Abstract
Plants may have the ability to modulate immune responses. In the present study, the effects of three plants belonging to Labiatae family, each traditionally used for the treatments of infections and inflammatory diseases, as well as the role of thymol (as one the major components of these plants), were investigated for their potential to affect the activation of lymphocytes. Four organic extracts of Thymus vulgaris and two other plants (i.e., T. daenensis and Zataria multiflora) were prepared. The effect of the extracts on mitogen (PHA)-stimulated peripheral blood lymphocytes was determined using a cell proliferation assay. The hexane extracts obtained from the three plants showed the strongest inhibitory effects on PHA-induced proliferation. Use of preparative thin layer and gas chromatographies in conjunction with the proliferation assay confirmed that thymol was the major component responsible for the observed effects from the three plants. Thymol inhibited inducible lymphocyte proliferation in a concentration-dependent manner, with reductions ranging from 62.8% at 50 µg/ml to 89.8% at 200 µg/ml (> 0.1 µg/ml (p < 0.01). Flow cytometric analysis using propidium iodide staining showed that the inhibitory effect of thymol at 200 µg/ml was due to a cytotoxic activity. In conclusion, the three Labiatae plants studied here each showed immunosuppressive effects against lymphocytes and it was most likely that thymol was the compound in these plants responsible for this effect.
Introduction
The family Labiatae is generally known for its various pharmacological effects (Ali et al., Citation2000; van Vuuren et al., Citation2009; Amirghofran, Citation2010; Ganjewala and Luthra, Citation2010; Ziaei et al., Citation2011). Thymus vulgaris L. (Thyme), a member of Labiatae, is a small shrubby plant native to the Mediterranean region that possesses a strong spicy taste and odor. As “Avishan” (in Persian), it is widely used in traditional medicine, including as an: expectorant, anti-broncholitic, anti-spasmodic, carminative, or a diuretic (Behnia et al., Citation2008; Undeger et al., Citation2009); its essential oil exhibits anti-bacterial, -fungal, and -oxidant properties (Bukovská et al., Citation2007; Sokovic et al., Citation2010). Another species, T. daenensis, is endemic in many parts of Iran; as “Avishan-e Denaii”, it too is used to treat infectious diseases. One other genus of the Labiatae family is the Zataria plants that are widely distributed in Iran, Afghanistan, and Pakistan (van Vuuren et al., Citation2009). Z. multiflora Boiss (“Avishan-e Shirazi”) is used for medicinal purposes including as an anesthetic, anti-septic, or anti-spasmodic (Zargari Citation1990, Nakhai et al., Citation2007). It is also used to treat pre-menstrual pain, chronic catharsis, asthma, jaundice, sore throat, neuralgia, and edema (Ramezani et al., Citation2004).
The abilities of these herbs to impart pharmaco-logic/microbiologic outcomes are mostly due to a presence of phenolic compounds such as thymol and carvacrol, as well as to hydro-carbons such as terpinene and cymene (Lambert et al., Citation2001; Santoro et al., Citation2007; Palaniappan and Holley Citation2010). Studies have shown that other major components of thyme (as well as of T. daenensis) are tannins, flavonoids, saponins, and triterpenic acids (Behnia et al., Citation2008; Mojab et al., Citation2008). Biochemical analyses have revealed that many of these agents are also major components of Z. multiflora plants (Saleem et al., Citation2004; Mahmoudabadi et al., Citation2007; Saei-Dehkordi et al., Citation2010; Hadian et al., Citation2011).
As noted, all of these Labiatae family plants display high similarity in major constituents and a wide shared spectrum of pharmacological properties. Clearly, that these plants act to stem bacterial/fungal growth suggests there are agents that are directly bactericidal/fungicidal (Bukovská et al., Citation2007; Mojab et al., Citation2008). However, there is some evidence indicating that these plants may also possess constituents with abilities to modulate immune responses as well. A considerable stimulation of leukopoiesis and an elevation of thrombocyte counts in blood have been observed following thyme treatment (von Ardenne et al., Citation1998). Anti-inflammatory effects against acute and chronic inflammation in the rat have also been reported with Z. multiflora (Hosseinzadeh et al., Citation2000). Despite these studies, to the best of our knowledge, no specific data relating to the potential effects of these plants on the growth and activity of lymphocytes (the main cells of the immune response) per se and, further, the identity of those components that could be responsible for said effects, has been reported.
