Abstract
Purpose: Early ambulation in the ventilated patient is gaining wider acceptance. We evaluated a new portable (1 lb), gas powered, volume ventilator designed for NIV via a proprietary nasal pillows interface (Breathe Technologies, CA). Methods: We developed a model to approximate a patient's nose, upper airway and trachea. The model was connected to a test lung (ASL5000, Ingmar Medical, Pittsburgh, PA) via 22 mm ID corrugated tubing. The nasal pillows were adjusted in the nares using a lanyard. The ASL was set to represent a normal patient, a COPD patient, and a patient with interstitial lung disease (ILD). The Breathe ventilator was set at delivered volumes of 100 mL, 150 mL, 200 mL, and 250 mL. Baseline data was also collected without the appliance connected. Delivered volume, inspired oxygen concentration (FIO2), inspiratory flow (V), and peak inspiratory pressure (PIP) were recorded for each breath. Data for 10 breaths were used to calculate the mean at each condition (± SD). Results: The Breathe volume ventilator delivered an augmented simulated patient tidal volume of 362 to 823 mL, augmenting the simulated patient's spontaneous volume by up to 459 mL, depending on ventilator settings and ASL lung conditions. Delivered FIO2 ranged from 0.36 to 0.45 and was also dependent on ventilator settings and ASL lung conditions. Conclusions: The PIP, delivered tidal volumes, and measured FIO2 support the hypothesis that this system can augment minute ventilation and supply supplemental oxygen in spontaneously breathing patients with a simple, non-invasive interface.
Introduction
Non-invasive ventilation (NIV) has changed the paradigm for treatment of patients with acute exacerbations of chronic obstructive pulmonary disease (COPD) and the long term management of patients with chronic neuromuscular disease (Citation1–5). As a result, research on the use of NIV in a number of other disease states continues to expand (Citation6–8).
Early ambulation of ventilated patients has also undergone a ‘rebirth’ in last few years and may represent another area where NIV may play a role (Citation9–13). NIV could also be used to facilitate ambulation in patients with chronic disease by reducing the respiratory workload and oxygen cost of breathing during rehabilitation, and those patients participating in activities of daily living. We evaluated a portable ventilation system for NIV designed to facilitate ambulation using a benchtop model of the upper airway and lungs.
Device description
The Non-Invasive Open Ventilation (NIOV™) system (Breathe Technologies, Irvine, CA) consists of a pneumatically powered, electronically controlled ventilator operating from a 50 psig gas source. The cannula style interface is held in place by a lanyard and nasal pillows are fitted into the nares and includes 2 venturis/air entrainment ports. The Breathe NIOV ventilator (Citation3 1/8″(h) × 1¼″(d) × 7 ½″(w), weight 1 lb) () provides patient triggered, volume breaths, which are time cycled. The inspiratory time is adjusted using a ‘percent delivery time’ control which bases inspiratory time on the total cycle time. All breaths are patient triggered based on a change in airway pressure. Sensitivity is adjustable from a setting of 0 to 9, which corresponds to negative pressures of approximately −0.01 to −0.34 cm H2O below baseline. Inspired oxygen (FIO2) delivered from the device is based on the input, typically at an FIO2 of 1.0. Gas from the ventilator is delivered to a nasal interface which includes two venturi ports for air entrainment (). As gas from the ventilator travels though the nozzles, room air can be entrained and increase the delivered tidal volume. Theoretically this entrainment may also add a small amount of humidity.
Figure 2. Components of the nasal interface. With each of the two nasal pillows there is a sense port, entrainment port, and nozzle.
