Abstract
Asbestos is known to cause malignant mesothelioma and lung cancer. Recent studies implicate tumor immunity in the development of various tumors, including malignant mesothelioma. In order to establish an in vitro T-cell model to clarify the effects of long-term exposure of asbestos on tumor immunity, in this study, human T-cell line MT-2 cells were cultured with asbestos for longer than 8 months and the resultant cells (MT-2Rst) were assessed for the expression of forkhead transcription factor FoxO1. Gene expression analysis revealed that the amount of FoxO1 mRNA decreased after long-term exposure of the MT-2 cells to asbestos. In accordance with this reduction in FoxO1, pro-apoptotic Foxo1 target genes Puma, Fas ligand and Bim were also seen to be down-regulated in MT-2Rst cells. Furthermore, shRNA-mediated knock-down of FoxO1 reduced the number of apoptotic parental MT-2 cells after treatment with asbestos. On the other hand, over-expression of FoxO1 did not affect asbestos-induced apoptosis in MT-2Rst cells. These results suggested that FoxO1 played an important role in regulating asbestos-induced apoptosis and confirmed the presence of multiple pathways regulating resistance to asbestos in MT-2Rst cells.
Introduction
Asbestos is a set of natural fibrous silicate minerals that includes chrysotile, crocidolite and amosite and has been used in a wide range of industrial applications for its physical properties such as resistance to fires, heat and chemical damage. It has been clearly shown that prolonged inhalation of asbestos fibers causes serious diseases in pulmonary tissues, malignant pleural mesothelioma (MPM), lung cancer, asbestos-related pleural plaque (PP) and pulmonary fibrosis in humans (Lemen et al. Citation2006; Craighead et al. Citation2008). While trade involving asbestos is now restricted or banned in many countries, there are still risks with existing buildings whose structures include asbestos.
MPM is the most serious of the asbestos-related diseases because it is a highly aggressive tumor and resistant to currently available therapies (Roggli et al. Citation2004; Robinson et al. Citation2005). Many studies have and still are attempting to clarify mechanisms of MPM development caused by asbestos. For example, it was an important finding that the reactive oxygen species (ROS) produced from iron found in asbestos fibers (such as crocidolite and amosite) caused DNA damage in alveolar epithelial and mesothelial cells (Toyokuni Citation2009; Huang et al. Citation2011). Recent investigations have also implicated tumor immunity in the onset/development of various tumors, including MPM (Schreiber et al. Citation2011; Izzi et al. Citation2012). Asbestos fibers have also been shown to cause reduced expression of NKp46 activating receptor and the release of cell-killing granules containing perforin and granzyme B in natural killer (NK) cells (Nishimura et al. Citation2009a,Citationb). With cytotoxic T-cells (CTL), asbestos repressed differentiation of naïve CTL to effector/memory CTL as well as their ability to proliferate (Maeda et al. Citation2010; Kumagai-Takei et al. Citation2013). Taking this and other earlier information together, we hypothesized that asbestos affected immunological systems in addition to impacting on epithelial and mesothelial cells in pulmonary tissues and that this inactivated host responses against any induced tumor cells.
In our earlier studies, MT-2 – a polyclonal T-cell line immortalized with human adult leukemia virus-1 – was cultured with a low concentration of chrysotile A, chrysotile B or crocidolite for >8 months (Miura et al. Citation2006; Maeda et al. Citation2011, Citation2012). The resultant seven independent sub-lines were designated CA (chrysotile A) 1–3, CB (chrysotile B) 1–3 and CR (crocidolite). These cell lines are useful to investigate the effect of continuous exposure of asbestos on T-cells since elevations in levels of anti-apoptotic factor Bcl-2 or interleukin (IL)-10 were observed both in MT-2 cells and in CD4+ T-cells purified from PP or MPM patients (Miura et al. Citation2006). These sub-lines also showed resistance to asbestos-induced apoptosis, even though exposure to asbestos induced apoptosis in the original MT-2 cells. Those investigations also reported that tyrosine kinase Src was active in MT-2CA1 cells and was implicated in resistance against the effects of asbestos. Activation of Src leads to the production of IL-10 that, in turn, induces over-expression of anti-apoptotic factor Bcl-2 in a process mediated by signal transducer and activator transcription factor 3 (STAT3). Nevertheless, it remains unclear whether activation of the Src/Bcl-2 pathway was the sole mechanism preventing apoptosis induced by asbestos.
Our laboratory has previously performed gene expression analysis of MT-2CA1–3 and of CB1–3 and compared findings against those in parental MT-2 cells (Maeda et al. Citation2011). That analysis revealed that forkhead transcription factor class O1 (FoxO1) was down-regulated after long-term exposure of the cells to asbestos. FoxO1 and its related molecules FoxO3 and FoxO4 belong to the winged-helix transcription factor family (Accili & Arden Citation2004; Eijkelenboom & Burgering Citation2013). Each is activated by ROS or by depletion of growth factors via prost-translational modifications such as phosphorylation, acetylation and ubiquitination. Activated FoxO family members regulate a wide range of cellular processes such as apoptosis, cell cycle arrest and various metabolic processes. Many genes have been identified as targets of FoxO family members, including pro-apoptotic genes such as Bcl-2 interacting mediator (Bim), p53 up-regulated modulator of apoptosis (Puma), Fas ligand and TRAIL.