In this regard, we hypothesized that thymol—as a main component in each of these plants—might be one compound that could affect lymphocyte function and so be responsible for the above mentioned immunomodulatory effects. Evidence indicating that the anti-inflammatory activity of thymol is mediated in part by its inhibitory effect on the release of elastase by neutrophils (Braga et al., Citation2006) and on cytokine responses by macrophages (Ocaña-Fuentes et al., Citation2010) suggests to us that like these two cell types, lymphocytes will likely be affected by this agent. The studies here sought to prove that these plants could alter the function of lymphocytes and that of the myriad constituents in each, thymol was the most likely causative agent.
Materials and methods
Preparation of extracts
Plants were collected from Fars province and identified by Dr. Ahmadreza Khosravi in the Biology Department at Shiraz University. Voucher specimens were deposited in the herbarium of Shiraz University. Aerial parts of the plants were air-dried, powdered, and defatted with petroleum ether for 4 h. The defatted materials were then extracted with methanol for 48 h at room temperature. The resultant extract was filtered and concentrated under reduced pressure. Extract yield (w/w) was 5.0% for T. vulgaris, 6.0% for Z. multiflora, and 5.8% for T. daenensis. The respective methanolic extracts were subsequently partitioned into hexane, ethyl acetate, and n-butanol. The dried extracts were later dissolved in dimethyl sulfoxide (DMSO, Sigma, St Louis, MO) and placed in RPMI 1640 (Sigma) medium to yield corresponding 2 mg/ml solutions.
Cell line and culture
The Jurkat cell line (a T-cell leukemia) prepared from the cell bank of the Pasteur Institute of Iran (IPI; Tehran, Iran) was seeded into flat-bottom 96-well plates and then maintained in complete medium (RPMI 1640 containing 10% fetal calf serum [Gibco-BRL, Frederick, MD], 100 U penicillin/ml, and 100 μg streptomycin/ml) under standard culture conditions (37°C, 95% humidified air, and 5% CO2). The cells were fed until confluent (i.e., reached 2 × 106/well) and then were treated with 0.01, 0.1, 10, 50, 100, or 200 µg thymol/ml for 48 h. The thymol was purchased from Merck (Darmstadt, Germany). Negative control cells were treated with DMSO at a final concentration equal to that delivered to thymol-treated cells (maximum, 1 µl/ml for test wells containing 200 µg/ml thymol).
In vitro cell proliferation assay
Peripheral blood lymphocytes (PBL) from at least five healthy male individuals (25–35 years-old, each of who provided informed consent) were separated by gradient centrifugation with Ficoll-Hypaque (Lymphoprep, Oslo, Norway). The effect of the extracts (0.01, 0.1, 10, 50, 100, or 200 µg/ml) and thymol (0.1–200 µg/ml) on the mitogen-induced PBL (1 × 105/well) and Jurkat cells (2.5 × 104/well) were determined using a 5-bromo-2′-deoxyuridine (BrdU) incorpora-tion assay (Roche Diagnostics, Penzberg, Germany) as described previously (Gharagozloo and Amirghofran, Citation2007). Phytohemagglutinin (PHA; 1:1750 dilution, Fluka Chemie GmbH, Steinheim, Germany) was used as the mitogen added to the cultures.
In each assay, cells were cultured for 48 h in the presence or absence of thymol or the extracts. After labeling with BrdU, the cells were incubated with anti-BrdU monoclonal antibody and then optical density (OD) was measured at 450 nm and using a 630 nm reference filter. The inhibition percentage was calculated as: 100 − [(OD treated cells/OD corresponding control) × 100]. The control was extract- (or thymol)-untreated cells stimulated with PHA and containing DMSO at a final concentration equal to that in the test wells (maximum, 1 µl/ml for test wells having 200 µg/ml thymol or extract). Dexamethasone (0.1, 1, 10, 50, 100, or 200 µg/ml) (Iran Daru Co., Tehran) was used as a positive control. All experiments were performed using triplicate wells and each experiment was performed at least three times.
