Abstract
High contamination by aflatoxin B1 (AFB1) has been detected in Beja province (Tunisia) in many dairy products and animal feed, which has resulted in many tons of cereals and cereals being removed from the market, causing economic loss. While removal represents a means of reducing risk, exposures still occur. Studies have increasingly focused on means of AFB1 biodegradation/elimination using lactic acid bacteria and clay mineral. In the study here, Lactobacillus paracasei BEJ01 (LP) and montmorilonite clay (MT) were used to reduce the physio-/immunotoxicologic disorders that could develop in rats that underwent AFB1 exposures for a total of 7 consecutive days. The results indicated that rats treated with AFB1 (80 μg/kg BW) alone had significant decreases in lymphocytes in their blood (including B-lymphocytes, CD3+, CD4+, and CD8+ T-lymphocyte subtypes, and NK cells), immunoglobulins (IgA and IgG) and pro-inflammatory cytokines; these rats also had altered oxidative stress status. In contrast, in rats treated with LP + MT (2 × 109 cfu/ml [∼ 2 mg/kg] + 0.5 mg MT/kg BW) for a total of 7 days before, concurrent with or after AFB1 treatment, there was a significant blockade/mitigation of each AFB1-impacted parameter. Moreover, treatment with the mixture at any point in relation to AFB1 treatment expectedly caused enhanced TNFα and IL-1β expression relative to control values; all other parameters were comparable to values noted in control rats. Alone, the mixture had no impact on host parameters. From the results here it may be concluded the the LP + MT mixture was effective in protecting these hosts against AFB1-induced immunologic/physiologic disorders and that LP + MT could prevent and/or mitigate AFB1 toxicities in vivo.
Introduction
Climate change in the word as well as insufficient storage conditions in developing and non-developing countries have resulted in elevated levels of toxic mycotoxins entering the alimentary chain (Kuiper-Goodman et al. Citation1987; Smith et al. Citation1994). Increasingly, the removal of aflatoxin B1 (AFB1) from food and feed has been based on their interactions with food-grade bacteria and minerals, principally clays and lactic acid bacteria (El-Nezami et al. Citation2002; Abbès et al. Citation2008, Citation2012). To date, there is still only a limited understanding of the complex interactions between lactic acid bacteria and clay that give rise to the immobilization/removal of mycotoxins from food or feeds. A better understanding of how bacteria/clay influence the transport and fate of AFB1 is, therefore, needed, not only for the development of improved methods of food AFB1 decontamination, but to provide information about AFB1 risk assessment/abatement going forward.
AFB1 is a potent liver carcinogen in laboratory animals (Wogan Citation1992; Long et al. Citation2015). AFB1 has been implicated as an etiological factor, apart from hepatitis B virus, in development of human liver cancers (van Rensburg et al. Citation1985; more recently, Tanaka et al. Citation2015). In transgenic mice it was shown that the chronic liver damage due to over-expression of hepatitis B virus large peptide envelope acted synergistically with AFB1 in impacting neoplastic development (Sell et al. Citation1998).
The immunotoxic potential of AFB1 is also known in many species, including laboratory and domestic animals (Bondy & Pestka Citation2000). In pigs, AFB1 causes decreases in blastogenic responses to mitogen, reductions in complement titers, decreases in macrophage activation and depression of delayed hypersensitivity responses (Mocchegiani et al. Citation1998). Interestingly, in other studies using comparable doses, no depression of the proliferative responses of lymphocytes to mitogens was noted. Conflicting reports regarding the immunosuppressive effects of AFB1 might be explained in part by differences in experimental design and specific immune response(s) examined. Another explanation may be possibly different biological effects secondary to the applied AFB1 dosage. In fact, like other cytotoxic molecules with toxic effects at a certain dosage and immunomodulatory effects at a different dosage (Mihich Citation2007) the AFB1 might affect the function and cytokine release of immune cells in relation to the dosage, timing and schedule of administration.
Because of its detrimental effects, a number of strategies have been developed to prevent the growth of mycotoxigenic fungi, as well as to decontaminate and/or detoxify mycotoxin-contaminated foods and animal feeds. The use of many of the available physical and chemical methods for the detoxification of food products contaminated with mycotoxins is restricted due to problems concerning safety issues, possible losses in the nutritional quality of treated commodities, coupled with limited efficacy and cost implications (Bata & Lasztity Citation1999). This has led to the search for alternative strategies, including use of biological agents.