In the study reported here, FoxO1 signaling in MT-2CA1 cells was analyzed and whether down-regulation of FoxO1 was implicated in resistance to asbestos-induced apoptosis in MT-2CA1 cells – in addition to up-regulation of the Src/Bcl-2 survival pathway – was also investigated.
Materials and methods
Reagents
Chrysotile A, chrysotile B and crocidolite were Union for International Cancer Control standard samples and obtained from the Japan Asbestos Association (Kohyama et al. Citation1996). Anti-FoxO1 antibody was obtained from Cell Signaling Technology (Danvers, MA). Anti-β-actin and anti-GAPDH antibodies were purchased from Santa Cruz Biotechnology (Santa Cruz, CA). TaqMan Assay probes for FoxO1, Bim, Puma, Fas ligand and GAPDH were purchased from Applied Biosystems (Foster City, CA). All culture media and supplements were bought from Sigma (St. Louis, MO). MT-2 immortalized human T-cells cells were obtained from the JCRB cell bank (Osaka, Japan). Human embryonic kidney 293T (HEK293T) cells were purchased from ATCC (Manassas, VA).
Cell culture
MT-2 cells were cultured in RPMI 1640 supplemented with 10% fetal bovine serum (FBS) and antibiotics at 37 °C in a 5% CO2 incubator. The MT-2 sub-lines to be generated – comprising CA1–3, CB1–3 and CR – were cultured with, respectively, 10 μg/ml chrysotile A, 10 μg/ml chrysotile B or 25 μg/ml crocidolite (Maeda et al. Citation2012). HEK293T cells were maintained in Dulbecco’s modified eagle medium supplemented with 10% FBS and antibiotics at 37 °C in a 5% CO2 incubator.
Plasmids
Human FoxO1 cDNA was isolated from a human heart cDNA library (Clontech, Mountain View, CA) by polymerase chain reaction (PCR), according to the registered sequence (GenBankNM_002015.3). The cDNA fragments corresponding to the FLAG epitope-tagged FoxO1 were generated with PCR and cloned into pMXs-puro retrovirus plasmid vector (Kitamura et al. Citation2003). The resultant plasmid was designated pMXs-FLAG-FoxO1. The sequence of the construct was confirmed by a di-deoxynucleotide chain-termination method using a DNA sequencing system 3100-Avant (Applied Biosystems). Lentivirus plasmid vectors pLKO.1-puro-Control and pLKO.1-puro containing shRNA targeting human FoxO1 (TRCN0000039579 and TRCN0000039580) were purchased from Sigma.
Western blot analysis
MT-2 cells were lysed in 20 mM Tris-HCl (pH 7.5) containing 1 mM EDTA, 1 mM EGTA, 10 mM 2-mercaptoethanol, 1% Triton X-100, 1% sodium deoxycholate, 0.1% sodium lauryl sulfate, 150 mM NaCl and 1% protease inhibitor cocktail (Sigma) and briefly sonicated. After centrifugation at 18 000 × g for 10 min, the supernatant was collected and measured using a BCA protein assay kit (Pierce, Rockford, IL). Cell lysate containing 50 μg protein was boiled in SDS-sample buffer and then subjected to SDS-PAGE separation. The resolved proteins were subsequently electrotransferred onto Immobilon P membranes (Millipore, Bedford, MA). After initial blocking with Tris-buffered saline containing 0.2% Tween 20 (TBS-T) supplemented with 5% BSA, membranes were then incubated with each primary antibody in TBS-T containing 1% BSA at dilutions recommended by the manufacturers for 1–2 h at room temperature (RT). Thereafter, the membrane was gently rinsed with TBS-T and then incubated with horseradish peroxidase-conjugated anti-mouse or anti-rabbit antibody secondary antibody in TBS-T at dilutions recommended by the manufacturers for 1 h at RT. After a final set of rinsing with TBS-T, the presence of the proteins of interest was evaluated using a chemiluminescence reaction mediated by an ECL Plus chemiluminescence detection kit (GE Healthcare, Little Chalfont, UK) and each was then visualized with Chemi-Stage (Toyobo, Osaka, Japan).
FoxO1 knockdown cell lines
Recombinant lentivirus was produced by transfection of lentivirus constructs with packaging plasmids pLP1, pLP2 and pLP/VSVG from a Block-it Lentiviral RNAi Expression System (Invitrogen, Carlsbad, CA) into HEK293T cells using Effectene transfection reagent (Qiagen, Hilden, Germany). Culture supernatants containing recombinant lentivirus were then harvested after 48–72 h. Growing MT-2 cells were infected with each recombinant lentivirus and the cells were then selected by culturing in the presence of 1 μg/ml puromycin (Sigma) for > 2 weeks at 37 °C. Resultant cell lines were designated Org-Ctrl (Scramble), Org-KD#1(TRCN0000039579) or Org-KD#2(TRCN0000039580).