MTT assay
Assessment of Jurkat cell growth was also examined using a 3-(4,5-dimethylthiazol-2-yl)-2,5-diphenyltetrazolium bromide (MTT) assay as previously described (Gharagozloo and Amirghofran, Citation2007). Briefly, cells (2.5 × 104/well) were seeded in 96-well plates and treated with 0.01–200 µg extract/ml for 48 h. Thereafter, 10 µl of MTT (5 mg/ml, Sigma) was added to each well and the cells incubated for an additional 4 h at 37°C, followed by treatment with DMSO. Absorbance in each well was then assessed spectrophotometrically at dual wavelengths of 570 and 630 nm on a microplate reader (Pharmacia). The inhibition percentage was calculated according to the same formula as in the BrdU assay (see above). Since activation of lymphocytes could enhance reduction of MTT dye in mitochondria and interfere with quantification of the effects of the components, the MTT assay was only used for testing proliferation of the Jurkat cells. All experiments were performed using triplicate wells and each experiment was performed at least three times.
Bioassay guided-fractionation and isolation of the active ingredient
A pilot study revealed that among the extracts of the plants, hexane extracts were more effective on the cells; thus, these latter materials were chromatographed over a silica gel (70–230 mesh, 500 g) using a step-gradient hexane/ether solvent system (100:0, 90:10, 75:25, 50:50, and then 0:100 v/v) to yield five fractions. Each fraction was tested for inhibitory effect on PBL proliferation via the BrdU assay. The active compound was subsequently isolated from the bioactive fraction by preparative thin layer chromatography (TLC) using hexane/ethyl acetate (90:10) as the mobile phase. Because it was suspected that the active compound might be thymol, the isolated compound and thymol standard (Merck) were subjected to gas chromatography using an Agilent 6890N chromatograph (FID) fitted with an HP-5 column (30 m × 0.25 mm; 0.25 µm film thickness) (Agilent Technologies, Böblin-gen, Germany). The oven temperature was automatically increased from 60 to 240°C at a rate of 4°C/min; the injector and detector temperatures were 240 and 250°C, respectively. Helium was used as the carrier gas (at a flow rate = 0.9 ml/min).
Propidium iodide (PI) staining for viability
Mitogen-activated PBL (1 × 105/well) and Jurkat cells (2.5 × 104/ml) were cultured in 96-well culture plates and treated with various concentrations of thymol for 48 h. Cells were then harvested and washed by phosphate-buffered saline (PBS) and re-suspended in 500 µl PBS containing 1 µl PI/ml. Samples were kept in PI staining solution at 4°C protected from light until analysis on a flow cytometer (FACSCalibur, Becton Dickinson, San Jose, CA). A minimum of 10,000 events per test population was analyzed. Ultimately, the viability percentage of cells was determined using Windows Multiple Document Interface software (WinMDI, La Jolla, CA).
Statistical analysis
The data were presented as mean (±SD) using SPSS software (Abaus Concepts, Berkeley, CA) and the Student’s t-test. A p value < 0.05 was considered significant.
Results
Effects of the extracts on the lymphocyte proliferation
The effects of the prepared extracts on the proliferation of PBL were evaluated using a BrdU incorporation assay. All the T. vulgaris extracts at concentrations of >50 µg/ml caused significant (p < 0.05) inhibition of PHA-induced PBL proliferation (). The hexane extract was more inhibitory against the lymphocytes (IC50 = 95.6 µg/ml) than the others. At higher concentrations, different extracts of T. daenensis showed inhibitory effects on lymphocyte proliferation. The strongest inhibitory effect was obatined with the hexane extract (IC50 = 53.3 µg/ml). With Z. multiflora, an inhibitory effect on cell growth was seen at concentrations of >10 µg/ml with all its extracts (p < 0.001). The most-inhibitory effect was demonstrated with the hexane (IC50 = 95.4 µg/ml) followed by the butanolic extract (IC50 = 99.6 µg/ml). Of note, a slight increase in lymphocyte proliferation at 0.01 µg hexane extract/ml was detected for each of the three plants (% inhibition,−21.2 to − 7.8, p < 0.04).
