Abstract
Triclosan is an antimicrobial chemical commonly used occupationally and by the general public. Using select immune function assays, the purpose of these studies was to evaluate the immunotoxicity of triclosan following dermal exposure using a murine model. Triclosan was not identified to be a sensitizer in the murine local lymph node assay (LLNA) when tested at concentrations ranging from 0.75–3.0%. Following a 28-day exposure, triclosan produced a significant increase in liver weight at concentrations of ≥ 1.5%. Exposure to the high dose (3.0%) also produced a significant increase in spleen weights and number of platelets. The absolute number of B-cells, T-cells, dendritic cells and NK cells were significantly increased in the skin draining lymph node, but not the spleen. An increase in the frequency of dendritic cells was also observed in the lymph node following exposure to 3.0% triclosan. The IgM antibody response to sheep red blood cells (SRBC) was significantly increased at 0.75% – but not at the higher concentrations – in the spleen and serum. These results demonstrate that dermal exposure to triclosan induces stimulation of the immune system in a murine model and raise concerns about potential human exposure.
Introduction
Triclosan (2,4,4′-trichloro-2′-hydroxydiphenyl ether) is a large production volume anti-microbial chemical commonly used occupationally and by the general public (FDA, Citation2008). Triclosan was originally introduced in the healthcare setting over 40 years ago for use in clinical applications and as an antiseptic and hand sanitizer (Jones et al., Citation2000; Ming et al., Citation2007). While there is evidence for the antimicrobial efficacy of triclosan in clinical applications, its current use in consumer products is questioned (Jones et al., Citation2000; Tan et al., Citation2002). Triclosan is used in consumer products including antibacterial soaps and toothpastes in concentrations ranging from 0.03–1.0% (Glaser, Citation2004). In addition it is also used as a preservative, fungicide and biocide in household cleaning products and is infused into other household items (Glaser, Citation2004).
Due to its widespread use there is the potential for exposure of workers and the general public to triclosan through dermal or oral exposure (Moss et al., Citation2000) and it has been estimated that a total of 188 670 employees in 16 different industries were potentially exposed to triclosan (www.cdc.gov/noes). A recent study examining triclosan exposure in healthcare workers found significantly higher urinary triclosan levels in workers who used triclosan-containing soaps (255–258 ng/ml) compared to those who did not (8.6–68.5 ng/ml) (MacIsaac et al., Citation2014). Triclosan has been shown to undergo dermal absorption in both rodent and to a lesser degree human skin, affording it the potential to interact with immune cells in the dermis as well as systemically (Black et al., Citation1975; Kanetoshi et al., Citation1992). A study conducted by Kanetoshi et al. (Citation1992) applied [3H]-triclosan to mouse skin and detected maximum systemic levels 12–18 h post exposure, with the greatest concentration in the gall bladder, liver, body fat, lungs, kidneys, blood, heart, testes, spleen and brain. A more recent study by Fang et al. (Citation2014) investigated the toxicokinetic properties of triclosan ([14C(U)]-triclosan) absorption in B6C3F1 mouse skin following dermal application. Maximum absorption was obtained at ≈12 h after dosing and radioactivity appeared in the excreta and in all tissues examined including liver and lung, with the highest levels in the gall bladder and the lowest levels in the brain.
Triclosan is environmentally persistent and has also been found in drinking water, surface water, wastewater, and in environmental sediment (Lindstrom et al., Citation2002). Given the prevalence of triclosan it is not surprising that measurable levels have been detected in the majority of individuals examined to date (Adolfsson-Erici et al., Citation2002; Calafat et al., Citation2008; Hines et al., Citation2014; Pycke et al., Citation2014; Sandborgh-Englund et al., Citation2006). In 1997, the Food and Drug Administration (FDA) reviewed extensive effectiveness data on triclosan in toothpaste and found that triclosan in this product was effective in preventing gingivitis (Gunsolley, Citation2006). However, other than for clinical applications and in toothpaste, the FDA has not concluded that the addition of triclosan in antibacterial soaps and body washes provides any benefit over washing with regular soap and water and, therefore, does not provide an extra benefit to health.
There are limited studies evaluating the toxicity of triclosan; however, in general, it has been shown to exhibit low oral and dermal toxicity with some evidence of higher toxicity via inhalation (DeSalva et al., Citation1989; Fang et al., Citation2010). Triclosan is not considered to produce significant mutagenic effects or genotoxicity; however, the carcinogenicity studies that have been conducted have been described as contradictory and/or inadequate (DeSalva et al., Citation1989; FDA, Citation2008; Lyman & Furia, Citation1969). Developmental and reproductive effects have also been suggested in recent studies (Lan et al., Citation2013; Paul et al., Citation2010, Citation2012; Witorsch, Citation2014). Findings from a recent epidemiological study suggest an association between prenatal exposure to triclosan and reduced early postnatal growth (Philippat et al., Citation2014). There is also evidence that triclosan may function as an endocrine disrupting compound (EDC). Studies have shown that triclosan is weakly androgenic (Foran et al., Citation2000) and estrogenic (Gee et al., Citation2008). Additionally, triclosan has been shown to disrupt thyroid homeostasis in mammalian models. Due to the lack of adequate toxicity data for dermal exposure, triclosan is currently under review by the National Toxicology Program for carcinogenicity and reproductive toxicity.
In general, triclosan has not been reported as a skin sensitizer when tested in animal models (DeSalva et al., Citation1989; Lyman & Furia, Citation1969) or in humans (DeSalva et al., Citation1989; Kligman & Epstein, Citation1975; Marzulli, Citation1973), although rare case reports have been described (Savage et al., Citation2011; Steinkjer & Braathen, Citation1988). Although not directly recognized as a sensitizer, recent human and animal studies do suggest a role for triclosan in allergic disease. Using data from the 2003–2006 National Health and Nutrition Examination Survey, a study conducted between 2003–2006 found a positive association between elevated urinary triclosan levels and allergy or hay fever diagnosis and concluded that triclosan may negatively affect the immune system (Clayton et al., Citation2011). More recent studies found positive associations between levels of urinary triclosan and aeroallergen (Bertelsen et al., Citation2013) and food sensitization, along with asthma exacerbation (Savage et al., Citation2012, Citation2014). Animal studies support these findings and suggest that, while triclosan may not be an allergen itself, it may act as an adjuvant and enhance allergic responses to a known allergen (Anderson et al., Citation2013b).