Stable cell line expressing recombinant FoxO1
Recombinant retroviruses were produced by transfection of the retroviral constructs, pMXs-FLAG-FoxO1 or empty pMXs-puro, with packaging plasmids pCMV-VSV-G and pCMV-gag/pol into HEK293T cells using Effectene (Qiagen) transfection reagent. Culture supernatants containing recombinant retrovirus were harvested after 48–72 h. Growing MT-2CA1 cells were incubated with each recombinant retrovirus for 24 h and infected cells then selected by culture in the presence of 1 μg/ml puromycin for > 2 weeks at 37 °C. The established cells were then named CA1FoxO1 and CA1Empty, respectively.
Real-time RT-PCR analysis
Total RNA was isolated from growing MT-2 cells with an RNA purification Kit (Qiagen) and cDNA synthesized using a PrimeScript II 1st strand cDNA synthesis kit with an oligo-dT primer (Takara, Shiga, Japan). Real-time PCR analysis was performed with TaqMan probes and Brilliant III Ultra Fast QPCR master mix (Agilent Technologies, Palo Alto, CA) using a Mx3000P QPCR System (Agilent Technologies), according to manufacturer protocols.
Apoptosis assay
MT-2 cells were treated with chrysotile A for 24 h at 37 °C, then washed with ice-cold PBS and fixed in 70% ethanol. After staining with 40 μg/ml propidium iodide and treatment with 10 μg/ml RNase, the DNA content of cells was analyzed with a FACSCanto II (Becton Dickenson, Franklin Lakes, NJ) flow cytometer and FlowJo software (FlowJo, Ashland, OR). Apoptotic cells were quantified as the population of cells with subG1-DNA content. A minimum of 20 000 events/sample was acquired.
Statistical analyses
All experiments were performed in triplicate. Data were expressed as mean ± SD. Differences in FoxO1 mRNA levels in MT-2 org and MT-2 sub-lines were analyzed using a one-way analysis of variance (ANOVA) with a Dunnett’s multiple comparison test. Differences between two samples in expression of FoxO1 target genes or in the population of apoptotic cells were evaluated using a Student’s t-test. All analyses were performed with IBM SPSS 20 software (IBM, Armonk, NY). Statistical significance was defined at p < 0.05.
Results
Expression of FoxO1 mRNA and FoxO1 protein
The MT-2 cell sub-lines CA1–3, CB1–3 and CR were established after continuous exposure to three kinds of asbestos, namely, chrysotile A, chrysotile B or crocidolite, respectively (Hyodoh et al. Citation2005; Miura et al. Citation2006; Maeda et al. Citation2011, Citation2012). Those established sub-lines exhibited reduced apoptotic fractions when exposed to CA, CB or CR at a high concentration. Microarray gene expression analysis of MT-2CA1–3, CB1–3 and MT-2Org cells revealed 84 genes were up-regulated and 55 genes down-regulated in the MT-2 sub-lines exposed to asbestos. The current study focused on FoxO1 – a protein whose transcriptional activity is induced by reactive oxygen species and that regulates apoptosis in various cells (Accili & Arden Citation2004; Eijkelenboom & Burgering Citation2013) – which was reduced in MT-2 cells after continuous exposure to asbestos. The study initially examined expression of FoxO1 mRNA in cells using real-time RT-PCR (). The data showed that the amount of FoxO1 mRNA in all the MT-2 sub-lines exposed to asbestos was 20–30% that in MT-2Org cells and was in agreement with the results of the microarray analysis. Consistent with the expression of FoxO1 mRNA, the presence of the FoxO1 protein was down-regulated in MT-2 cells exposed to asbestos ().
Figure 1. Expression of FoxO1 in MT-2Org and MT-2Rst cells. (A) Total RNA was isolated from MT-2Org and MT-2Rst cells and amount of FoxO1 mRNA measured by real-time RT-PCR. The amount of FoxO1 mRNA in MT-2Org is shown as 100%. Data shown are means ± SD of three independent experiments. (B) Cell extracts of MT-2Org and MT-2Rst were analyzed by immunoblotting using anti-FoxO1 or anti-GAPDH antibodies. Data are representative of three independent experiments. Differences between MT-2Org and MT-2Rst sub-lines were analyzed using a Dunnett’s test.
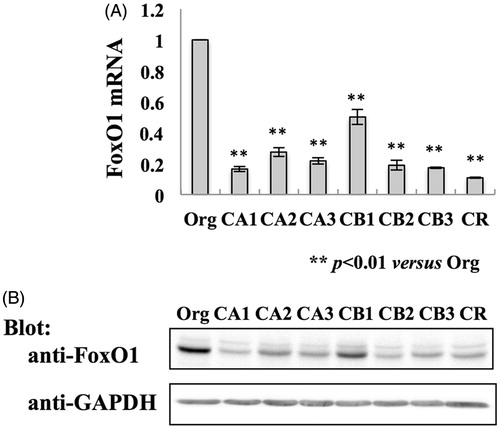
Down-regulation of FoxO1 target genes
This study also analyzed mRNA expression of FoxO1 target genes related to induction of apoptosis (). Puma and Bim – members of the Bcl-2 superfamily – have a pro-apoptotic function through binding to anti-apoptotic protein Bcl-2 or Bcl-xL (Puthalakath & Strasser Citation2002). Fas-ligand – a member of the TNF superfamily of cytokines – induces receptor-mediated apoptosis through binding to its receptor Fas (Nagata Citation1997). These genes have a FoxO1 binding sequence (i.e. Daf-16 binding element) on their promoter regions (Furuyama et al. Citation2000). In accordance with the reduction of FoxO1 in the MT-2Rst cells, the mRNA levels of these molecules were also decreased in the CA1 cells. These findings suggested that death signaling mediated by FoxO1 was suppressed in MT-2CA1 cells.