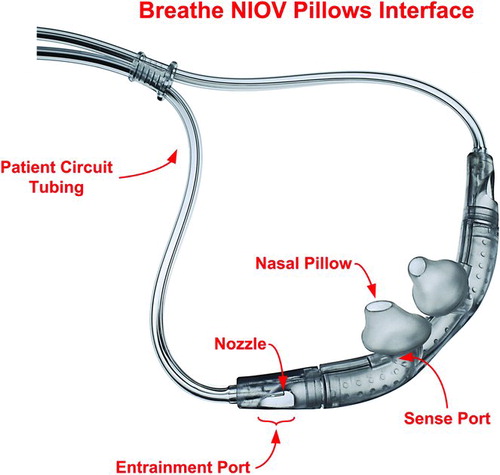
Although the ventilator produces a constant volume breath that is time-cycled, because of the entrainment ports, neither the volume nor pressure delivered to the patient is controlled. The ventilator has three password-protected volume settings that are titrated by the clinician to the patient's needs at varying activity levels. The patient can select from these three volume settings as needed to support their current activity.
Methods
A model of the nose and upper airway was constructed to approximate a patient's nose, upper airway, and trachea but was not physiologically precise (). The model was constructed based on the “Airway Larry” adult airway management training head. (http://www.enasco.com/product/LF03667U). The airway resistance of the nasal model was measured from the pressure drop across the device via an RT 200 analyzer (Timeter Instrument Corp., Lancaster, PA). The model was attached to a computerized test lung (ASL 5000, Ingmar Medical, Pittsburgh, PA) via standard 22 mm internal diameter (ID) tubing equivalent to the normal anatomic deadspace (150 mL). The test lung was set to simulate spontaneous breathing with lung conditions corresponding to normal lungs, a patient with chronic obstructive pulmonary disease (COPD) and a patient with interstitial lung disease (ILD). These characteristics are shown in .
Table 1. Test lung settings in spontaneous mode
Lung characteristics were altered in each condition as was the level of inspiratory effort by the test lung. The test lung was set to create a sinusoidal inspiratory flow profile. The ventilator was set at volumes of 100 mL, 150 mL, 200 mL, and 250 mL.
The percentage delivery time was set at 21% (the default) and the trigger sensitivity was set at the 4 (default) value. Baseline data was also collected using each of the lung conditions without the nasal model connected. The ASL 5000 was utilized to measure airway pressure, volume, and flow at a frequency of 512 Hz. FIO2 was measured continuously via the integral oxygen analyzer. The oxygen analyzer was calibrated to both room air and an FIO2 of 1.0. Delivered volume was determined by averaging the measured volume over one minute based on piston displacement of the test lung and reported as mean ± SD. We also calculated the difference between the delivered volume and the set ventilator volume to quantify the degree of air entrainment, based on the assumption that the ventilator volume settings were accurate. Peak flow and peak airway pressure are also presented as mean ± SD. Data from a minimum 10 breaths were used for the calculations.
Statistical analysis
Entrainment was expected to change with changes in set ventilator volume, inspiratory effort, and modifications in lung mechanics. We employed one-way ANOVA models to measure the differences between successive volume settings treated as categorical variables. We fit a separate model for each of the three lung conditions.
In addition, we employed linear regression analysis to measure the relationship between volume and combinations of compliance, resistance, ventilator setting, the interaction between compliance and ventilator setting, and the interaction between resistance and ventilator setting.Finally, we used a linear regression analysis to study the relationship between ventilator setting and volume. Separate models were fitted for each lung condition in order to be able to rank the strength of the relationship between those two variables.
Results
For each test lung setting, the FIO2 was lowest at the lowest set ventilator volume and increased with each subsequent ventilator volume setting. For all ventilator volume settings, FIO2 was greatest at the lung setting representing the ILD condition, and FIO2 had the greatest setting-to-setting increase with the COPD condition ().
Table 2. Mean baseline, ventilator, entrained, and total volume (mL) and mean FIO2 and peak inspiratory pressure (PIP) delivered under each condition.