Triclosan has recently attracted the attention of the scientific community, regulatory agencies and the general public because of its high production volume, widespread applications and endocrine-disrupting effects. There is much debate about the benefits of its use in consumer products relative to its potential toxicity and environmental contamination (Halden, Citation2014). Earlier this year, concerns about the potential health effects of triclosan prompted the state of Minnesota to ban this chemical from consumer soaps statewide, starting in 2017. In an effort to help to fill some existing data gaps, this study begins to characterize the immunomodulatory effects of triclosan following dermal exposure in a murine model.
Materials and methods
Test articles and chemicals
All chemicals, triclosan (CAS# 3380-34-5), α-hexylcinnamaldehyde (HCA) [CAS# 101-86-0] and cyclophosphamide [CP; CAS# 50-18-0] were purchased from Aldrich Chemical Company, Inc. (Milwaukee, WI).
Species selection
Female BALB/c and B6C3F1 mice were used in these studies. BALB/c mice have a T-helper (TH)-2 bias and are commonly used to evaluate potential IgE-mediated sensitization and were, therefore, used in the hypersensitivity studies (Woolhiser et al., Citation2000). B6C3F1 mice are the strain of choice for immunotoxicity studies and were used to evaluate the IgM response to sheep red blood cells (SRBC) (Luster et al., Citation1992).
All mice were purchased from Taconic (Germantown, NY) at 6–8-weeks-of-age. On arrival, the animals were allowed to acclimate for a minimum of 5 days. Each shipment of animals was randomly assigned to treatment group, weighed and individually identified via tail tattoo or marking using a permanent marker. A preliminary analysis of variance on body weights was performed to ensure an homogeneous distribution of animals across treatment groups. The mice were housed at a maximum of five mice/cage in ventilated plastic shoebox cages with hardwood chip bedding. NIH-31 modified 6% irradiated rodent diet (Harlan Teklad, Indianapolis, IN) and tap water was provided from water bottles, ad libitum. Temperature in the facility was maintained at 68–72°F and relative humidity at 36–57%; a light/dark cycle was maintained at 12-h intervals. All animal experiments were performed in an Association for Assessment and Accreditation of Laboratory Animal Care (AAALAC) accredited National Institute for Occupational Safety and Health (NIOSH) animal facility in accordance with an animal protocol approved by the Institutional Animal Care and Use Committee (IACUC).
Triclosan exposures
The concentrations of triclosan used in these studies were based on findings from previous studies (Anderson et al., Citation2013b). For the hypersensitivity study, BALB/c mice (five mice/group) were topically treated with acetone vehicle, increasing concentrations of triclosan or positive control [30% HCA (v/v; sensitization positive control)] on the dorsal surface of each ear (25 μl/ear) once a day for 3 consecutive days. For the immune phenotyping and hematology studies, B6C3F1 mice (n = 5) were topically exposed to acetone or increasing concentrations of triclosan on the dorsal surface of each ear (25 μl/ear) once a day for 28 consecutive days. For analysis of the IgM response to SRBC, B6C3F1 mice (n = 6) were topically exposed to acetone or increasing concentrations of triclosan on the dorsal surface of each ear (25 μl/ear) once a day for 28 consecutive days. Cyclophosphamide (20 mg/kg in isotonic sterile saline) was included as positive control for analysis of the IgM response to SRBC and was injected intraperitoneally for 4 consecutive days prior to sacrifice.
Murine local lymph node assay
To determine the sensitization potential of triclosan, a local lymph node assay (LLNA) was conducted. Triclosan dosing concentrations (0.75–3.0%) and vehicle (acetone) were selected based on findings from previous studies (Anderson et al., Citation2013b). The murine LLNA was performed according to the methods previously described by Anderson et al. (Citation2013a).
Phenotypic analysis
Animals were euthanized by CO2 inhalation 24 h after the final exposure, weighed and examined for gross pathology. Blood was collected in EDTA-coated Vacutainer tubes following transection of the abdominal aorta and hematological analysis was conducted (see below). The liver, spleen, kidneys and thymus were removed, cleaned of connective tissue and weighed. Left and right auricular draining lymph nodes (DLN; drain site of chemical application) and spleen cell suspensions were prepared by mechanical disruption of tissues between frosted microscope slides in phosphate-buffered saline (PBS, pH 7.4) and counted on a Cellometer (Nexcelom, Lawrence, MA). Cells were then aliquoted (1–2 × 106) into a 96-well U-bottom plate and washed in staining buffer (PBS + 1% bovine serum albumin + 0.1% sodium azide). Cells were re-suspended in staining buffer containing anti-mouse CD16/32 antibody (clone 2.4G2) for blocking of Fc receptors (BD Biosciences, San Jose, CA). Cells were next re-suspended in staining buffer containing a cocktail of fluorochrome-conjugated antibodies specific for cell surface antigens: CD45-Allophycocyanin (clone 30-F11), CD3e-V500 (500A2), CD4-Allophycocyanin-H7 (GK1.5), CD8a-PE-CF594 (53-6.7), CD45R/B220-Alexa Fluor 700 (RA3-6B2), NK1.1-FITC (PK136) (BD Biosciences), CD11c-eFluor 450 (N418) and CD11b-PerCP-Cyanine5.5 (M1/70) (eBioscience, San Diego, CA). Cells were then washed in staining buffer and fixed in Cytofix buffer (BD Biosciences). Within 24 h, cells were re-suspended in staining buffer and analyzed on an LSR II flow cytometer (BD Biosciences). Data analysis was performed with FlowJo 7.6.5 software (TreeStar Inc., Ashland, OR). A minimum of 50 000 events was captured for each sample. Leukocytes were first identified by their expression of CD45 and the subsets were further identified as follows: CD4 T-cells (CD4+CD3+), CD8 T-cells (CD8+CD3+), B-cells (B220+CD3−), NK cells (NK1.1+CD3−) and dendritic cells (CD11b+CD11c+).
Hematology
Select hematological parameters were evaluated using a Hemavet 950 automatic hematology analyzer (Drew Scientific, Waterbury, CT). Endpoints analyzed included peripheral erythrocyte and leukocyte counts, leukocyte differentials (lymphocytes, neutrophils, monocytes, basophils, and eosinophils), platelet counts, hematocrit, hemoglobin levels, mean corpuscular hemoglobin (MCH) and hemoglobin concentration (MCHC), mean platelet volume (MCV) and platelet distribution width (PDW).