Figure 2. Expression of FoxO1 target genes. Total RNA was prepared from MT-2Org and MT-2CA1 cells and the amounts of Bim, Puma and Fas-ligand mRNA measured by real-time RT-PCR. Amounts shown are relative to those in MT-2Org cells. Data shown are means ± SD of three independent experiments. Differences between MT-2Org and MT-2CA1 were compared with using a Student’s t-test.
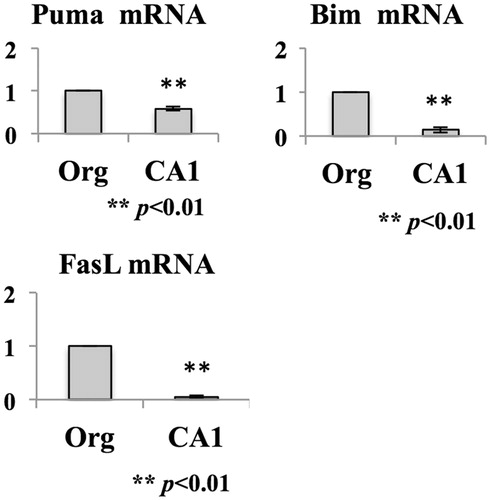
Knockdown of FoxO1 in MT-2Org cells
To investigate the effect of down-regulation of FoxO1 in MT-2 cells, FoxO1 knockdown cells were established by introducing lentivirus vector expressing shRNA targeting human FoxO1. The non-specific effects associated with RNA interference are well known; therefore, this study employed two different shRNA sequences targeting human FoxO1 as well as a scramble shRNA sequence as a control for knockdown of the FoxO1. As shown in , both of the shRNA targeting FoxO1 reduced the amount of FoxO1 protein in MT-2Org cells. To investigate the role of FoxO1 in regulation of apoptosis induced by chrysotile A, cells were treated with various concentrations of chrysotile A for 24 h. Knockdown of FoxO1 reduced the number of apoptotic cells after treatment with chrysotile A (25 μg/ml) to 50–70% of MT-2OrgCtrl cells; shRNA did not affect basal apoptotic levels. These results suggested FoxO1 played a role in regulation of apoptosis induced by chrysotile A in MT-2 cells.
Figure 3. Effect of FoxO1 knock-down on MT-2Org cells. (A) Extracts from MT-2OrgCtrl, MT-2OrgKD#1 and MT-2OrgKD#2 cells were analyzed by immunoblotting using anti-FoxO1 and anti-actin antibodies. Data are representative of three independent experiments. (B) MT-2OrgCtrl and MT-2KD cells were treated with 10 (grey bars) or 25 (black bars) μg/ml chrysotile A for 24 h. Apoptotic cells were measured using flow cytometry. Values shown are relative to those of apoptotic cells of MT-2Ctrl treated with 25 μg/ml chrysotile A. Data shown are means ± SD of three independent experiments. Differences between Ctrl and KD#1 or Ctrl and KD#2 cells treated with 25 μg/ml chrysotile A were analyzed using a Student’s t-test.
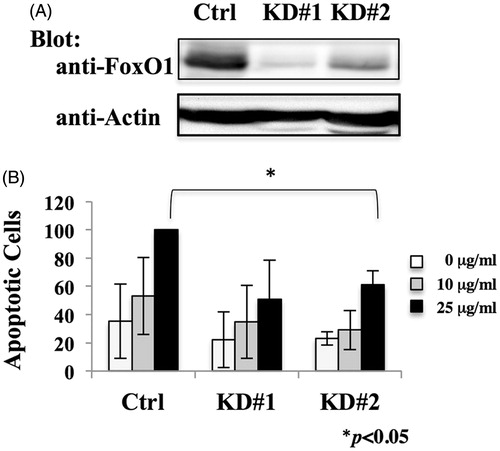
Expression of FoxO1 in MT-2 CA1 cells
To investigate the effect of recovery of FoxO1 expression in MT-2CA1 cells, MT-2CA1 cells were infected with retrovirus vector expressing FLAG-epitope tagged human FoxO1. The resultant cells express FLAG-FoxO1 protein () at a level above that of control MT-2CA1Empty cells. MT-2CA1FoxO1 cells express an amount of FoxO1 comparable to that of MT-2Org cells (data not shown). These cells were exposed to various concentrations of chrysotile A (). Expression of recombinant FoxO1 protein did not enhance the number of apoptotic cells or affect the basal level of apoptosis after treatments with chrysotile A at ≤ 25 μg/ml. This indicated that recovery of FoxO1 was not sufficient to cancel resistance to asbestos in MT-2CA1 cells. Therefore, expression of pro-apoptotic factor Puma, a target of FoxO1, was analyzed (). Expression of Puma mRNA increased in cells expressing recombinant FoxO1 above that in the MT-2CA1Empty cells, indicating FLAG-FoxO1 could function to induce target gene expression in MT-2CA1 cells. However, expression of anti-apoptotic factor Bcl-2 was not affected by FoxO1 expression. We previously reported that Bcl-2 was up-regulated in CA1 in a STAT3-dependent manner (Miura et al. Citation2006; Maeda et al. Citation2012). The findings here indicated that expression of Bcl-2 was independent of FoxO1, and that survival signaling via Bcl-2 could overcome death signaling induced by expression of FoxO1.
