capacity (FRC), tidal volume (Vt), and effective alveolar ventilation, depending on the frequency, negative pressure, and pressure amplitudes exerted over the chest wall (Citation21). Oscillations were shown to have a waveform-dependent effect on air trapping (RV/TLC) and expiratory reserve volume in CF patients (Citation22). Oscillatory frequencies of 0.5–1.0 Hz improved oxygenation, decreased end-tidal carbon dioxide pressure (PCO2), and improved the spontaneous respiratory rate in normal subjects (Citation6). Reduction of end-tidal PCO2 and increased oxygen saturation were also shown in eucapnic and hypercapnic COPD patients following application of 1–2.3 Hz oscillations to the chest (Citation7).
Intercostal muscle vibrations, applied externally and synchronized to the inspiration segment of the respiratory cycle, decreased dyspnea and reduced respiratory rates in normal subjects and COPD patients (Citation8). These changes may be related to vibratory stimulus of muscle spindles within the intercostal muscles, which are known to play an important role in producing or modifying dyspnea (Citation30). In animal studies, oscillations were shown to depress the active force generated by airway smooth muscle (Citation31), possibly due to changes in the organization of contractile filaments within smooth muscle cells. An opposite effect was reported on tracheal smooth muscle tone, where an increase in active force was accompanied by coughing, swallowing, and sighing (Citation32).
There is some data on the effect of positive expiratory pressure (PEP) and continuous positive air pressure (CPAP) in stable COPD (Citation32, 33). Holanda et al. have recently shown that CPAP, only in low pressure levels (5 cmH2O), may cause regional lung deflation in COPD patients (Citation34). In our study, the sham CPAP device did not improve any of the parameters tested.
The oscillatory airway pressure device used in this study was developed specifically for COPD. It uses the airways as a conduit to administer pulses and oscillations into the chest cavity, according to a preprogrammed protocol. This new device has the potential to be incorporated as a self-administered technique, adding to current COPD treatments. It is a unique and simple system, enabling patients with COPD to physically improve their resting pulmonary function, their exercise performance, and their perceived QOL. A potential advantage is that patients are independent in treatment, thus consuming fewer healthcare resources. The device is home-based, requires minimal disposable materials, and can be used passively, without skilled training or supervision.
This small, short-term study showed clinically significant responses within a 2-week treatment period (, ). These improvements were achieved in patients who were stable at recruitment, and there were no changes in their medical treatment regimen during the study period aside from adding treatment with the oscillatory and sham devices. Furthermore, in this small cohort, patients with lower baseline exercise capacity, as evaluated by 6MW, seemed to have greater improvement in their subsequent, post-treatment 6MW test. Larger long-term studies are required to define the optimal protocol regimen for improvement in pulmonary function and exercise ability. Further studies are also required to study the efficacy of this new device when used in combination with pulmonary rehabilitation, and for the reduction in the frequency and duration of COPD exacerbations.
Conclusions
In summary, we report promising findings from the first randomized, double-blind, controlled, crossover study using a novel oscillatory device for potential treatment of COPD. A 2-week treatment regimen with an oscillatory device improved 6MW performance, pulmonary function, and health-related quality of life in patients with moderate-to-very-severe COPD. Determining the optimal role of this novel oscillatory device alongside current therapy and rehabilitation programs calls for further research.
Acknowledgements
The authors wish to thank Shifra Fraifeld, a Senior Editor at the Hadassah—Hebrew University Medical Center, for her editorial assistance in the preparation of this manuscript.
Author contributions: 1— AL, GF, RB contributed to study conception and design, ZGF, GF, NA, UL, RB contributed to data acquisition and ZGF, NA, NB, AR, GF, RB to data interpretation and analysis; 2— ZGF, AL, RB drafted the manuscript, ZGF, NS, UL, NB, AL, GF, RB critically revised the paper, and ZGF, NB, AL, GF, RB contributed significantly intellectual content; 3—all authors approved the final version of the manuscript. RB is the guarantor of the work, taking responsibility for the integrity of the study as a whole, from inception to the published article.