Figure 1. Effect of different extracts of Thymus vulgaris, T. daenensis, and Zataria multiflora on proliferation of mitogen-treated peripheral blood lymphocytes (PBL). Results are expressed as mean (±SD) inhibition of PBL proliferation in the presence of 0.01–200 µg extract/ml as compared to the proliferation of controls—as assessed in BrdU incorporation assays. Controls were extract-untreated cells stimulated with PHA and containing DMSO at a final level equal to that in the test wells (maximum, 1 µl DMSO/ml for test wells containing 200 µg extract/ml). Data shown represent three independent experiments, each performed in triplicate.
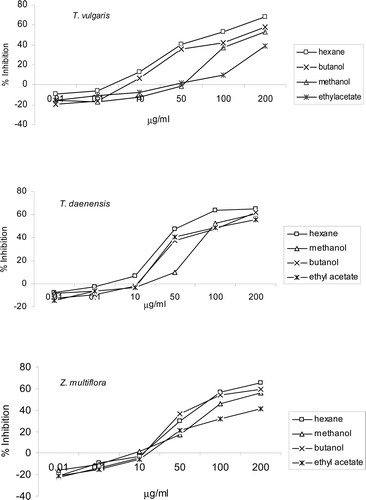
Effects of the fractions obtained from the hexane extracts of the plants
The hexane extract of each plant was the most effective inhibitor of lymphocyte activation. These extracts were further fractionated on silica gels by column chromatography and then tested for the presence of the active compound by TLC using hexane/ethyl acetate as the mobile phase. As shown in the , among all the fractions obtained from the T. daenensis hexane extract, the second fraction (i.e., 90:10 hexane/ether) showed the most-inhibitory effect on the lymphocytes (IC50 = 49.6 µg/ml). With Z. multiflora, a strong inhibition of lymphocyte growth was obtained with the same fraction (IC50 = 26.01 µg/ml). This 10% ether fraction of the hexane extract of T. vulgaris was also the most effective with in inhibiting lymphocyte proliferation (IC50 = 45.2 µg/ml). This fraction was subjected for further purification to isolate the active compound(s) responsible for these inhibitory effects.
Table 1. Effect of different fractions of the plants on the growth of peripheral blood lymphocytes using BrdU incorporation assay.
Identification of the active compound
A typical chromatogram of the isolated compound from T. daenensis is shown in . The retention times (RT) of the isolated active compound from T. daenensis, Z. multiflora, and T. vulgaris were 25.25, 25.45, and 25.32 min, respectively. In comparison, the RT for standard thymol was 24.97 min. By means of mass spectral analysis, an m/z = 150 was found for each of the isolated active compound (data not shown); standard thymol has an m/z value of 150.
Effects of thymol on cell proliferation
Both freshly isolated PBL and a T-cell leukemia Jurkat cells were exposed to increasing amounts of thymol for 48 hr prior to performing a BrdU incorporation assay. PBL proliferation was inhibited by different concentrations of thymol ranging from 62.82 (±7.4)% at 50 µg/ml to 89.8 (±5.7)% at 200 µg/ml (p < 0.001). This effect of thymol on the PBL was compared with the same effect of dexamethazone. As shown in , both compounds reduced lymphocyte proliferation in a concentration-dependent manner (p < 0.03) with an IC50 value of 16.9 (±3.5) µg thymol/ml and 1.05 (±0.5) µg dexamethasone/ml. The IC50 value of thymol here was less than that of the whole extract of the plants (e.g., 137.4 µg/ml for T. vulgaris, data not shown), and less than that for the hexane extract (e.g., 95.6 µg/ml for T. vulgaris). Thymol also significantly inhibited DNA synthesis in Jurkat cells at concentrations >0.1 μg/ml (p < 0.01; as indicated in both the MTT and BrdU assays—).