Lactic acid bacteria (LAB; lactobacilli and bifidobacteria), as part of the normal gut flora and consumption, are associated with a range of health benefits, including modulation of immune function and cancer prevention (Zhang et al. Citation2015). The literature has also provided a brief overview of mycotoxin detoxification using LAB (Fan et al. Citation2015). Along these lines, there has been increasing interest in a hypothesis that absorption of AFB1 in consumed food could be reduced by a variety of microorganisms in the gastrointestinal tract; consequently, numerous investigators showed that some dairy strains of LAB and bifidobacteria were able to bind effectively to AFB1 in buffered solution. Jebali et al. (Citation2014) also reported LAB fermentation could significantly reduce AFB1 concentration in PBS. However, such a reduction might not be sufficient for complete detoxification of the toxin.
Accordingly, in the present study, a mixture of L. paracasei BEJ01 (LP) and Tunisian montmorilonite clay (MT) was used to test how/if these materials could mitigate AFB1 toxicity in situ. Specifically, the aim of the study was to evaluate the potential for AFB1-binding LP and MT to prevent/mitigate effects of AFB1 on induction of inflammatory and/or immune response-related endpoints in rats.
Materials and methods
Chemicals and bacteria
Standard AFB1 (purity >98%) were obtained from Sigma (St. Louis, MO) and a stock solution was prepared in ethanol/water (1:1 [v/v]). Anti-CD3+, -CD4+, -CD8+, -CD56+ and B-cell (CD54) monoclonal antibodies (MAb) were each purchased from Serotec (Oxford, UK). All other chemicals purchased were of analytical grade.
For these studies, from the stock, a working solution of 80 μg AFB1/ml was prepared in phosphate-buffered saline (PBS, pH 6.5) for use in the in vivo study. All solutions were freshly prepared using sterile distilled water and held at 4 [± 1]°C until use. The bacterial strain used in the present study was Lactobacillus paracasei BEJ01, a lactic acid bacteria (LAB) isolated from 1-month-old artisanal butter made from cow milk from local Tunisian producers (Abbès et al. Citation2013). The Tunisian Montmorillonite (TM) was collected from Chemsi Mountain in Western Tunisia. The physico-chemical parameters of the TM have previously been described in detail by Abbès et al. (Citation2008).
Animals
Fisher rats (male, 6–7-weeks-of-age) were purchased from the Pasteur Institute of Tunis (Tunis, Tunisia). On arrival, the rats were housed in pathogen-free facilities maintained at 23 ± 1 °C, with a relative humidity of 55 ± 10% and a 12-h light:dark cycle. All rats had ad libitum access to filtered water and standard pellet diet (Vegetable fiber, 7.5%; protein, 14.5%; fat, 3% and metabolic energy, 0.017 MJ) that was pre-tested and confirmed to be mycotoxin-free. Male and not female rats were used in order to avoid potential confounding effects of female hormones/reproductive cycle interference on the studied parameters.
Experimental design
After a 1 week acclimatization period, rats were randomly allocated into six groups (10 rats/group; ). Rats in Group 1 were to receive saline by gavage daily (volume never exceeded 200 μl) for a total of 2 weeks; rats in Group 2 were to receive saline by gavage daily for Week 1 and then the LP + MT mixture daily for Week 2; rats in Group 3 were to receive saline by gavage daily for Week 1 and then AFB1 daily for Week 2; rats in Group 4 were to receive LP + MT by gavage daily for Week 1 and then AFB1 daily for Week 2 (pre-AFB1 exposure regimen); rats in Group 5 were to receive saline by gavage daily for Week 1 and then LP + MT and AFB1 daily for Week 2 (concurrent exposure regimen); and rats in Group 6 were to receive AFB1 by gavage daily for Week 1 and then LP + MT daily for Week 2 (post-AFB1 exposure regimen). A control group for Group 6, i.e. AFB1 by gavage daily for Week 1 and then saline daily for Week 2, was not examined here (as these types had already been examined in earlier pilot studies).
Table 1. Experimental design.