Declaration of Interests
Respinova Ltd. underwrote the cost of the study and paid for statistical analysis. Study design; data collection/control, analysis, and interpretation; manuscript preparation; and submission decisions were completed at the Medical Center, in consultation with an independent statistician. Respinova approved the study design, had access to data, and approved the final manuscript and submission decisions.
A.L. is the Medical Director (consultant) for Respinova. R.B. and G.F. were awarded no-fee stock options in the company; however, this direct relationship was discussed and established only after completion of the study and manuscript preparation. No other funding was received and no other specific or general potential conflict exists for any author with relation to the manuscript.
References
- Global initiative for the diagnosis, management, and prevention of chronic obstructive pulmonary disease. Pocket guide to COPD diagnosis, management, and prevention. A guide for health care professionals. Journal (serial on the Internet). 2010 Date: Available from: http://www.goldcopd.org/uploads/users/files/GOLD_Pocket_2010Mar31.pdf. (Accessed July 31, 2011).
- Niewoehner DE. Clinical practice. Outpatient management of severe COPD. N Engl J Med 2010 Apr 15; 362(15):1407–1416.
- Casaburi R, ZuWallack R. Pulmonary rehabilitation for management of chronic obstructive pulmonary disease. N Engl J Med 2009 Mar 26; 360(13):1329–1335.
- Lacasse Y, Goldstein R, Lasserson TJ, Martin S. Pulmonary rehabilitation for chronic obstructive pulmonary disease. Cochrane Database Syst Rev 2006(4):CD003793.
- Edo H, Kimura H, Niijima M, Sakabe H, Shibuya M, Kanamaru A, Homma I, Kuriyama T. Effects of chest wall vibration on breathlessness during hypercapnic ventilatory response. J Appl Physiol 1998 May; 84(5):1487–1491.
- Hardinge FM, Davies RJ, Stradling JR. Effects of short term high frequency negative pressure ventilation on gas exchange using the Hayek oscillator in normal subjects. Thorax 1995 Jan; 50(1):44–49.
- Spitzer SA, Fink G, Mittelman M. External high-frequency ventilation in severe chronic obstructive pulmonary disease. Chest 1993 Dec; 104(6):1698–1701.
- Sibuya M, Yamada M, Kanamaru A, Tanaka K, Suzuki H, Noguchi E, Altose MD, Homma I. Effect of chest wall vibration on dyspnea in patients with chronic respiratory disease. Am J Respir Crit Care Med 1994 May; 149(5):1235–1240.
- Mason TJ. Therapeutic ultrasound an overview. Ultrason Sonochem 2011 Jul; 18(4):847–852.
- ATS statement: guidelines for the six-minute walk test. Am J Respir Crit Care Med 2002 Jul 1; 166(1):111–117.
- Quanjer PH, Tammeling GJ, Cotes JE, Pedersen OF, Peslin R, Yernault JC. Lung volumes and forced ventilatory flows. Report Working Party Standardization of Lung Function Tests, European Community for Steel and Coal. Official Statement of the European Respiratory Society. Eur Respir J Suppl 1993 Mar; 16:5–40.
- Schunemann HJ, Guyatt GH, Stahl E, Stubbing D, Mador J, McKim D, Austin P, Goldstein R. Validation of a new version of the chronic respiratory questionnaire (CRQ): the self-administred standardized CRQ. Eur Respir J 2002; 20:156S.
- Juniper EF, Guyatt GH, Willan A, Griffith LE. Determining a minimal important change in a disease-specific Quality of Life Questionnaire. J Clin Epidemiol 1994 Jan; 47(1):81–87.
- Redelmeier DA, Guyatt GH, Goldstein RS. Assessing the minimal important difference in symptoms: a comparison of two techniques. J Clin Epidemiol 1996 Nov; 49(11):1215–1219.
- Holland AE, Hill CJ, Rasekaba T, Lee A, Naughton MT, McDonald CF. Updating the minimal important difference for six-minute walk distance in patients with chronic obstructive pulmonary disease. Arch Phys Med Rehabil 2010 Feb; 91(2):221–225.
- Puhan MA, Mador MJ, Held U, Goldstein R, Guyatt GH, Schunemann HJ. Interpretation of treatment changes in 6-minute walk distance in patients with COPD. Eur Respir J 2008 Sep; 32(3): 637–643.