Figure 3. Thymol inhibitory effects on mitogen-induced proliferation of peripheral blood lymphocytes (PBL) as compared to those caused by dexamethaxone (Dexa). Results are expressed as the mean (±SD) inhibition of cell proliferation in the presence of 0.1–200 µg/ml thymol or dexamethasone as compared to the proliferation of controls - as assessed in BrdU incorporation assays. Control was dexamethasone- (or thymol)-untreated cells stimulated with PHA and containing DMSO at a final level equal to that in the test wells (maximum, 1 µl DMSO/ml for test wells containing 200 µg/ml dexamethasone or thymol). Dexamethasone at all concentrations significantly decreased lymphocyte proliferation (p < 0.03). *Thymol value significantly different from control at p < 0.006. Data shown represent three independent experiments, each performed in triplicate.
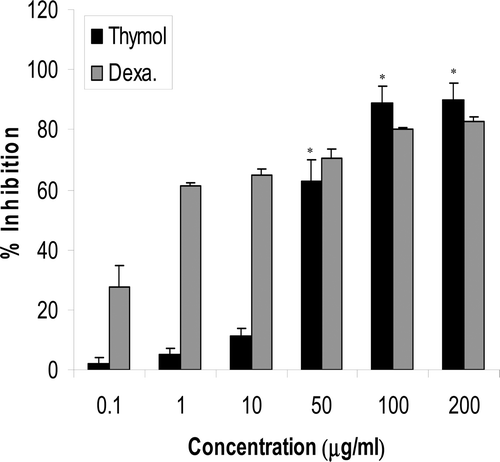
Figure 4. Effects of different concentrations of thymol on the proliferation of Jurkat cells as measured by BrdU (black) and MTT (gray) assays. Results are expressed as the mean of Jurkat cell growth inhibition in the presence of 0.01–200 µg thymol/ml as compared to the proliferation of controls. Controls were thymol-untreated cells containing DMSO at a final level equal to that in the test wells (maximum, 1 µl DMSO/ml for test wells containing 200 µg thymol/ml). Thymol at >0.1 µg/ml significantly decreased the growth of cells as determined with both methods (p < 0.01). Data shown represent three independent experiments, each performed in triplicate.
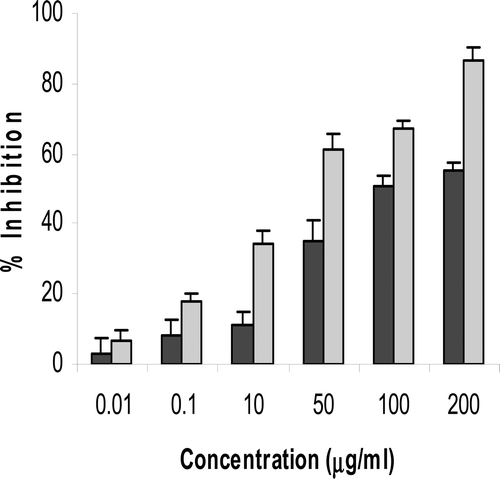
Effects of thymol on cell viability measured by PI staining
The viability of PBL and Jurkat cells after 48-h treatment with thymol was analyzed by flow cytometry. As shown in and , 200 µg thymol/ml significantly decreased the viability of the PBL and Jurkat cells. At this concentration, viability was 24.6 (± 7.0) % for PBL and 46.6 (±7.9)% for the leukemia cells (p < 0.01).
Figure 5. Determination of thymol cytotoxicity against peripheral blood lymphocytes (PBL) and Jurkat cells via propidium iodide (PI) staining. Flow cytometry data was analyzed by gating cell populations according to PI log fluorescence (y-axis) vs. forward side scatter (x-axis). (A) The mean percentage of viable Jurkat and peripheral blood lymphocytes (PBL) treated 48 h with different thymol concentrations. Data shown represent three independent experiments, each performed in triplicate. Controls were thymol-untreated PBL (i.e., 0 µg/ml) stimulated with PHA and thymol-untreated Jurkat cells, both containing DMSO at a final concentration equal to that in the test wells (maximum, 1 µl DMSO/ml for test wells containing 200 µg thymol/ml). *Value significantly different from respective controls at p < 0.05. (B) Histograms of PI uptake. Selected histograms indicate patterns of PBL and Jurkat cells treated with varying thymol concentrations. M1; Viable cells, M2: Dead Cells.