The selected doses of AFB1 (80 μg/kg BW) and of LP (2 × 109 cfu/ml ∼2 mg/kg) and MT (0.5 mg MT/kg BW) were based on previous work (Abbès et al. Citation2012, Citation2013, Citation2015). At 12 h after the final treatment (i.e. after end of Week 2 exposures), blood samples were collected from the retro-orbital venous plexus; from this material, both plasma and serum samples were derived and stored at −80 °C for later analyses of various end-points. The rats were then euthanized by ether asphyxiation to permit harvesting of spleen samples. All protocols here were performed in accordance with procedures that adhered to the European Community regulations controlling experiments in live animals (86/609).
Measures of total white and red blood cells and lymphocyte subtypes
Total white and red blood cells (WBC and RBC, respectively) were determined using a Coulter STKS blood counter (Coulter Electronics, Ltd., Luton, UK). To perform subtype analyses using 2-color flow cytometry, any erythrocytes present in 1-ml samples of heparinized blood/animal were first lysed with 0.02 ml FACSLysing solution (Becton Dickinson, San Jose, CA) for 10 min at 4 °C. Thereafter, dedicated aliquots of the blood were separately incubated for 20 min at room temperature with 0.05 ml of a suspension containing fluorescein isothiocyanate (FITC)- or phycoerythrin (PE)-conjugated MAb against CD4, CD8, CD54 or CD56 surface markers (Becton Dickinson) at levels of each MAb as recommended by the manufacturer. The samples were then analyzed on a FACScan flow cytometer (Becton Dickinson) equipped with Cellquest software. A minimum of 10 000 events/sample were analyzed. Specifically, lymphocytes (n = 2000) were measured and the percentage of positive cells in this population (gate) was assessed.
Immunoglobulin profile and plasma cytokines
Serum total IgA and IgG levels were measured via kinetic nephelometry (Roche Diagnostics, Barcelona, Spain). Plasma tumor necrosis factor (TNF)-α and interleukin (IL)-1β levels were measured using ELISA kits purchased from Biosource International (Camarillo, CA); the detection limit of these kits was each 4 pg/ml. The optical density of each sample was measured using a Spectra Image automatic plate reader (Tecan, Männedorf, Switzerland) at 450 nm. In each case, levels of a given cytokine were determined by extrapolation from a standard curve generated in parallel using kit-provided standards.
Caspase-3 enzyme activity determination
Caspase-3 activities in splenocytes – a measure of mycotoxin-induced apoptosis/DNA damage (rather than due to normal metabolic processes associated with splenocytes – as opposed to what might occur in thymus due to thymic lymphocyte selection processes) – and overall cell death were determined using the procedure of Kim et al. (Citation2000). In brief, splenocytes were harvested from portions of the isolated spleens by standard protocols. After lysing of red blood cells with 0.84% [w/v] ammonium chloride solution, the cells were re-pelleted, re-suspended in PBS (pH 7.4) and counted using a hemocytometer. Aliquots of the splenocytes (103 cells/ml) were then centrifuged at 5000 rpm and the pellet incubated in ice-cold lysing buffer (0.5 M EDTA, 5 M NaCl, 10% SDS), in 1 M Tris buffer (pH 7.4) for 10 min. The materials were then centrifuged at 5000 rpm for 20 min, supernatants (cell extracts containing caspase-3) were retrieved and protein concentrations were then determined using a BCA assay kit (Thermo Fisher Scientific, Rockford, IL).
Cytosolic extracts (1–2 mg/ml protein) in 10-μl aliquots were added to wells of 96-well flat-bottom microtitre plates. Thereafter, 50 μl substrate buffer (containing 100 mM HEPES (pH 7.5), 10% sucrose, 0.1% CHAPS, 5 mM dithiothreitol, 16 mM caspase-3 substrate (Ac-Asp-Glu-Val-Asp-p-nitroanalide [all Sigma]) was added and the plates were incubated for 3 h at 37 °C. In the presence of active caspase-3, release of pNA occurs, with the free pNA producing a yellow color that can be measured spectrophotometrically at 405 nm in the plate reader. Additional controls, free from cell lysates and others lacking substrate, were included in the assays. A standard curve was prepared to permit extrapolation of the sample pNA level. From this, results were then expressed as caspase-3 specific activity (pmol pNA/hr/mg protein).