Spleen in vivo response to the T cell-dependent antigen SRBC
The primary IgM response to SRBC was enumerated using a modified hemolytic plaque assay. Four days prior to euthanasia (i.e. Day 29), the mice were immunized with 7.5 × 107 SRBC (in 200 μl volume) by intravenous injection. All SRBC for these studies were drawn from a single donor animal (Lampire Laboratories, Pipersville, PA). On the day of sacrifice, mice were euthanized by CO2 inhalation, body and organ weights were recorded and spleens were collected in 3 ml Hank's balanced salt solution (HBSS). Blood was also retrieved in serum collection tubes following transection of the abdominal aorta and stored at −20 °C for subsequent analysis of serum anti-SRBC IgM levels (see below). Single cell suspensions of the spleens from individual animals were prepared in HBSS by disrupting the spleen between the frosted ends of microscope slides. To identify the total number of spleen cells, 20 μl of cells were added to 10 ml Isoton II diluent (1:500; Beckman Coulter, Indianapolis, IN) and two drops of Zap-o-globin II Lytic Reagent (Beckman Coulter) were added to lyse red blood cells. Cells were then counted using a Coulter Counter.
Dilutions (1:30 and 1:120) of spleen cells were then prepared and 100 μl of each dilution were added to test tubes containing a 0.5 ml warm agar/dextran mixture (0.5% Bacto-Agar, DIFCO; and 0.05% DEAE dextran; Sigma, St. Louis, MO), 25 μl of 1:1 ratio of SRBC suspension and 25 μl of 1:4 dilution (1 ml lyophilized) guinea pig complement (Cedarlane Labs, Burlington, Canada). Each sample was vortexed, poured into a petri dish, cover-slipped and incubated for 3 h at 37 °C. The plaques (representing antibody-forming B-cells or plaque-forming cells [PFC]) were then counted. Results were expressed in terms of both specific activity (IgM PFC per 106 spleen cells) and total activity (IgM PFC per spleen).
Serum IgM response to SRBC
Serum samples were analyzed for anti-SRBC IgM using a commercially available ELISA kit (Life Diagnostics, West Chester, PA), according to manufacturer recommendations with modifications. In brief, test serum was diluted (1:200, 1:400, 1:800 and 1:1,600) and incubated in the anti-SRBC coated micro-titer wells for 45 min at 25 °C. The wells were subsequently washed, 100 μl horseradish peroxidase-conjugated secondary antibody was added and the plates incubated for an additional 45 min at 25 °C. Thereafter, the wells were washed to remove unbound antibodies and 100 μl tetramethylbenzidine peroxidase (TMB) reagent was added to each well. The plates were incubated for 20 min at room temperature before color development was stopped by addition of 50 μl of kit-provided Stop Solution. Optical density in each well was then measured spectrophotometrically at 450 nm using a Spectra Max M2 plate reader (Molecular Devices, Sunnyvale, CA). The concentration of anti-SRBC IgM in the test samples was determined by comparison to a standard curve generated in parallel using SoftMax Pro software and reported as units of anti-SRBC IgM (U/ml) plotted vs absorbance values at 450 nm.
Statistical analyses
For analysis of the data generated from the described animal studies, the data were first tested for homogeneity using the Bartlett's Chi Square test. If homogeneous, a one-way analysis of variance (ANOVA) was conducted. If the ANOVA showed significance at p < 0.05 or less, the Dunnett's Multiple Range t-test was used to compare treatment groups with the control group. Linear trend analysis was performed to determine if triclosan had exposure concentration-related effects for the specified endpoints. Statistical analysis was performed using GraphPad Prism v5.0 (San Diego, CA). Statistical significance was designated at *p ≤ 0.05 and **p ≤ 0.01.
Results
In vivo studies did not identify triclosan as an allergic sensitizer in the LLNA
All animals appeared clinically normal throughout the course of the study and there were no changes in body weight. These animals had no visible signs of skin irritation or inflammation during the course of this study following exposure to triclosan (). No significant increase in DLN proliferation was identified after treatment with triclosan (); however, a slight increase in proliferation (1254 ± 385) was observed following exposure to 3.0% triclosan compared to the vehicle control (607 ± 128), resulting in a stimulation index (SI; fold-change above vehicle control) value of 2.1. HCA (30%) was used as a positive control for these experiments, resulting in an average SI value of 8.9.
Figure 2. Allergic sensitization potential after dermal exposure to triclosan. Analysis of allergic sensitization potential of triclosan using LLNA. Disintegration per minute (DPM) represent [3H]-thymidine incorporation into draining lymph node cells of BALB/c mice following exposure to vehicle or concentration of triclosan (0.75–3.0%). SI value is stimulation index (fold-change over vehicle control). Bars shown are means (±SE) of five mice per group.
![Figure 2. Allergic sensitization potential after dermal exposure to triclosan. Analysis of allergic sensitization potential of triclosan using LLNA. Disintegration per minute (DPM) represent [3H]-thymidine incorporation into draining lymph node cells of BALB/c mice following exposure to vehicle or concentration of triclosan (0.75–3.0%). SI value is stimulation index (fold-change over vehicle control). Bars shown are means (±SE) of five mice per group.](/cms/asset/f9b560e8-54f7-4c35-b144-a387020652d7/iimt_a_1029146_f0002_b.jpg)
Dermal exposure to triclosan for 28 days results in increased liver weights
There was no loss of body weight or overt signs of toxicity in animals exposed to triclosan for 28 days. However, animals exposed to 3.0% triclosan developed a mild irritation and scabbing/crusting on the ears after ∼ 2 weeks of exposure that resolved by the end of the study. In contrast to body weight data, a statistically significant increase in liver weights was observed following exposure to 1.5% and 3.0% triclosan (11% and 44%, respectively, vs values for vehicle-treated mice) (). There was also a statistically significant increase in spleen weight following exposure to 3.0% triclosan. While this increase was not significant when expressed as percentage body weight, there was a significant increasing trend for both spleen weights and spleen as a percentage of body weight (Linear Trend Test, p < 0.05). No other significant changes in body or organ weight were observed following exposure to any concentration of triclosan (). There was a dose-dependent increase (Linear Trend Test, p < 0.05) in platelets reaching statistical significance at 3.0%, but dermal exposure to triclosan did not alter any other analyzed hematological parameter ().
Table 1. Body\organ weights of female B6C3F1 mice dermally exposed to triclosan for 28 days.
Table 2. Hematology parameters of female B6C3F1 mice dermally exposed to triclosan for 28 days.
Dermal exposure to triclosan increased number and sub-populations of leukocytes in DLN, but not spleen
Dermal exposure to triclosan resulted in a dose-dependent (Linear Trend Test, p < 0.05) increase in DLN cellularity, reaching statistical significance at 1.5% and 3.0% (). Significant increases in the absolute number of B-cells, T-cells (CD4 and CD8), NK cells and dendritic cells were observed in DLN (), reaching statistical significance at 1.5% and 3.0% for all populations. A small but significant increase in the frequency of the dendritic cell population was observed in the DLN following exposure to 3.0% triclosan (). No significant changes were observed in the frequency of the other cell populations in the DLN. No differences in cellularity, absolute number or frequency of cells were identified in the spleen ( and ).