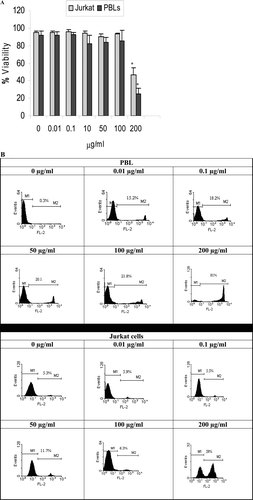
Discussion
In the present study, the effects of three important plants from the family Labiatae — locally named “Avishan” — on the growth and proliferation of human lymphocytes was investigated. At the first step, four extracts of the plants were prepared, among which the hexane extract of all the plants showed the most-inhibitory effect. After fractionation of the hexane extracts, it was appeared that a 10% ether fraction had the strongest inhibitory effect.
Preparative TLC, gas chromatography, and PBL proliferation assays were used to confirm that thymol: (A) had an IC50 comparable to that of the known immuno-inhibitory drug dexamethasone; and, (B) was one of the components in each of the three plants responsible for their immunomodulatory effects. The inhibitory effect of thymol on the growth of Jurkat cells was confirmed in both MTT and BrdU assays. As Jurkat cells are considered to be similar to resting T-lymphocytes, the growth-inhibitory response to thymol by these cells could be considered evidence of the immunoinhibitory effect of thymol and its capability to inhibit cell growth of peripheral blood mononuclear cells.
In order to determine whether this inhibitory effect of thymol on PBL and Jurkat cells was either due to a cytotoxic effect or a decrease in cell proliferative capacity, PI exclusion was used to distinguish dead cells. The results of studies here showed that thymol had no significant cytotoxic effects at <200 µg/ml. This indicated that the observed reduced proliferation of the cells was most likely due to an effect of this compound on the cells ability to respond to mitogen and/or cellular mechanisms involved in normal proliferation/division/etc.
The anti-inflammatory activity of thymol has been previously shown through its inhibitory effect on release of human neutrophil elastase (Braga et al., Citation2006). The immunomodulatory effects of the plants studied here have also been reported in a few previous investigations. For example, extracts of Z. multiflora have known anti-inflammatory activities against xylene-induced ear edema (Hosseinzadeh et al., Citation2000) as well as against acute inflammation induced by acetic acid. With respect to chronic inflammation, Z. multiflora has been shown to result in significant anti-inflammatory activity as well (Hosseinzadeh et al., Citation2000). Moreover, the benefits of Z. multiflora in experimental models of mouse inflammatory bowel disease have been reported (Nakhai et al., Citation2007). Similarly, T. vulgaris has been shown to stimulate leukopoiesis and elevate thrombocyte counts in the blood (von Ardenne et al., Citation1998). The stimulatory effect of the hexane extract of the plants on lymphocyte proliferation at low concentration found in our study may be in line with this particular set of observations. Treatment of colitic animals with a combination of thyme and oregano oils resulted in decreased levels of mRNA for several pro-inflammatory cytokines including interleukin (IL)-1β, IL-6, granulocyte-macrophage–colony stimulating factor (GM-CSF), and tumor necrosis factor (TNF)-α (Bukovská et al., Citation2007). When these essential oils were applied to mice with trinitrobenzene sulphonic acid-induced colitis, it resulted in a decrease in host mortality rate, accelerated body weight gain recovery, and reduced the levels of macroscopic damage within the colon tissues. In particular, two fractions of an oregano (Origanum vulgare) extract (containing trans-sabinene hydrate, thymol, and carvacrol as the main components) displayed strong anti-inflammatory properties, based on cytokine responses by treated THP-1 macrophages (Ocaña-Fuentes et al., Citation2010).