Determination of anti-oxidative parameters
Several measures of host anti-oxidative status were determined using commercial diagnostic kits (Nanjing Jiancheng Biotechnology Co., Ltd, Jiangsu, China) and a Shimadzu UV-2410PC spectrophotometer (Shimadzu, Tokyo, Japan). The parameters evaluated in the blood were malondialdehyde (MDA) level, superoxide dismutase (SOD) activity, glutathione peroxidase (GHS-Px) activity, and total anti-oxidative capacity (T-AOC) (Liu et al. Citation1998).
Statistical analysis
All data are reported as mean ± SD. Data across treatment groups were analyzed using a one-way analysis of variance (ANOVA) and a Duncan’s multiple-range test. All analyses were performed using Stat-Packets Statistical Analysis software (Walonick Associates Inc., Minneapolis, MN).
Results
Total and differential WBC and subtype counts
Relative to in control rats, total WBC levels were increased in AFB1 alone-treated rats (). The differential counts of WBC revealed that, within this increase, there was a significant (p < 0.05) decrease in levels of lymphocytes and concomitant significant increases in the other cell types’ counts (eosinophils, neutrophils, monocytes) in these rats treated with AFB1 alone. On the other hand, total and differential counts of WBC in rats treated with the LP + MT mixture alone were comparable to values in the controls (p < 0.05). Pre- and co-treatment of AFB1-treated rats with the LP + MT mixture resulted in significant improvements (p < 0.05) in both total and individual WBC subtype counts. However, when the mixture was given over the 1 week after the AFB1 exposures, many of these values still differed from control rat levels (p < 0.05).
Table 2. Effect of treatments on total WBC and leukocyte counts in rats.
The effects of the LP + MT mixture and of AFB1 on T-lymphocyte subtypes and B-lymphocytes in the blood of the rats are presented in . Oral administration of the LP + MT mixture alone had no significant effects on any of the various lymphocyte phenol-types, illustrating its non-immunotoxic nature. In contrast, rats treated with AFB1 alone had significant (p < 0.05) reductions in CD3+, CD4+ and CD8+ T-lymphocyte, B-lymphocyte and NK counts. These changes were blocked/reversed in hosts treated with LP + MT with, critically, hosts receiving the treatment after AFB1 exposure having values back at control rat values.
Table 3. Effects of treatments on B- and T-lymphocyte subtypes in blood of rats.
Immunoglobulin profile
Data presented in show the effects of the various treatments on serum IgA and IgG levels. The results indicated that treatment with AFB1 alone induced a decrease (p < 0.05) in both isotypes. Conversely, treatment with the mixture LP + MT alone did not cause any perturbation in these levels, again suggesting no affect on host humoral immunity. The combined treatment of LP + MT before, concurrent with or after AFB1 caused a normalizing of these profiles.
Figure 1. IgA and IgG levels in rat blood after treatment. Rats were orally exposed daily, as indicated in Table 1. Plasma was collected from blood isolated 12 h after the final treatment. Data shown are means ± SD. *For each given isotype, the value was significantly different from control and all other treatment group values (p < 0.05).
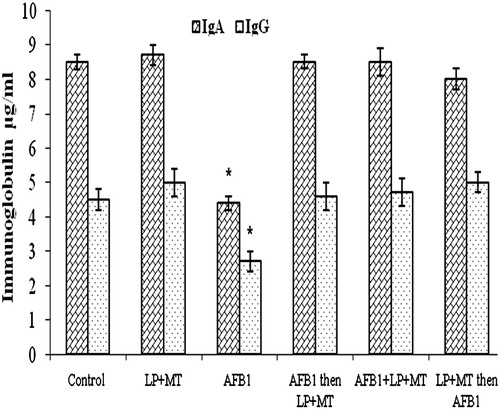
Plasma TNFα and IL-1β
Analysis of plasma from the treated rats showed that levels of TNFα were unaffected by the LP + MT mixture alone (). In AFB1-treated rats, TNFα levels were significantly (p < 0.05) lower than those in control rats. Oral administration of the mixture LP + MT resulted in remarkable increments in peripheral TNFα, with values superceding those of normal rats regardless of whether the LP + MT-treatment modality occurred before, concurrent with or after the AFB1 treatments. These exact same patterns were noted with respect to circulating IL-1β levels in these hosts.