The volume delivered to the test lung by the ventilator was greater than the test lung's spontaneous volume baseline for all test conditions. With each successive volume setting on the ventilator the volume delivered to the test lung increased. Volume increases of the test lung over baseline ranged from 25–458 mL, depending on the ventilator setting and test lung settings ( and ). Increasing the set ventilator volume resulted in a delivered volume to the test lung that was different from the sum of lung and ventilator volumes. This additional volume results from air entrainment (). However, at the 100 mL setting there was no entrainment and in some cases the volume delivered to the test lung was less than the sum of set ventilator volume and spontaneous breathing volume.
Entrainment was significantly greater at the 250-mL setting, thereby augmenting the delivered volume. The total volume was lowest in the COPD condition as compared to either of the other conditions. At normal lung conditions the largest entrained volume was 82% of ventilator volume (25% of total volume) at the 250 mL setting vs. 26% of ventilator volume (9% of total volume) for the COPD model at the same setting. The mean FIO2 was 0.42 ± 0.001 (range 0.36–0.45) and the mean PIP was 5.1 cm H2O (range 1.3–9.5). No instances of auto-triggering was observed with any of the lung models or ventilator settings.
shows the effects of successive increments of set ventilator volume. For all lung conditions, increasing set volume from 0 to 100 mL resulted in a statistically significant increase in delivered volume but did not demonstrate entrainment. In the COPD condition, a further 50 mL increase in set volume also resulted in decreased anticipated entrainment. However, all other increases in set volume (from 150 mL to 250 mL for the COPD condition and from 100 mL to 250 mL for the other conditions) led to increases in entrained volume. The largest entrainment volumes were associated with the highest set volume of 250 mL.
To further investigate the relationship among variables, we fitted a linear regression model in which volume (V) depends upon compliance (C), resistance (R), and ventilator setting (S) and the interactions between compliance and setting (C*S) and between resistance and setting (R*S).
After dropping the non-significant C*S effect, the fitted model was
with R2 = 0.92. This equation says that volume depends positively on C and S and negatively (inversely) on R. The negative effect of R is stronger at higher vent settings.
An alternative approach recognizes that each C/R combination is equivalent to a specific lung model. Each model results in a different relationship between ventilator setting (S) and volume (V). The healthy lung model exhibits the strongest relationship between S and V. Specifically, each 50 mL increase in S results in a 91.5 mL increase in V. For ILD, the relationship is weaker; a 50 mL increase in S is associated with an 85 mL increase in V. Finally, the COPD condition exhibits the weakest relationship; a 50-mL increase in S results in a 64.5 mL change in V ().
Examining the volume entrained/lost in , the relationship between the baseline volume and ventilator volume does not appear linear with increases in ventilator settings. shows that in fact there is a strong linear correlation between the baseline and ventilator volumes (R2 = 0.99). The weakest relationship between the two volumes was with the COPD lung model but there was still a strong linear correlation (R2 = 0.94).
Figure 5. Correlation between ventilator VT setting and anticipated entrained volume. Negative values represent total delivered volumes that were less than the sum of the ventilator VT setting and the lung model baseline VT.
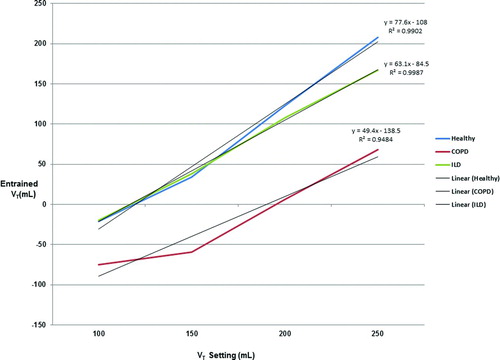
The measured resistance of the nasal model was 1.7 cm H2O/L/s at a flow of 40 L/min and 6.1 cm H2O/L/s at a flow of 80 L/min. These flow rates represent the lowest and highest flows generated by the ventilator at the lowest and highest VT settings.