Lymphocyte proliferation is a critical event leading to the initiation and development of inflammation. In considering the inhibitory effects of thymol observed in the present study, it is reasonable to assume that the anti-inflammatory effects of these three plants (T. vulgaris, T. daenensis, and Z. multiflora) belonging to Labiatae family may be related, in part, to the effect of this compound. It is likely that thymol exerts these anti-inflammatory effects via an influence on nuclear factor-κB (NF-κB), a transcription factor that can activate expression of genes involved in immune and inflammation responses, including pro-inflammatory cytokines (Bollrath and Greten, Citation2009; Imanifooladib et al., 2010). Further studies are needed to clarify more precisely the role of this compound and its precise mechanism of action on/in the immune system.
Conclusions
The results here showed that extracts of T. vulgaris, T. daenensis and Z. multiflora had immunomodulatory effects on the proliferation of lymphocytes and that thymol was one of the responsible compounds for the inhibitory effect of the extracts. Due to the effect of this agent on the lymphocytes and the Jurkat cell line, thymol deserves to be investigated for other possible capacities to modulate immune responses.
Acknowledgements
This work was supported by grant 3036 from Shiraz University of Medical Sciences.
Declaration of interest
The authors report no conflicts of interest. The Authors are alone responsible for the content and writing of the paper.
References
- Ali, M. S., Saleem, M., Ali, Z., and Ahmad, V. U. 2000. Chemistry of Zataria multiflora (Lamiaceae). Phytochemistry 55:933–936.
- Amirghofran, Z. 2010. Medicinal plants as immunosuppressive agents in traditional Iranian medicine. Iran J Immunol 7:65–73.
- Behnia, M., Haghighi, A., Komeylizadeh, H., Tabaei, S. J., and Abadi, A. 2008. Inhibitory effects of Iranian Thymus vulgaris extracts on in vitro growth of Entamoeba histolytica. Korean J Parasitol 46:153–156.
- Bollrath, J., and Greten, F. R. 2009. IKK/NF-kappaB and STAT3 pathways: central signalling hubs in inflammation-mediated tumor promotion and metastasis. EMBO Rep 10:1314–1319.
- Braga, P. C., Dal Sasso, M., Culici, M., Bianchi, T., Bordoni, L., and Marabini, L. 2006. Anti-inflammatory activity of thymol: Inhibitory effect on the release of human neutrophil elastase. Pharmacology 77:130–136.
- Bukovská, A., Cikos, S., Juhás, S., Il’ková, G., Rehák, P., and Koppel, J. 2007. Effects of a combination of thyme and oregano essential oils on TNBS-induced colitis in mice. Mediators Inflamm 2007:23296.
- Ganjewala, D., and Luthra, R. 2010. Essential oil biosynthesis and regulation in the genus Cymbopogon. Nat Prod Commun 5:163–172.
- Gharagozloo, M. and Amirghofran, Z. 2007. Effects of silymarin on the spontaneous proliferation and cell cycle of human peripheral blood leukemia T-cells. J Cancer Res Clin Oncol 133:525–532.
- Hadian, J. Ebrahimi, S. N., Mirjalili, M. H., Azizi, A., Ranjbar, H. and Friedt, W. 2011. Chemical and genetic diversity of Zataria multiflora Boiss. accessions growing wild in Iran. Chem Biodivers 8:176–188.
- Hosseinzadeh, H., Ramezani, M. and Salmani, G. 2000. Antinociceptive, anti-inflammatory, and acute toxicity effects of Zataria multiflora Boiss extracts in mice and rats. J Ethnopharmacol 73:379–385.
- Imanifooladi, A. A., Yazdani, S. and Nourani, M. R. 2010. The role of nuclear factor-κB in inflammatory lung disease. Inflamm Allergy Drug Targets 9:197–205.