Figure 2. TNFα and IL-1β in plasma from rats after treatment. Rats were orally exposed daily, as indicated in Table 1. Plasma was collected from blood isolated 12 h after final treatment. Data shown are means ± SD. *For each given cytokine, the value was significantly different from control and all other treatment group values (p < 0.05); ‡For each given cytokine, the value was significantly different from control, LP + MT only, and AFB1 only, but not from one another (p < 0.05).
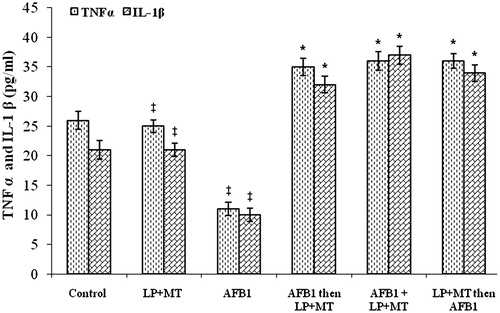
Splenocyte caspase-3 activity
The results of measures of splenocyte caspase-3 activity are presented in . There were no significant differences in activity between cells from rats treated with the mixture LP + MT alone (5.97 ±0.18 pmol pNA/h/mg protein) and those from the controls (5.91 ± 0.30). In contrast, splenocytes from rats that had received AFB1 alone had significant increases in activity to 51.45 ± 3.17 pmol pNA/h/mg protein. These levels were significantly reduced when the mixture LP + MT was used as a pre-, co- or post-treatment; values dropped to, respectively, 6.73 ± 0.12, 6.70 ± 1.94, and 6.93 ± 0.27 pmol pNA/h/mg protein.
Figure 3. Caspase-3 activity in splenocytes isolated from rats after treatment. Rats were orally exposed daily, as indicated in Table 1. Spleens were collected 12 h after the final treatment. Data shown are means ± SD. *Value significantly different from control and all other treatment group values (p < 0.05).
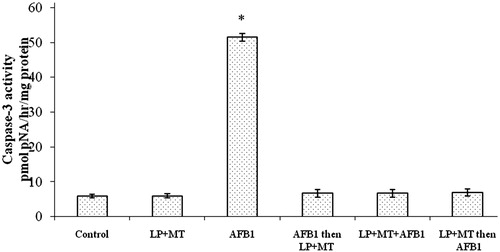
Anti-oxidative parameters
Several indicators related to oxidative stress in the treated rats, such as MDA, SOD, GSH and T-AOC, were measured in the harvested spleens. As shown in , AFB1 alone induced a significant increase in MDA levels and decreases in levels of SOD, GSH and T-AOC in the spleen. All of the changes in the measured oxidative stress-related parameters were blocked/reversed by pre-, co- or post-treatment of the rats with LP + MT.
Table 4. Effect of treatments on splenic oxidative stress enzymes.
Note to Readers: A control group for Group 6, i.e. AFB1 by gavage daily for Week 1 and then saline daily for Week 2, was not examined here (as such a group had already been examined in earlier pilot studies). Those studies showed that, at 12 h after the final treatment period (i.e. after end of Week 2 saline exposure following Week 1 AFB1 daily exposures), rats still displayed a significant level of AFB1-related toxicity. Specifically, body and lymphoid organ weights were significantly decreased in the AFB1-treated rats compared to in saline-only treated rats (data not shown). In addition, two rats (out of n = 10 to start) in the AFB1-only group died at the end of the second week of the regimen. This indicated there was no spontaneous amelioration of AFB1-induced effects in the rats due to a mere period of 7 days post-exposure to the toxicant.
Discussion
Aflatoxin B1 (AFB1), the most hazardous mycotoxin, is extremely toxic, mutagenic and carcinogenic (Bhatnagar et al. Citation2003; Council for Agricultural Science and Technology Citation2003). AFB1 poses a severe threat to both livestock productivity and human health, thus resulting in huge economic losses worldwide each year (Marisa & Das Citation2003; Diaz Citation2005). Interest in biological detoxification of AFB1 has greatly increased during the past decade. Several fungal species have been found to be able to biotransform AFB1 into less toxic metabolites; such fungi include Pleurotus ostreatus (Motomura et al. Citation2003) and Armillariella tabescens (Liu et al. Citation1998). The biotransformation activities of these fungi were mainly demonstrated with cell extracts. However, practical applications of these fungi may be limited by factors such as long incubation time required for the detoxification and complicated procedures needed for obtaining the active extracts.