Figure 4. Effect of FoxO1 over-expression on MT-2CA1 cells. (A) Extracts of MT-CA1Empty and MT-2CA1FoxO1 cells were analyzed by immunoblotting using anti-FoxO1 and anti-actin antibodies. Data shown are representative of three independent experiments. (B) MT-CA1Empty and MT-2CA1FoxO1 cells were treated with 10 (grey bars) or 25 (black bars) μg/ml chrysotile A for 24 h. Control cells without treatment are shown as open bars. Apoptotic cells were measured using flow cytometry. Values shown are relative to those of apoptotic cells of MT-2Ctrl with 10 (grey bars) and 25 (black bars) μg/ml chrysotile A.
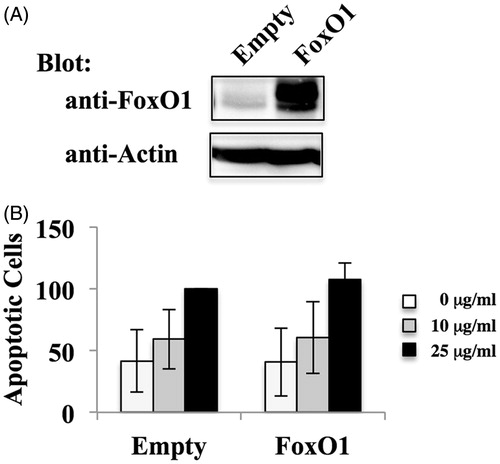
Figure 5. Expression of anti- or pro-apoptotic factors in MT-2CA1FoxO1. Total RNA was isolated from MT-2CA1Empty and MT-2CA1FoxO1 cells and the amount of Puma or Bcl-2 measured using real-time RT-PCR. Values shown are relative to the amount in MT-2CA1Empty cells. Differences between MT-2CA1Empty and MT-2CA1FoxO1 cells were analyzed using a Student’s t-test.
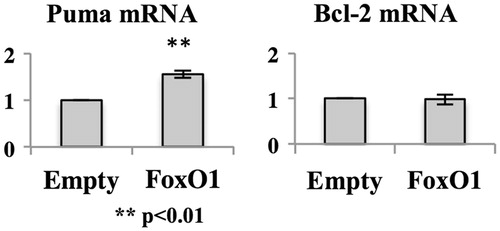
Discussion
It is well known that exposure to asbestos causes various diseases of the respiratory system including pulmonary fibrosis, asbestos-related pleural plaque (PP), lung cancer, and malignant pleural mesothelioma (MPM) (Lemen et al. Citation2006; Craighead et al. Citation2008). Mechanisms proposed for the cellular transformations caused by asbestos involve (1) iron-including asbestos fibers such as crocidolite and amosite causing ROS production in cells around the fibers, as well as apoptosis of alveolar macrophages following insufficient destruction of phagocytized fibers – all of which result in DNA damage in cells surrounding the fibers – and (2) physical DNA damage caused by the direct action of the incorporated fibers on chromatin. It was reported recently that chrysotile that does not include iron also induces iron overload in a rat model (Jiang et al. Citation2012). Furthermore, molecular analysis of MPM cells revealed several molecules implicated in the development of MPM. In particular, inactivation of the NF2/merlin signaling pathway caused by loss of NF2/merlin, LATS2 (Murakami et al. Citation2011) or up-regulation of YAP1 (Mizuno et al. Citation2012) in MPM cells. In addition, inactivation of p16/ink4a, inactivation of tumor suppressor PTEN, and somatic mutations of BRCA 1-associated protein-1 (BAP1) were also reported in MPM cells (Kratzke et al. Citation1995; Opitz et al. Citation2008; Bott et al. Citation2011; Testa et al. Citation2011). We have focused on the immunological effects of asbestos; our studies have shown that asbestos fibers suppressed NK and CTL cell functions (Nishimura et al. Citation2009a,Citationb; Matsuzaki et al. Citation2012; Kumagai-Takei et al. Citation2013).
MT-2 cells have been used as a CD4 T-cell model for chronic and continuous exposure to a low concentration of asbestos in human respiratory tissue. Studies with MT-2 cells revealed that asbestos fibers modified the characters of MT-2 cells, such as over-expression of Bcl-2, expression of cytokine production, reduction of chemokine C-X-C receptor-3 (CXCR3) and alteration of cytoskeletal molecules. In particular, over-expression of Bcl-2 was also found in CD4 T-cells isolated from PP or MPM patients (Miura et al. Citation2006). CXCR3 and interferon (IFN)-γ are basically expressed in activated T-helper (TH)-1-type cells, playing an important role in host-defense during immune surveillance against malignant cells (Rotondi et al. Citation2007). Similar results were obtained with ex vivo and in vivo analyses. Continuous exposure to asbestos reduced the expression of cell surface CXCR3 and IFNγ in CD4 T-cells freshly prepared from healthy donors. CD4 T-cells from PP or MPM patients express a lower amount of CXCR3 and IFNγ compared with those from healthy donors. These results supported the claim that the MT-2 cell is a good model for analyzing the effect of asbestos on human CD4 T-cells. On the other hand, it was reported that MT-2 cells express FoxP3, known as a master gene of the regulatory T (Treg) cell (Yagi et al. Citation2004; Ohkura et al. Citation2013).