Figure 3. Effects of dermal exposure to triclosan on lymph node and spleen cellularity. Effects of dermal exposure to triclosan for 28 days on (A) lymph node and (B) spleen cellularity in female B6C3F1 mice. Values shown are means (±SE) for each group. Levels of statistical significance are denoted *p < 0.05 and **p < 0.01 as compared to acetone vehicle.
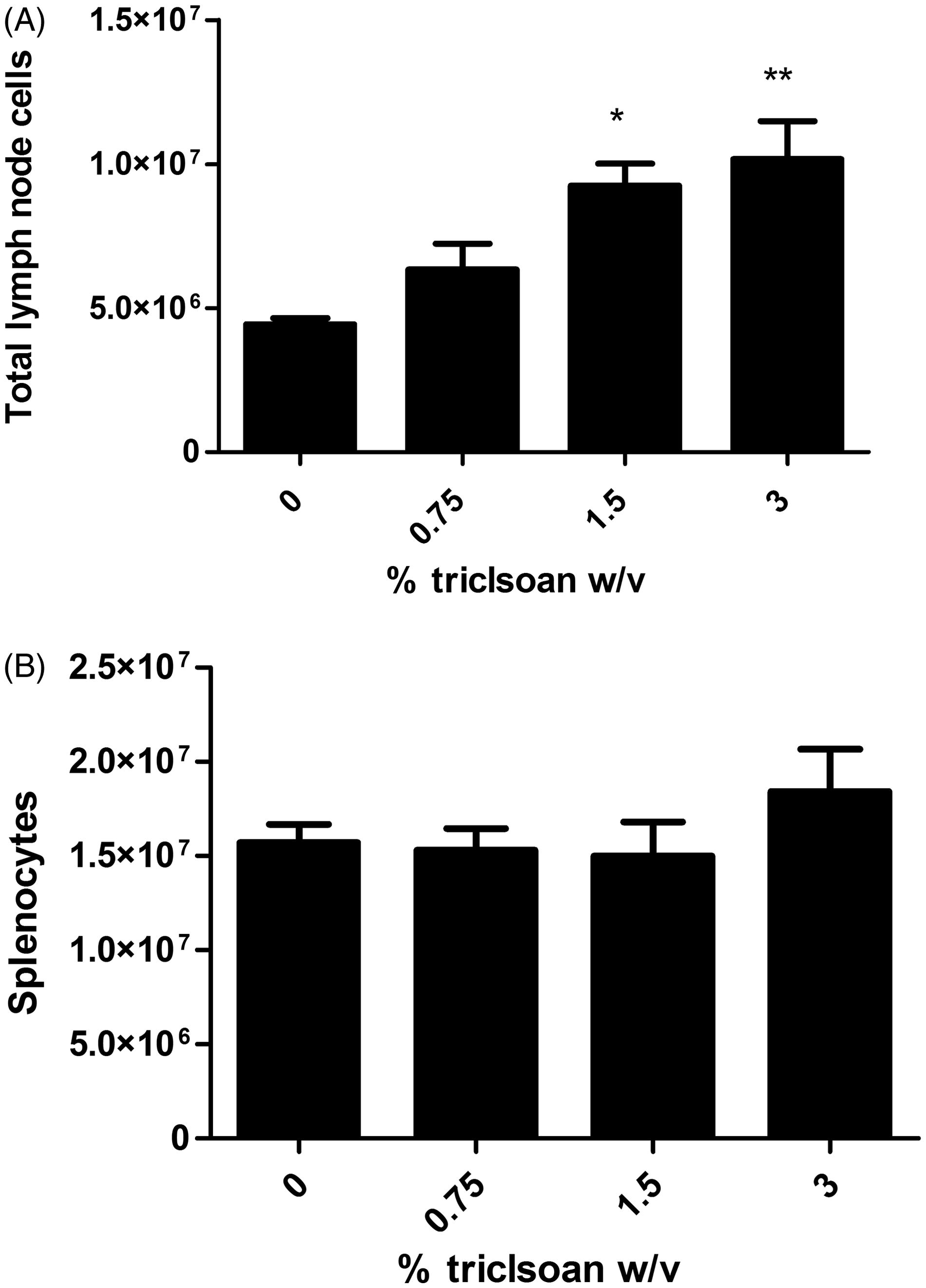
Figure 4. Effects of dermal exposure to triclosan for 28 days on leukocyte populations. Effects of dermal exposure to triclosan for 28 days on (A) total cell number and (B) frequency of lymphocyte subpopulations in female B6C3F1 mice. Numbers of B-cells, CD4+ and CD8+ T-cells, dendritic cells and NK cells were enumerated using flow cytometry. Values shown are means (±SE) for each group. Levels of statistical significance are denoted *p < 0.05 and **p < 0.01 as compared to acetone vehicle.