Discussion
We found that the Breathe NIOV system was capable of augmenting tidal volume in a model of spontaneous breathing. The degree of tidal volume augmentation was proportional to the ventilator volume and compliance and inversely proportional to the resistance. The entrainment feature in the interface has an improved efficiency at higher tidal volumes. The average delivered FIO2 was approximately 0.43.
Positive pressure NIV dates back to the 1930s for use in the treatment of acute pulmonary edema (Citation14). Intermittent positive pressure breathing (IPPB) was widely used from the 1940s–1980s to deliver bronchodilators to COPD patients (Citation15) and was studied as a modality for treating respiratory failure (Citation16). In the 1960s NIV was used to treat patients with neuromuscular disease and with the incorporation of nasal and face masks to treat sleep apnea and muscular dystrophy from the 1980s until present (Citation17).
Numerous observational and randomized controlled trials have shown that NIV improves quality of life and exercise tolerance in chronic restrictive and obstructive pulmonary disease (Citation18–24).Ventilators suitable for NIV use have gotten smaller, lighter, and more portable over the past decade but arguably remain too bulky for someone with pulmonary disease to carry. One study found that in COPD patients, dyspnea did not improve when walking and carrying an NIV ventilator, due to the added weight (Citation25). The NIOV device in this bench study is small enough to clip onto a person's belt or be placed in a shoulder bag and provides supplemental ventilatory support and oxygen to the user.
The NIOV system was able to augment tidal volume delivered to the test lung at all settings tested. As set ventilator volume increased, the entrained volume increased further augmenting ventilation. The largest delivered volume (spontaneous volume + ventilator volume + entrained volume) was at the 250 mL setting.
The smaller volumes delivered with the COPD model compared to the Healthy and ILD models is not counterintuitive since downstream resistance has a negative effect on the rate of entrainment, resulting in lower than expected total volume delivery. With a set compliance of 125 cm H2O/L/s one would expect the volume to increase more across all settings with this model than with the other lung models. This would likely be the case if the airway resistance was the same with all three models. With the COPD model, the airway resistance was double that of the other models. This demonstrates that lung model resistance and the operating characteristics of the ventilator are the major determinants of the entrained volume.
For the evaluation, the NIOV device had a set inspiratory time of 21% of the total breath period with all settings, therefore an increase in delivered volume increased the inspiratory flow. At the 100 mL setting the inspiratory flow was 24 L/min and was 60 L/min at the 250-mL setting. Our data suggest that the higher driving pressure and flow facilitates entrainment. In the COPD model with higher airway resistance, entrainment was reduced and the delivered volume was less than the other models. The increase in downstream resistance results in back pressure which reduces the entrainment effectiveness of the venturi apparatus.
The NIOV device is small and lightweight but requires a 50 psig gas source necessitating the concurrent use of an oxygen cylinder. This diminishes the portability feature due to having to either carry a cylinder or having one in tow in a rolling carrier. However, most patients who would use this technology already use oxygen cylinders. Albeit better than having to manage a heavy ventilator, the required compressed gas source may limit mobility. Additionally, oxygen cylinders present a projectile hazard if dropped or mishandled. There is no humidification with the system and the entrainment of room air to add humidity may be insufficient is some cases. In a study with 18 subjects wearing the NIOV for 6 consecutive hours, the mean score was 4/5 on a Likert scale indicating that nasal dryness did not present a significant problem during the usage time (Citation26).
Clinical trials conducted using the NIOV system support the hypothesis that providing ambulatory ventilation in conjunction with supplemental oxygen can improve exercise tolerance and dyspnea. Hilling et al. (Citation27) demonstrated that exercise distances using NIOV increased in 25 out of 30 subjects with a mean improvement in 6 minute walk distance (6MWD) of 57 m (±54). In a follow up study of 32 subjects, a mean improvement in 6MWD distance of 36 (±34.1) meters was observed in subjects using NIOV (Citation28). In a third study, NIOV was evaluated in 18 subjects with severe COPD at 3 pulmonary rehabilitation centers (Citation26). Subjects reported experiencing less dyspnea, greater mobility, and improved exercise endurance compared to their current oxygen systems.