- Koehne-Voss S, Schmidli H, Smith DM, Pigeot I. The impact of period effects on dose level contrasts in alternating cross-over designs for first-time-in-human studies. Pharm Stat 2011 Jan; 10(1):45–49.
- Heller SR, Amiel SA, Mansell P. Effect of the fast-acting insulin analog lispro on the risk of nocturnal hypoglycemia during intensified insulin therapy. U.K. Lispro Study Group. Diabetes Care 1999 Oct; 22(10):1607–1611.
- Johnson KE, Sanders JJ, Gellin RG, Palesch YY. The effectiveness of a magnetized water oral irrigator (Hydro Floss) on plaque, calculus and gingival health. J Clin Periodontol 1998 Apr; 25(4):316–321.
- Van Gestel AJ, Kohler M, Steier J, Teschler S, Russi EW, Teschler H. Cardiac autonomic dysfunction and health-related quality of life in patients with chronic obstructive pulmonary disease. Respirology 2011 Aug;16(6):939–946.
- Petros AJ, Fernando SS, Shenoy VS, al-Saady NM. The Hayek oscillator. Nomograms for tidal volume and minute ventilation using external high frequency oscillation. Anaesthesia 1995 Jul; 50(7):601–606.
- Kempainen RR, Williams CB, Hazelwood A, Rubin BK, Milla CE. Comparison of high-frequency chest wall oscillation with differing waveforms for airway clearance in cystic fibrosis. Chest 2007 Oct; 132(4):1227–1232.
- Konstan MW, Stern RC, Doershuk CF. Efficacy of the Flutter device for airway mucus clearance in patients with cystic fibrosis. J Pediatr 1994 May; 124(5 Pt 1):689–693.
- Marks JH, Hare KL, Saunders RA, Homnick DN. Pulmonary function and sputum production in patients with cystic fibrosis: a pilot study comparing the PercussiveTech HF device and standard chest physiotherapy. Chest 2004 Apr; 125(4):1507–1511.
- Myers TR. Positive expiratory pressure and oscillatory positive expiratory pressure therapies. Respir Care 2007 Oct; 52(10):1308–1326; discussion 27.
- Alves LA, Pitta F, Brunetto AF. Performance analysis of the Flutter VRP1 under different flows and angles. Respir Care 2008 Mar; 53(3):316–323.
- Cantin AM, Bacon M, Berthiaume Y. Mechanical airway clearance using the frequencer electro-acoustical transducer in cystic fibrosis. Clin Invest Med 2006 Jun; 29(3):159–165.
- Marks JH, Homnick DN, Hare KL, Cucos D. The Percussivetech HF compared to the Flutter device in cystic fibrosis patients: a six month pilot study. Journal (serial on the Internet). 1999 Date: Available from: http://www.vortran.com/images/stories/printables/P-NEB-User-Guide.pdf (Accessed July 31, 2011).
- Volsko TA, DiFiore J, Chatburn RL. Performance comparison of two oscillating positive expiratory pressure devices: Acapella versus Flutter. Respir Care 2003 Feb; 48(2):124–130.
- Homma I, Obata T, Sibuya M, Uchida M. Gate mechanism in breathlessness caused by chest wall vibration in humans. J Appl Physiol 1984 Jan; 56(1):8–11.
- Shen X, Wu MF, Tepper RS, Gunst SJ. Mechanisms for the mechanical response of airway smooth muscle to length oscillation. J Appl Physiol 1997 Sep; 83(3):731–738.
- Plowman L, Edwards PH, Lauff DC, Berthon-Jones M, Sullivan CE. Tracheal smooth muscle responses to upper airway pressure in conscious dogs. J Appl Physiol 1990 Apr; 68(4):1555–1561.
- Fagevik Olsen M, Westerdahl E. Positive expiratory pressure in patients with chronic obstructive pulmonary disease—a systematic review. Respiration 2009; 77(1):110–118.
- Holanda MA, Fortaleza SC, Alves-de-Almeida M, Winkeler GF, Reis RC, Felix JH, Lima JW, Pereira ED. Continuous positive airway pressure effects on regional lung aeration in patients with COPD: a high-resolution CT scan study. Chest 2010 Aug; 138(2):305–314.