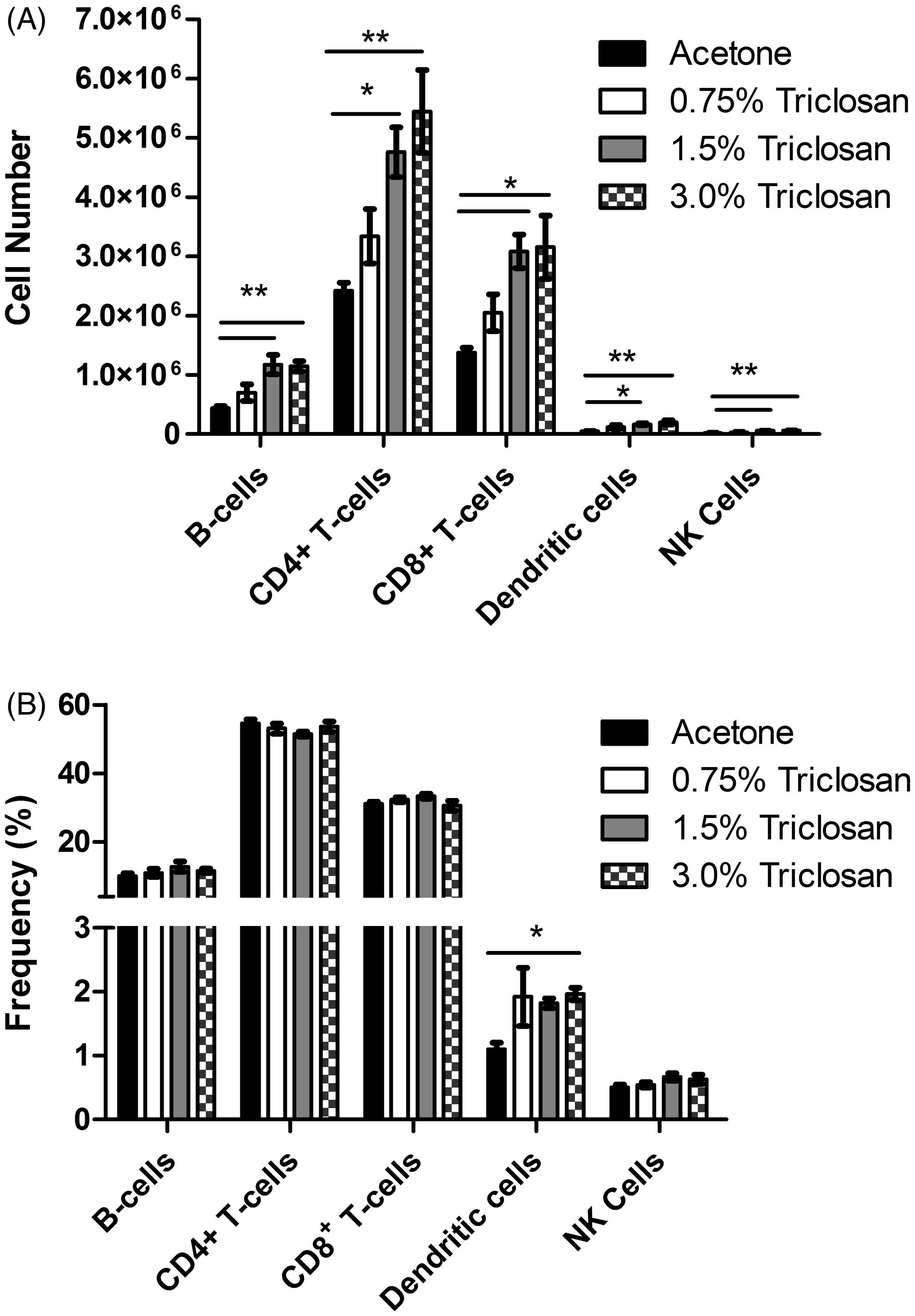
Figure 5. Effects of dermal exposure to triclosan for 28 days on splenocyte populations. Effects of dermal exposure to triclosan for 28 days on (A) total cell number and (B) frequency of lymphocyte subpopulations in female B6C3F1 mice. Numbers of B-cells, CD4+ and CD8+ T-cells, dendritic cells and NK cells were enumerated using flow cytometry. Values shown are means (±SE) for each group.
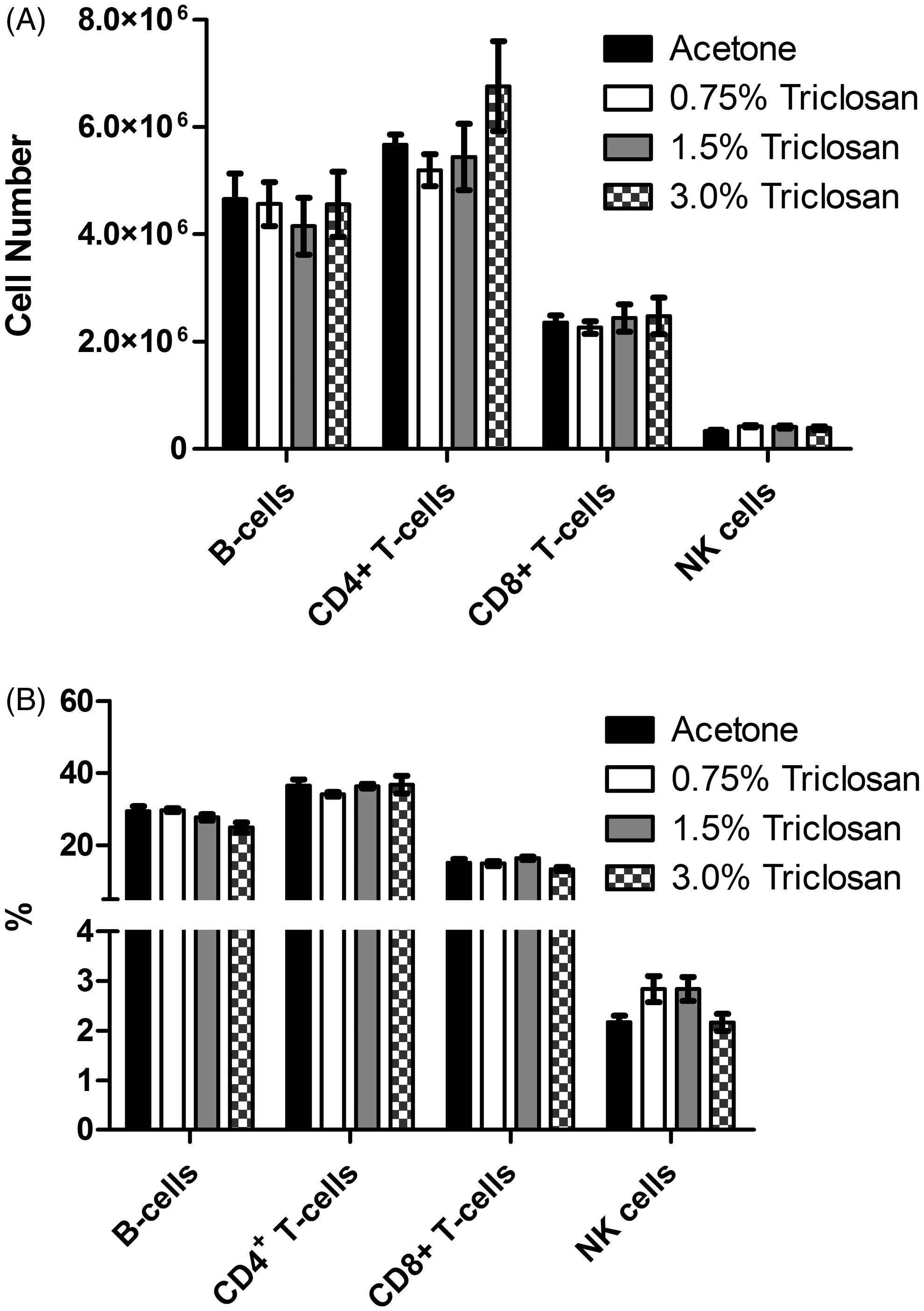
Dermal exposure to triclosan did not suppress the splenic or serum IgM response to SRBC
To evaluate if exposure to triclosan was immunosuppressive, the murine IgM response to SRBC was examined following a 28-day exposure to triclosan. No statistically significant reductions in the PFC/spleen and specific (PFC/106 cells) IgM antibody activity against SRBC were observed after exposure to triclosan ( and ). However, exposure of mice to 0.75% triclosan resulted in statistically significant increases in PFC/spleen and PFC/106 cells (45 and 54%, respectively, vs values for vehicle-treated mice) (). Although not statistically significant, this increase was also observed in the serum anti-SRBC IgM levels (). Mice exposed to positive control cyclophosphamide (CP) had a significantly reduced specific spleen IgM response and total IgM response compared to levels noted in vehicle-treated controls ().