Limitations
There are several limitations to our methods. We used a lung model and while volume and FIO2 can be characterized, the model cannot sense a reduction in dyspnea or detect improved comfort. Patient studies are needed to determine utility of the device. We used a set of compliance and resistance characteristics based on disease states, which cannot represent the entire range in the actual target population. Additionally, linear resistance was used for the lung models and may not represent the true inspiratory and expiratory airway resistance of the different lung models. We did not measure humidity, which may be an important issue in patient use.
The nasal model used in this experiment was created to resemble the human nasal airway anatomy but was not physiologically precise. Nasal resistance varies from person to person but is reported to be approximately 3 cm H2O/L/s (Citation29). Determining actual resistance in the human nasal cavity and upper airway is difficult due to differences in individuals’ anatomy. The differences in airway resistance at various flow rates may have affected the rate of entrainment and therefore decreasing the total volume delivered to the test lung.
Conclusions
The addition of the Breathe NIOV device augmented tidal volume delivered to a spontaneously breathing model. The tidal volume is augmented both by the ventilator setting and the entrained volume. At higher volume settings and higher compliance/lower resistance, tidal volume is enhanced. The NIOV system results in ventilation of the model and stable FIO2 in the range of 0.36 to 0.45. This device may be useful for ambulation at home and pulmonary rehabilitation. Studies evaluating walking distance and patient comfort during exercise or ambulation need to be done.
Declaration of Interest Statement
Breathe Technologies provided research support funding to our institution for this evaluation and bench study. The authors have no other conflicts of interest to disclose. The authors are responsible for the content and the writing of this paper.
References
- Nava S, Hill N. Non-invasive ventilation in acute respiratory failure. Lancet 2009; 374(9685):250–259.
- Keenan SP, Mehta S. Noninvasive ventilation for patients presenting with acute respiratory failure: the randomized controlled trials. Respir Care 2009; 54(1):116–126.
- Benditt JO. Full-time noninvasive ventilation: possible and desirable. Respir Care 2006 Sep;51(9):1005–1012; discussion 1012–1015.
- Ambrosino N, Vagheggini G. Noninvasive positive pressure ventilation in the acute care setting: where are we? Eur Respir J 2008; 31(4):874–886.
- Flandreau G, Bourdin G, Leray V, Bayle F, Wallet F, Delannoy B, Management and long-term outcome of patients with chronic neuromuscular disease admitted to the intensive care unit for acute respiratory failure: a single-center retrospective study. Respir Care 2011; 56(7):953–960.
- Elliott MW. Domiciliary non-invasive ventilation in stable COPD? Thorax 2009; 64(7):553–556.
- Benditt JO. Novel uses of noninvasive ventilation. Respir Care 2009; 54(2):212–219.
- Theerakittikul T, Ricaurte B, Aboussouan LS. Noninvasive positive pressure ventilation for stable outpatients: CPAP and beyond. Cleve Clin J Med 2010;77(10):705–714.
- Bourdin G, Barbier J, Burle JF, Durante G, Passant S, Vincent B, The feasibility of early physical activity in intensive care unit patients: a prospective observational one-center study. Respir Care 2010; 55(4):400–407.
- Hall JB. Creating the animated intensive care unit. Crit Care Med. 2010;38(10 Suppl):S668–675.
- Kress JP. Clinical trials of early mobilization of critically ill patients. Crit Care Med 2009; 37(10 Suppl):S442–447.
- Bailey PP, Miller RR 3rd, Clemmer TP. Culture of early mobility in mechanically ventilated patients. Crit Care Med 2009; 37(10 Suppl):S429–435.
- Schweickert WD, Pohlman MC, Pohlman AS, Nigos C, Pawlik AJ, Esbrook CL, Early physical and occupational therapy in mechanically ventilated, critically ill patients: a randomised controlled trial. Lancet 2009; 373(9678):1874–1882.