Treg cells are designated as CD4+CD25+FoxP3+ T-cells that inactivate immune responses in part through the suppressive cytokines transforming growth factor (TGF)-β and IL-10. Treg cells are also thought to be involved in the development/progression of tumors, including MPM (Nishikawa & Sakaguchi Citation2010; Ireland et al. Citation2012). In particular, Treg cells are found in MPM tissues (Hegmans et al. Citation2006; Anraku et al. Citation2008; Shimizu et al. Citation2009); depletion of Treg cells with a neutralizing antibody induces rejection of malignant mesothelioma in mice (Hegmans et al. Citation2006). We previously reported that MT-2 cells produced TGFβ and IL-10 and inhibit the proliferation of effector cells in vitro (Maeda et al. Citation2008). Furthermore, it was shown that MT-2 sub-lines continuously exposed to asbestos produced more TGFβ and IL-10 than did parental cells. In agreement with those observations, the concentrations of TGFβ and IL-10 in the blood of PP or MPM patients was shown to be higher than in that of healthy donors (Maeda et al. Citation2008). It is possible exposure to asbestos enhanced production of suppressive cytokines by Treg cells in vivo.
The present study clearly showed that transcription factor FoxO1 was down-regulated in the MT-2Rst sub-lines (). Further, expression of pro-apoptotic factors regulated by FoxO1, i.e. Fas-ligand, Puma and Bim, were also suppressed in MT-2CA1 and that this outcome was consistent with reductions in cell levels of FoxO1 protein. Moreover, death signaling mediated by FoxO1 was suppressed by continuous exposure to asbestos in MT-2 cells and knockdown of FoxO1 in MT-2Org cells reduced the number of apoptotic cells induced by chrysotile A. It is known that FoxO1 regulates multiple target genes that control various cellular processes, such as apoptosis, metabolism and cell cycle progression (Eijkelenboom & Burgering Citation2013). Therefore, it is plausible that multiple FoxO1 target molecules and signaling pathways implicated in apoptosis were affected by knockdown or down-regulation of FoxO1 in the MT-2 cells. These results suggested to us that down-regulation of FoxO1 played an important role in the escape from apoptosis induced by asbestos in the MT-2 cells. On the other hand, over-expression of FoxO1 in MT-2CA1 cells did not alter expression of Bcl-2 or the number of apoptotic cells, indicating that expression of Bcl-2 was independent of FoxO1. These results suggested that both suppression of FoxO1 and over-expression of Bcl-2 contributed to MT-2CA1 cell resistance against asbestos-induced apoptosis. It is also possible down-regulation of FoxO1 affected various cellular processes – including cell cycle progression and metabolism – in addition to suppressing apoptosis. Further analysis is required to more fully evaluate the role of FoxO1 down-regulation induced by asbestos to gain a more complete understanding of mechanisms of effects of asbestos on T-cells.
Both FoxO1 and Bcl-2 are implicated in the general processes of apoptosis. Thus, MT-2 cells exposed to asbestos may have resistance to other natural apoptotic stimuli. Furthermore, asbestos fibers may induce ectopic viability to increase the number of Treg cells around the asbestos fibers. In addition to the findings in this study, FoxO1 is thought to suppress cell cycle progression (Eijkelenboom & Burgering Citation2013). Thus, it is possible that a loss of FoxO1 accelerated proliferation of MT-2 and Treg cells in vitro and in vivo.
The overall results here indicated that asbestos might activate Treg cell function through multiple pathways, ectopic viability, proliferation and cytokine production, as well as suppress tumor immunity. Further analyses are clearly needed required to characterize the overall chronic effect of asbestos on the immune system.
Conclusions
Our laboratory previously reported long-term exposure to asbestos altered immune cells in vivo and in vitro. Specifically, the human T-cell line MT-2 acquired resistance to a high concentration of asbestos after continuous exposure to low concentrations of asbestos in vitro. The present study clearly showed the effects of long-term exposure to asbestos on transcription factor FoxO1 in MT-2 cells. Namely, continuous exposure to asbestos reduced the expression of FoxO1 and its down-stream pro-apoptotic factors Bim, Puma and Fas ligand. Furthermore, knock-down of FoxO1 suppressed asbestos-induced apoptosis in the MT-2 cells. These results indicated FoxO1 regulated apoptosis induced by asbestos in MT-2 cells and down-regulation of FoxO1 signaling was implicated in the acquisition of resistance to asbestos after continuous exposure to asbestos in these cells. These findings might help to increase our understanding of the effect of asbestos on tumor immunity during the progression of malignant mesothelioma.