Figure 6. Triclosan does not suppress the spleen IgM response to SRBC. Analysis of antibody producing cells after a 28-day dermal exposure to triclosan on the (A) total and (B) specific activity IgM response to SRBC in the spleen and serum. (C) Bars represent mean fold-change (±SE) of six mice/group. Cyclophosphamide (CP) was included as the positive control. Levels of statistical significance are denoted *p < 0.05 and **p < 0.01 as compared to acetone vehicle.
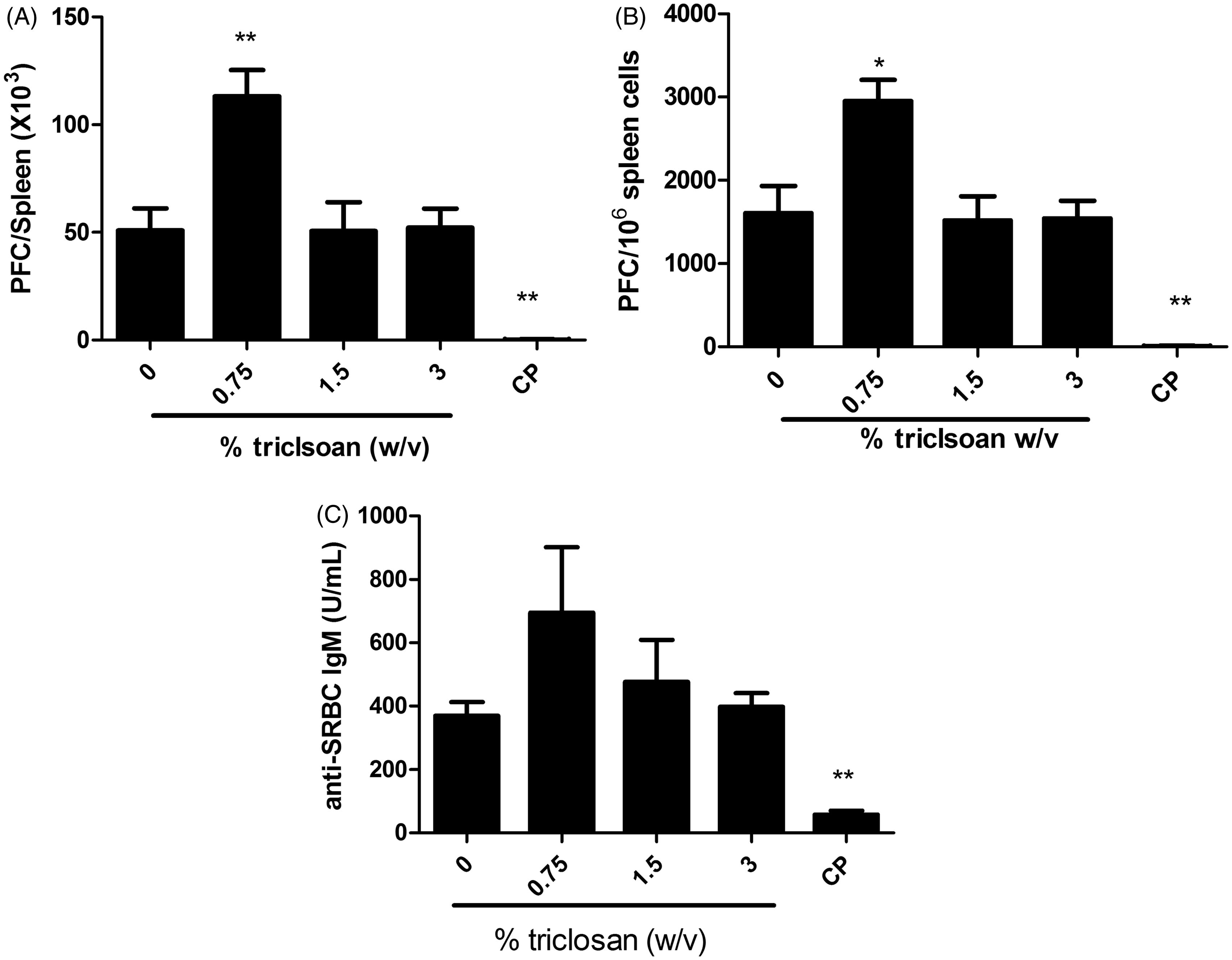
Discussion
The immune system is very sensitive to a variety of chemical and physical stressors and can, therefore, be used as a tool to examine the subclinical effects of chemical exposure (Luster et al., Citation1992). Exposures to chemicals have been shown to induce immune abnormalities; therefore, there is an increasing need for the immunological evaluation of exposure to these agents. In an attempt to fill some of the data gaps associated with triclosan exposure-related health effects, the immunotoxicity of triclosan following dermal exposure was evaluated in the studies described here.
The results from these studies confirm that triclosan is a non-sensitizing chemical, as evidenced by the lack of significant lymphocyte proliferation in the LLNA. While an EC3 (3-fold increase over vehicle control) could not be calculated for triclosan in the LLNA after 3 days of dermal exposures, there was a significant dose-dependent increase in DLN cellularity after the prolonged 28 days of dermal exposure. Flow cytometric analysis identified this increase in cellularity to be a result of significant increases in the total number of B-cells, T-cells, dendritic cells and NK cells in the DLN, also indicating the potential for immune stimulation. Interestingly the frequency of dendritic cells also increased following exposure to the highest concentration of triclosan in the DLN. Dendritic cells, derived from hematopoietic bone marrow progenitor cells, are extremely important initiators and regulators of immune responses due to their critical role in transferring information about the environment to the adaptive immune system (Ainscough et al., Citation2013). Previous studies conducted in our laboratory have shown that dermal exposure to triclosan – while not allergenic alone – can augment allergic responses to ovalbumin in a mouse model of asthma (Anderson et al., Citation2013a). Based on the findings of our previous work and the current study, although not directly sensitizing, triclosan does induce immune-related responses following dermal exposure.