- Barach AL, Martin J, Eckman M. Positive pressure respiration and its application to the treatment of acute pulmonary edema. Ann Intern Med 1938; 12:754–795.
- Motley HL, Lang LP, Gordon B. Use of intermittent positive pressure breathing combined with nebulization in pulmonary disease. Am J Med 1948; 5:853–856.
- Fraimow W, Cathcart R, Goodman E. The use of intermittent positive pressure breathing in the prevention of the carbon dioxide narcosis associated with oxygen therapy. Am Rev Respir Dis 1960; 81:815–822.
- Mehta S, Hill N. Noninvasive ventilation. Am J Respir Crit Care Med 2001; 163:540–577.
- van't Hul A, Gosselink R, Hollander P, Postmus P, Kwakkel G. Training with inspiratory pressure support in patients with severe COPD. Eur Respir J 2006; 27(1):65–72.
- Barakat S, Michele G, Nesme P, Nicole V, Guy A. Effect of a noninvasive ventilatory support during exercise of a program in pulmonary rehabilitation in patients with COPD. Int J Chron Obstruct Pulmon Dis 2007; 2(4):585–591.
- Toledo A, Borghi-Silva A, Sampaio LM, Ribeiro KP, Baldissera V, Costa D. The impact of noninvasive ventilation during the physical training in patients with moderate-to-severe chronic obstructive pulmonary disease (COPD). Clinics (Sao Paulo) 2007; 62(2):113–120.
- Borghi-Silva A, Mendes RG, Toledo AC, MalosaSampaio LM, da Silva TP, Kunikushita LN, Adjuncts to physical training of patients with severe COPD: oxygen or noninvasive ventilation? Respir Care 2010; 55(7):885–894.
- Vila B, Servera E, Marin J, Diaz J, Gimenez M, Komaroff E, Noninvasive ventilatory assistance during exercise for patients with kyphoscoliosis: a pilot study. Am J Phys Med Rehabil 2007; 86(8):672–677.
- Menadue C, Alison JA, Piper AJ, Wong KK, Hollier C, Ellis ER. High- and low-level pressure support during walking in people with severe kyphoscoliosis. Eur Respir J 2010; 36(2):370–378.
- Borel JC, Wuyam B, Chouri-Pontarollo N, Deschaux C, Levy P, Pepin JL. During exercise noninvasive ventilation in chronic restrictive respiratory failure. Respir Med 2008; 102(5):711–719.
- Dreher M, Doncheva E, Schwoerer A, Walterspacher S, Sonntag F, Kabitz HJ, Preserving oxygenation during walking in severe chronic obstructive pulmonary disease: noninvasive ventilation versus oxygen therapy. Respiration 2009; 78(2):154–160.
- McCabe, L, Cayou C, Hilling L, Kops R, Heron G, Morishige R. Use of a novel non-invasive open ventilation system during rest, activities of daily living, and exercise in patients with severe COPD. Abstract presented at American Thoracic Society International Conference; May 18–23, 2012; San Francisco, CA.
- Hilling L, Cayou C, Garvey C, Wondka T, Kops RS. Improved 6MWT distance with a highly portable non-invasive ventilator. Abstract presented at American Thoracic Society International Conference; 2010 May 14–19, New Orleans, LA.
- Garvey C, Hilling L, Cayou C, Escobar R, Heron G, McCabe L. Open, Noninvasive Ventilation Using a 1 lb Ventilator, Oxygen, and a Low Profile Mask Improves 6 MWT Distances in Advanced COPD. Abstract presented at American Thoracic Society International Conference; May 13–18, 2011, Denver, CO.
- Nathan RA, Eccles R, Howarth PH, Steinsvag SK, Togias A. Objective monitoring of nasal patency and nasal physiology in rhinitis. J Allergy Clin Immunol 2005; 115(5):S442–S459.