Acknowledegments
The authors thank Ms. Tamayo Hatayama and Shoko Yamamoto for their technical support. This study was supported by JSPS KAKENHI Grant Number 23890237 and 25860471 and Kawasaki Medical School Project Grants 23-6, 24-4 and 25-67.
Disclosure statement
The authors report no conflicts of interests. The authors alone are responsible for the content and writing of the paper.
References
- Accili D, Arden KC. 2004. FoxOs at the crossroads of cellular metabolism, differentiation, and transformation. Cell. 117:421–426.
- Anraku M, Cunningham KS, Yun Z, Tsao MS, Zhang L, Keshavjee S, Johnston MR, De Perrot M. 2008. Impact of tumor-infiltrating T-cells on survival in patients with malignant pleural mesothelioma. J Thoracic Cardiovasc Surg. 135:823–829.
- Bott M, Brevet M, Taylor BS, Shimizu S, Ito T, Wang L, Creaney J, Lake RA, Zakowski MF, Reva B, et al. 2011. The nuclear deubiquitinase BAP1 is commonly inactivated by somatic mutations and 3p21.1 losses in malignant pleural mesothelioma. Nat Genet. 43:668–672.
- Craighead JE, Craighead J, Gibbs A, Editors. 2008. Diseases associated with asbestos industrial products and environmental exposure. New York: Oxford University Press.
- Eijkelenboom A, Burgering BM. 2013. FOXOs: Signalling integrators for homeostasis maintenance. Nat Rev Mol Cell Biol. 14:83–97.
- Furuyama T, Nakazawa T, Nakano I, Mori N. 2000. Identification of the differential distribution patterns of mRNAs and consensus binding sequences for mouse DA-16 homologues. Biochem J. 349:629–634.
- Hegmans JP, Hemmes A, Hammad H, Boon L, Hoogsteden HC, Lambrecht BN. 2006. Mesothelioma environment comprises cytokines and T-regulatory cells that suppress immune responses. Eur Respir J. 27:1086–1095.
- Huang SX, Jaurand MC, Kamp DW, Whysner J, Hei TK. 2011. Role of mutagenicity in asbestos fiber-induced carcinogenicity and other diseases. J Toxicol Environ Health. 14:179–245.
- Hyodoh F, Takata-Tomokuni A, Miura Y, Sakaguchi H, Hatayama T, Hatada S, Katsuyama H, Matsuo Y, Otsuki T. 2005. Inhibitory effects of anti-oxidants on apoptosis of a human polyclonal T-cell line, MT-2, induced by an asbestos, chrysotile A. Scand J Immunol. 61:442–448.
- Ireland DJ, Kissick HT, Beilharz MW. 2012. The role of regulatory T-cells in meso-thelioma. Cancer Microenviron. 5:165–172.
- Izzi V, Masuelli L, Tresoldi I, Foti C, Modesti A, Bei R. 2012. Immunity and malignant mesothelioma: from mesothelial cell damage to tumor development and immune response-based therapies. Cancer Lett. 322:18–34.
- Jiang L, Akatsuka S, Nagai H, Chew SH, Ohara H, Okazaki Y, Yamashita Y, Yoshikawa Y, Yasui H, Ikuta K, et al. 2012. Iron overload signature in chrysotile-induced malignant mesothelioma. J Pathol. 228:366–377.
- Kitamura T, Koshino Y, Shibata F, Oki T, Nakajima H, Nosaka T, Kumagai H. 2003. Retrovirus-mediated gene transfer and expression cloning: Powerful tools in functional genomics. Exp Hematol. 31:1007–1014.
- Kohyama N, Shinohara Y, Suzuki Y. 1996. Mineral phases and some re-examined characteristics of the International Union Against Cancer standard asbestos samples. Am J Indust Med. 30:515–528.
- Kratzke RA, Otterson GA, Lincoln CE, Ewing S, Oie H, Geradts J, Kaye FJ. 1995. Immunohistochemical analysis of the p16INK4 cyclin-dependent kinase inhibitor in malignant mesothelioma. J Natl Cancer Inst. 87:1870–1875.
- Kumagai-Takei N, Nishimura Y, Maeda M, Hayashi H, Matsuzaki H, Lee S, Hiratsuka J, Otsuki T. 2013. Effect of asbestos exposure on differentiation of cytotoxic T-lymphocytes in mixed lymphocyte reaction of human peripheral blood mononuclear cells. Am J Respir Cell Mol Biol. 49:28–36.
- Lemen RA, Dodson R, Hammar S, editors. 2006. Epidemiology of asbestos-related diseases and the knowledge that led to what is known today. Boca Raton (FL): CRC Press.
- Maeda M, Miura Y, Nishimura Y, Murakami S, Hayashi H, Kumagai N, Hatayama T, Katoh M, Miyahara N, Yamamoto S, et al. 2008. Immunological changes in mesothelioma patients and their experimental detection. Clin Med Circ Respir Pulm Med. 2:11–17.
- Maeda M, Nishimura Y, Hayashi H, Kumagai N, Chen Y, Murakami S, Miura Y, Hiratsuka J, Kishimoto T, Otsuki T. 2011. Reduction of CXC chemokine receptor 3 in an in vitro model of continuous exposure to asbestos in a human T-cell line, MT-2. Am J Respir Cell Mol Biol. 45:470–479.