- Lambert, R. J., Skandamis, P. N., Coote, P. J. and Nychas, G. J. 2001. A study of the minimum inhibitory concentration and mode of action of oregano essential oil, thymol and carvacrol. J Appl Microbiol 91:453–462.
- Mahmoudabadi, A. Z., Dabbagh, M. A. and Fouladi, Z. 2007. In vitro anti-candida activity of Zataria multiflora Boiss. Evid Based Complement Alternat Med 4:351–353.
- Mojab, F., Poursaeed, M., Mehrgan, H. and Pakdaman, S. 2008. Anti-bacterial activity of Thymus daenensis methanolic extract. Pak J Pharm Sci 21:210–213.
- Nakhai, L. A., Mohammadirad, A., Yasa, N., Minaie, B., Nikfar, S., Ghazanfari, G., Zamani, M. J., Dehghan, G., Jamshidi, H., Boushehri, V. S., Khorasani, R. and Abdollahi, M. 2007. Benefits of Zataria multiflora Boiss in experimental model of mouse inflammatory bowel disease. Evid Based Complement Alternat Med 4:43–50.
- Ocaña-Fuentes, A., Arranz-Gutiérrez, E., Señorans, F. J. and Reglero, G. 2010. Supercritical fluid extraction of oregano (Origanum vulgare) essentials oils: Anti-inflammatory properties based on cytokine response on THP-1 macrophages. Food Chem Toxicol 48:1568–1575.
- Palaniappan, K. and Holley, R. A. 2010. Use of natural antimicrobials to increase antibiotic susceptibility of drug resistant bacteria. Int J Food Microbiol 140:164–168.
- Ramezani, M., Hosseinzadeh, H. and Samizadeh, S. 2004. Antinociceptive effects of Zataria multiflora Boiss fractions in mice. J Ethnopharmacol 91:167–170.
- Saei-Dehkordi, S. S., Tajik, H., Moradi, M. and Khalighi-Sigaroodi, F. 2010. Chemical composition of essential oils in Zataria multiflora Boiss. from different parts of Iran and their radical scavenging and antimicrobial activity. Food Chem Toxicol 48:1562–1567.
- Saleem, M., Nazli, R., Afza, N., Sami, A. and Ali, M. S. 2004. Biological significance of essential oil of Zataria multiflora boiss. Nat Prod Res 18:493–497.
- Santoro, G. F., das Graças Cardoso, M., Guimarães, L. G., Salgado, A. P., Menna-Barreto, R. F. and Soares, M. J. 2007. Effect of oregano (Origanum vulgare L.) and thyme (Thymus vulgaris L.) essential oils on Trypanosoma cruzi (Protozoa: Kinetoplastida) growth and ultrastructure. Parasitol Res 100:783–790.
- Sokovic, M., Glamoclija, J., Marin, P. D., Brkic, D. and van Griensven, L. J. 2010. Anti-bacterial effects of the essential oils of commonly consumed medicinal herbs using an in vitro model. Molecules 15:7532–7546.
- Undeger, U., Basaran, A., Degen, G. H. and Basaran, N. 2009. Anti-oxidant activities of major thyme ingredients and lack of (oxidative) DNA damage in V79 Chinese hamster lung fibroblast cells at low levels of carvacrol and thymol. Food Chem Toxicol 47:2037–2043.
- von Ardenne, M., and Reitnauer, P. G. 1998. The elevation of the leukocyte and thrombocyte counts produced by a thyme extract in the peripheral blood as compared to that caused by 2-cyanoethylurea. Pharmazie 36:703–705.
- van Vuuren, S. F., Suliman, S. and Viljoen, A. M. 2009. The anti-microbial activity of four commercial essential oils in combination with conventional antimicrobials. Lett Appl Microbiol 48:440–446.
- Zargari, A. (Ed.) 1990. Medicinal Plants. Volume 4. Tehran, Iran: Tehran University Press, pp. 1–57.
- Ziaei, A., Ramezani, M., Wright, L., Paetz, C., Schneider, B. and Amirghofran, Z. 2011. Identification of spathulenol in Salvia mirzayanii and the immunomodulatory effects. Phytother Res 25:557–562.