Reduction of AFB1 by bacteria has also been reported; most of the published studies focused on lactic acid bacteria, such as strains belonging to Lactobacillus (El-Nezami et al. Citation1998; Gratz et al. Citation2005), Bifidobacterium (Peltonen et al. Citation2001), Propionibacterium (El-Nezami et al. Citation2000) and Lactococcus (Pierides et al. Citation2000). However, the AFB1 reduction by these bacteria was proven to be mainly by cell binding rather than biotransformation. Most importantly, this kind of binding seemed reversible, which meant that AFB1 could potentially not be removed completely from contaminated media.
Recently, it was shown that an isolate bacterium Lactobacillus plantarum MON03 and L. paracasei BEJ01 from Tunisian artisanal butter was able to bind AFB1 and AFM1 in vitro and in vivo (Jebali et al. Citation2014; Abbès et al. Citation2016). Similarly, in a previous study, it was demonstrated that Tunisian Montmorillonite (TM) was able to remove several forms of aflatoxin from contaminated food and foodstuffs (Abbès et al. Citation2008). Although currently-used technologies in detoxification of AFB1 seem satisfactory, establishing newer methods and/or strategies as effective decontamination paradigms remain of critical importance. Accordingly, the aim of this study was to determine the abilities of a composite of living L. paracasei BEJ01 (LP) cells and TM clay to prevent AFB1-induced immunotoxicity disorders in rat hosts without imparting any untoward effects of their own.
The results of the current study indicated AFB1 induced reductions in peripheral lymphocytes typical to those reported previously (Berek et al. Citation2001; Abbès et al. Citation2006) and that AFB1 induced immunosuppressive effects via depressing the activity/number of T- and B-lymphocytes in the hosts. Past searches for underlying mechanisms revealed that apoptosis was a key event, since there was clear evidence of AFB1-induced immune cell death in the spleen (Abbès et al. Citation2010; Jebali et al. Citation2014). As the spleen is an important organ for maturing lymphocytes, the deaths of lymphocytes in the spleen would consequently reduce levels of circulating peripheral lymphocytes (Zhang et al. Citation2004).
In the current study, it was seen that the lymphocyte phenotype counts were greatly reduced by AFB1 treatment. The decreased expressions of CD3+, CD4+, CD8+ T-lymphocytes, NK cells and B-lymphocytes in the blood were clear markers of the immunosuppressive impact of AFB1. These effects on the rat immune system were in accordance with those reported by Pestka et al. (Citation1987). Along these lines, Berek et al. (Citation2001) stated that mycotoxins induced immunosuppression by depressing T- and B-lymphocyte activity. Moreover, Swamy et al. (Citation2004) showed that mycotoxin-contaminated diets caused reduced B-lymphocyte counts in broiler chickens. This progressive loss of CD3+, CD4+, CD8+ and NK cell numbers has been related to many factors such as reduced interferon (IFN)-γ and interleukin (IL)-2 expression and the added presence of immunosuppressive factors (mainly prostaglandin E2) produced by suppressor cells in the spleen (Segura et al. Citation2000). The current results also support the findings of Karagezian (Citation2000), who reported that a single intravenous dosing of zearalenone mycotoxin to rats led to formation of pronounced abnormalities in lymphocyte membrane metabolism.
The increased number of total WBC and granulocytes and reductions in expression of key inflammatory cytokines (e.g. TNFα and IL-1β) after AFB1 treatment noted here were in line with observations in our previous work (see Abbès et al. Citation2010). Moreover, the decrease in both serum IgA and IgG levels in these AFB1-treated rats corresponded with known effects of the toxin on host cellular and humoral immune systems. Indeed, the changes in B-lymphocyte levels and immunoglobulin production after AFB1 treatment could be a result of the decreased number and altered functions of T-lymphocytes caused by the mycotoxin, based on the findings reported in Lim et al. (Citation2005). Lastly, the results here showing that levels of lipid peroxidation (MDA) were increased and those of GSH, SOD and T-AOC decreased in the spleen due to AFB1 – each markers of oxidative damage/stress – were in accordance with earlier findings of effects of the mycotoxin on oxidative stress in situ (Hassan et al. Citation2014).