- Maeda M, Nishimura Y, Kumagai N, Hayashi H, Hatayama T, Katoh M, Miyahara N, Yamamoto S, Hirastuka J, Otsuki T. 2010. Dysregulation of the immune system caused by silica and asbestos. J Immunotoxicol. 7:268–278.
- Maeda M, Yamamoto S, Chen Y, Kumagai-Takei N, Hayashi H, Matsuzaki H, Lee S, Hatayama T, Miyahara N, Katoh M, et al. 2012. Resistance to asbestos-induced apoptosis with continuous exposure to crocidolite on a human T-cell. Sci Total Environ. 429:174–182.
- Matsuzaki H, Maeda M, Lee S, Nishimura Y, Kumagai-Takei N, Hayashi H, Yamamoto S, Hatayama T, Kojima Y, Tabata R, et al. 2012. Asbestos-induced cellular and molecular alteration of immunocompetent cells and their relationship with chronic inflammation and carcinogenesis. J Biomed Biotechnol. 2012:492608.
- Miura Y, Nishimura Y, Katsuyama H, Maeda M, Hayashi H, Dong M, Hyodoh F, Tomita M, Matsuo Y, Uesaka A, et al. 2006. Involvement of IL-10 and Bcl-2 in resistance against an asbestos-induced apoptosis of T-cells. Apoptosis. 11:1825–1835.
- Mizuno T, Murakami H, Fujii M, Ishiguro F, Tanaka I, Kondo Y, Akatsuka S, Toyokuni S, Yokoi K, Osada H, et al. 2012. YAP induces malignant mesothelioma cell proliferation by upregulating transcription of cell cycle-promoting genes. Oncogene. 31:5117–5122.
- Murakami H, Mizuno T, Taniguchi T, Fujii M, Ishiguro F, Fukui T, Akatsuka S, Horio Y, Hida T, Kondo Y, et al. 2011. LATS2 is a tumor suppressor gene of malignant mesothelioma. Cancer Res. 71:873–883.
- Nagata, S. 1997. Apoptosis by death factor. Cell. 88:355–365.
- Nishikawa H, Sakaguchi S. 2010. Regulatory T-cells in tumor immunity. Intl J Cancer. 127:759–767.
- Nishimura Y, Maeda M, Kumagai N, Hayashi H, Miura Y, Otsuki T. 2009a. Decrease in phosphorylation of ERK following decreased expression of NK cell-activating receptors in human NK cell line exposed to asbestos. Intl J Immunopathol Pharmacol. 22:879–888.
- Nishimura Y, Miura Y, Maeda M, Kumagai N, Murakami S, Hayashi H, Fukuoka K, Nakano T, Otsuki T. 2009b. Impairment in cytotoxicity and expression of NK cell-activating receptors on human NK cells following exposure to asbestos fibers. Intl J Immunopathol Pharmacol. 22:579–590.
- Ohkura N, Kitagawa Y, Sakaguchi S. 2013. Development and maintenance of regulatory T-cells. Immunity. 38:414–423.
- Opitz I, Soltermann A, Abaecherli M, Hinterberger M, Probst-Hensch N, Stahel R, Moch H, Weder W. 2008. PTEN expression is a strong predictor of survival in mesothelioma patients. Eur J Cardiothorac Surg. 33:502–506.
- Puthalakath H, Strasser A. 2002. Keeping killers on a tight leash: Transcriptional and post-translational control of the pro-apoptotic activity of BH3-only proteins. Cell Death Differ. 9:505–512.
- Robinson BW, Musk AW, Lake RA. 2005. Malignant mesothelioma. Lancet. 366:397–408.
- Roggli VL, Oury TD, Sporn TA, editors. 2004. Pathology of asbestos-associated diseases. New York: Springer Verlag.
- Rotondi M, Chiovato L, Romagnani S, Serio M, Romagnani P. 2007. Role of chemokines in endocrine autoimmune diseases. Endocr Rev. 28:492–520.
- Schreiber RD, Old LJ, Smyth MJ. 2011. Cancer immunoediting: integrating immunity's roles in cancer suppression and promotion. Science. 331:1565–1570.
- Shimizu Y, Dobashi K, Imai H, Sunaga N, Ono A, Sano T, Hikino T, Shimizu K, Tanaka S, Ishizuka T, et al. 2009. CXCR4+FoxP3+CD25+ lymphocytes accumulate in CXCL12-expressing malignant pleural mesothelioma. Intl J Immunopathol Pharmacol. 22:43–51.
- Testa JR, Cheung M, Pei J, Below JE, Tan Y, Sementino E, Cox NJ, Dogan AU, Pass HI, Trusa S, et al. 2011. Germline BAP1 mutations predispose to malignant mesothelioma. Nat Genet. 43:1022–1025.
- Toyokuni S. 2009. Role of iron in carcinogenesis: Cancer as a ferrotoxic disease. Cancer Sci. 100:9–16.
- Yagi H, Nomura T, Nakamura K, Yamazaki S, Kitawaki T, Hori S, Maeda M, Onodera M, Uchiyama T, Fujii S, et al. 2004. Crucial role of FoxP3 in the development and function of human CD25+CD4+ regulatory T-cells. Intl Immunol. 16:1643–1656.