The T cell dependent antibody response is one of the most sensitive indicators of immune integrity because it relies on an organized immune response that is dependent on the functional capacity and cooperation of numerous cell types including B-cells, T-cells and macrophages (Anderson et al., Citation2006). While these studies did not show a suppression of the IgM response to SRBC following triclosan exposure, there was a significant increase in the IgM response to SRBC at the low concentration (0.75%) for both the total and specific activity. While this finding does not suggest that dermal exposure to triclosan is immunosuppressive, it does further support the hypothesis that triclosan can activate the immune system. These effects occurred in the absence of alterations in splenic cellularity and phenotype; however, the kinetics of these responses was not examined. In addition, while the dermal toxicokinetic studies did detect radiolabeled triclosan in the spleen of exposed animals, the levels were minimal (Fang et al., Citation2014). While this is a non-traditional finding for this assay, it does suggest stimulation of the immune system similar to what was observed in the lymph nodes, although only at the lowest concentration. While the biological significance and mechanism of this finding is currently unknown, indirect activation occurring as a result of cytokine or hormonal signaling is one possible explanation. Studies investigating the immunotoxicity of malathion have reported similar kinds of findings for this assay (Johnson et al., Citation2002).
In the present study, exposure to triclosan induced significantly increased platelet counts and liver weights, further support that dermal exposure to triclosan can induce systemic effects. Similar to the work described in this manuscript, a study conducted by Zorrilla et al. (Citation2009) also observed increases in liver weight following oral gavage exposures (100–300 mg/kg) to triclosan in male Wistar rats. Upon further evaluation, the authors found that there were significant alterations in markers for hepatic enzymes (CYP2B and CYP1A1) following exposure to triclosan. Studies conducted by Rodricks et al. (Citation2010) identified an increased frequency of hepatocellular adenomas and carcinomas in male and female mice following long-term exposure to triclosan. A function of the liver is to produce thrombopoietin that regulates the production of platelets through production and differentiation of megakaryocytes in the bone marrow. In these studies, a dose-dependent increase in platelets was observed in mice exposed to triclosan. Taken together, these findings suggest the liver may be a potential target for triclosan.
In summary, triclosan was identified as a non-sensitizing chemical capable of inducing stimulation of the immune system reflected by increases in numbers (B-cells, T-cells, NK cells and dendritic cells) and frequency (dendritic cells) of cells in the DLN, increased splenic IgM response to SRBC and increased liver weights and platelet counts following 28-days of dermal exposure. These results, in addition to its high production volume, widespread applications and potential endocrine-disrupting effects encourage additional investigations into the effects of triclosan and increased awareness about triclosan exposure. These are the first studies to evaluate the immunotoxicity induced by dermal exposure to triclosan using a murine model. Although significant data gaps still exist for the complete toxicological evaluation of this chemical, these results suggest that triclosan, when evaluated in a murine model, can produce immune-related effects when tested at concentrations similar to those used in consumer products and may induce liver specific toxicity. The results presented here raise concern for the need for additional, long-term exposure studies.
Acknowledgments
The authors express special thanks to Katie Anderson for her technical assistance. The findings and conclusions in this report are those of the authors and do not necessarily represent the official position of the National Institute for Occupational Safety and Health.
Declaration of interest
The authors report no conflicts of interest. The authors alone are responsible for the content and writing of the paper.
References
- Adolfsson-Erici, M., Pettersson, M., Parkkonen, J., and Sturve, J. 2002. Triclosan, a commonly used bactericide found in human milk and in the aquatic environment in Sweden. Chemo-sphere 46:1485–1489
- Ainscough, J. S., Frank Gerberick, G., Dearman, R. J., and Kimber, I. 2013. Danger, intracellular signaling, and orchestration of dendritic cell function in skin sensitization. J. Immunotoxicol. 10:223–234
- Anderson, S. E., Franko, J., Anderson, K. L., et al. 2013a. Immunotoxicity and allergic potential induced by topical application of dimethyl carbonate (DMC) in a murine model. J. Immunotoxicol. 10:59–66
- Anderson, S. E., Franko, J., Kashon, M. L., et al. 2013b. Exposure to triclosan augments allergic response to ovalbumin in a mouse model of asthma. Toxicol. Sci. 132:96–106
- Anderson, S. E., Munson, A. E., and Meade, B. J. 2006. Analysis of immunotoxicity by enumeration of antibody-producing B-cells. In: Current Protocols in Toxicology (Bus, J. S., Costa, L. G., Hodgson, E., et al. Eds.). Hoboken, NJ: Wiley and Sons, pp. 18.11.1–18.11.19
- Bertelsen, R. J., Longnecker, M. P., Lovik, M., et al. 2013. Triclosan exposure and allergic sensitization in Norwegian children. Allergy 68:84–91
- Black, J. G., Howes, D., and Rutherford, T. 1975. Percutaneous absorption and metabolism of Irgasan DP300. Toxicology 3:33–47
- Calafat, A. M., Ye, X., Wong, L. Y., et al. 2008. Urinary concentrations of triclosan in U.S. population: 2003–2004. Environ. Health Perspect. 116:303–307
- Clayton, E. M., Todd, M., Dowd, J. B., and Aiello, A. E. 2011. The impact of bisphenol A and triclosan on immune parameters in U.S. population, NHANES 2003–2006. Environ. Health Perspect. 119:390–396
- DeSalva, S. J., Kong, B. M., and Lin, Y. J. 1989. Triclosan: A safety profile. Am. J. Dentistry 2:185–196
- Fang, J. L., Stingley, R. L., Beland, F. A., et al. 2010. Occurrence, efficacy, metabolism, and toxicity of triclosan. J. Environ. Sci. Health 28:147–171
- Fang, J. L., Vanlandingham, M., da Costa, G. G., and Beland, F. A. 2014. Absorption and metabolism of triclosan after application to the skin of B6C3F1 mice. Environ. Toxicol. (DOI: 10.1002/tox.22074). Retrieved from: http://ntp.niehs.nih.gov/ntp/htdocs/chem_background/exsumpdf/triclosan_508.pdf [last accessed 19 Mar 2015]
- FDA (Food and Drug Administration). 2008. Triclosan: Supporting Information for Toxicological Evaluation by National Toxicology Program. USFDA. Washington, DC: Department of Health and Human Services
- Foran, C. M., Bennett, E. R., and Benson, W. H. 2000. Developmental evaluation of a potential nonsteroidal estrogen: Triclosan. Marine Environ. Res. 50:153–156
- Gee, R. H., Charles, A., Taylor, N., and Darbre, P. D. 2008. Estrogenic and androgenic activity of triclosan in breast cancer cells. J. Appl. Toxicol. 28:78–91
- Glaser, A. 2004. The Ubiquitous Triclosan: A Common Antibacterial Agent Exposed. Beyond Pesticides/National Coalition Against Misuse of Pesticides. Available at: http://www.beyondpesticides.org/pesticides/factsheets/Triclosan=20cited.pdf. [last accessed 22 Jan 2015]
- Gunsolley, J. C. 2006. A meta-analysis of six-month studies of anti-plaque and anti-gingivitis agents. J. Am. Dental Assoc. 137:1649–1657
- Halden, R. U. 2014. On the need and speed of regulating triclosan and triclocarban in the United States. Environ. Sci. Technol. 48:3603–3611
- Hines, E. P., Mendola, P., von Ehrenstein, O. S., et al. 2014. Concentrations of environmental phenols and parabens in milk, urine and serum of lactating North Carolina women. Reprod. Toxicol. doi:10.1016/j.reprotox.2014.11.006. (online 11–22–14)
- Johnson, V. J., Rosenberg, A. M., Lee, K., and Blakley, B. R. 2002. Increased T-lymphocyte dependent antibody production in female SJL/J mice following exposure to commercial-grade malathion. Toxicology 170:119–129
- Jones, R. D., Jampani, H. B., Newman, J. L., and Lee, A. S. 2000. Triclosan: A review of effectiveness and safety in health care settings. Am. J. Infect. Control 28:184–196
- Kanetoshi, A., Katsura, E., Ogawa, H., et al. 1992. Acute toxicity, percutaneous absorption, and effects on hepatic mixed-function oxidase activities of 2,4,4′-trichloro-2′-hydroxydiphenyl ether (Irgasan DP300) and its chlorinated derivatives. Arch. Environ. Contam. Toxicol. 23:91–98
- Kligman, A. M., and Epstein, W. 1975. Updating the maximization test for identifying contact allergens. Contact Dermatitis 1:231–239
- Lan, Z., Hyung Kim, T., Shun Bi, K., et al. 2013. Triclosan exhibits a tendency to accumulate in the epididymis and shows sperm toxicity in male sprague-dawley rats. Environ. Toxicol. 30:83–91
- Lindstrom, A., Buerge, I. J., Poiger, T., et al. 2002. Occurrence and environmental behavior of the bactericide triclosan and its methyl derivative in surface waters and in wastewater. Environ. Sci. Technol. 36:2322–2329
- Luster, M. I., Pait, D. G., Portier, C., et al. 1992. Qualitative and quantitative experimental models to aid in risk assessment for immunotoxicology. Toxicol. Lett. 64:71–78
- Lyman, F. L., and Furia, T. 1969. Toxicology of 2,4,4′-trichloro-2′-hydroxy-diphenyl ether. Indust. Med. Surgery 38:64–71
- MacIsaac, J. K., Gerona, R. R., Blanc, P. D., et al. 2014. Health care worker exposures to the anti-bacterial agent triclosan. J. Occup. Environ. Med. 56:834–839
- Marzulli, F. N. 1973. Anti-microbials: Experimental contact sensitization in man. J. Soc. Cosmet. Chem. 24:399–421
- Ming, X., Rothenburger, S., and Yang, D. 2007. In vitro anti-bacterial efficacy of Monocryl plus anti-bacterial suture (Poliglecaprone 25 with triclosan). Surg. Infect. 8:201–208
- Moss, T., Howes, D., and Williams, F. M. 2000. Percutaneous penetration and dermal metabolism of triclosan (2,4,4′-trichloro-2′-hydroxydiphenyl ether). Food Chem. Toxicol. 38:361–370
- Paul, K. B., Hedge, J. M., Bansal, R., et al. 2012. Developmental triclosan exposure decreases maternal, fetal, and early neonatal thyroxine: A dynamic and kinetic evaluation of putative mode-of-action. Toxicology 300:31–45
- Paul, K. B., Hedge, J. M., De Vito, M. J., and Crofton, K. M. 2010. Developmental triclosan exposure decreases maternal and neonatal thyroxine in rats. Environ. Toxicol. Chem. 29:2840–2844
- Philippat, C., Botton, J., Calafat, A. M., et al. 2014. Prenatal exposure to phenols and growth in boys. Epidemiology (Cambridge) 25:625–635
- Pycke, B. F., Geer, L. A., Dalloul, M., et al. 2014. Human fetal exposure to triclosan and triclocarban in an urban population from Brooklyn, New York. Environ. Sci. Technol. 48:8831–8838
- Rodricks, J. V., Swenberg, J. A., Borzelleca, J. F., et al. 2010. Triclosan: A critical review of the experimental data and development of margins of safety for consumer products. Crit. Rev. Toxicol. 40:422–484
- Sandborgh-Englund, G., Adolfsson-Erici, M., Odham, G., and Ekstrand, J. 2006. Pharmaco-kinetics of triclosan following oral ingestion in humans. J. Toxicol. Environ. Health 69:1861–1873
- Savage, J. H., Johns, C. B., Hauser, R., and Litonjua, A. A. 2014. Urinary triclosan levels and recent asthma exacerbations. Ann. Allergy Asthma Immunol. 112:179–181
- Savage, J. H., Matsui, E. C., Wood, R. A., and Keet, C. A. 2012. Urinary levels of triclosan and parabens are associated with aeroallergen and food sensitization. J. Allergy Clin. Immunol. 130:453–460
- Savage, L., Rose, R., and Wilkinson, M. 2011. Airborne contact dermatitis to triclosan. Contact Dermatitis 65:239–240
- Steinkjer, B., and Braathen, L. R. 1988. Contact dermatitis from triclosan (Irgasan DP 300). Contact Dermatitis 18:243–244
- Tan, L., Nielsen, N. H., Young, D. C., Trizna, Z., and Council on Scientific Affairs (AMA). 2002. Use of anti-microbial agents in consumer products. Arch. Dermatol. 138:1082–1086
- Witorsch, R. J. 2014. Critical analysis of endocrine disruptive activity of triclosan and its relevance to human exposure through the use of personal care products. Crit. Rev. Toxicol. 44:535–555
- Woolhiser, M. R., Munson, A. E., and Meade, B. J. 2000. Comparison of mouse strains using the local lymph node assay. Toxicology 146:221–227
- Zorrilla, L. M., Gibson, E. K., Jeffay, S. C., et al. 2009. The effects of triclosan on puberty and thyroid hormones in male Wistar rats. Toxicol. Sci. 107:56–64