That use of the LP + MT mixture was able to block, mitigate and/or reverse the effects of the AFB1, regardless of timing, in relation to the toxin exposure was very interesting. The protective effects of LP and the MT could be explained by the ability of this bacterium and the clay to bind AFB1 in vitro or in the gastrointestinal tract and, thereby, reduce its bioavailability (Abbès et al. Citation2010). Many microorganisms, e.g. bacteria, yeasts, molds, are able to remove or degrade small amounts of mycotoxins in foodstuffs (Styriak et al. Citation2001). Results of several studies suggest that binding is a major mechanism of detoxification by the organisms (Gratz Citation2007). Strains L. rhamnosus GG and LC-705 seem to be most effective in such detoxifications (Lahtinen et al. Citation2004). However, the binding mechanism itself still remains not understood. It had been suggested that carbohydrate-rich mannoproteins or glucans might be involved in the binding, as levels of complex formation appear to be strain-and toxin-specific (Shetty & Jespersen Citation2006). Raju and Devegowda (Citation2000) attributed the binding of aflatoxins by yeast cell walls to mannan oligosaccharides, while toxin binding was attributed to glucan components (Yiannikouris et al. Citation2004). Haskard et al. (Citation2001) suggested that aflatoxins bind predominantly to carbohydrate and protein components of cells. Clearly, systematic studies are still needed to understand the precise binding mechanisms.
Many clay minerals, e.g. smectite, hydrated sodium/calcium and montmorillonite clay, are also able to “remove” small amounts of mycotoxins from foodstuffs (Styriak et al. Citation2001). Bursian et al. (Citation1992) showed that addition of clay to a diet protected mink against reproductive defects induced by mycotoxins. Further, as demonstrated in our previous study, LP was efficacious in abrogating zearalenone toxicity. The mechanism by which the clay could impact against AFB1 is also still misunderstood. It may be due to a presence of various types of active binding sites on/in the clay (Hosono et al. Citation1990; Philips et al. Citation1995).
There are several possible mechanisms of action to explain the present findings. Under the current test conditions, sites located on the surface/edges of the clay particles could be saturated by the AFB1 wherein the toxin is linked at basal planes in inter-layer channels and so produce a stable complex that leads to reduce bioavailability of the AFB1 to local cells (and, overall, systemically as well). Even in a scenario wherein bacteria are present that are already binding AFB1, these same molecules would bind the AFB1-bacteria complex (assuming of course both the LP and MT binding molecules are not stoichemically competing for the same portion of AFB1 entity), this too would lead to decreased AFB1 bioavailability/toxicity. It is also plausible that bacteria can adhere to enterocytes in the small intestine and then generate a biofilm that protects against AFB1 contact with local cells. In addition, some bacteria can produce bactericides that also might facilitate AFB1 elimination (Wang et al. Citation2015). Clearly, the exact mechanisms remain to be determined to explain how the LP and MT, moreover in concert, are acting in situ to mitigate AFB1 toxicity. It would also be important to determine if these mechanisms have a general applicability against other toxins (fungal-based or not) so that the potential utility of this combined treatment could be expanded.
Future studies are essential to ascertain the exact molecules implicated in mycotoxin mitigation/elimination by the bacteria (and associated products) or the MT, as well as the reduction of potential effects of such toxin(s) on the gastrointestinal/general immune system. For example, it will be interesting to examine the molecules with regard to contact with the toxin and then determine which is dominant to achieve the current observed effects, LP or MT (by assessing level of molecule expression and/or location or availability of the molecule on the LP vs on the MT).
Conclusion
Results of the present study demonstrated that AFB1 affected the oxidative status in rats, down-regulated B- and T-lymphocyte levels and negatively impacted on the expression of several key inflammation-regulating cytokines. Pre-, co- or post-treatment using a mixture of L. paracasei BEJ01 plus MT imparted protective effects against these AFB1 (immuno-) toxicities. Thus, use of a mixture of LP + MT could potentially provide an option for use in protecting against immunotoxicities in many farm animal species that face inadvertent exposure to this class of mycotoxins in their feedstuff.
Acknowledgments
The work was supported by the Tunisian Ministry of Higher Education and Scientific Research (Unit of Immunology, Environmental Microbiology, and Cancerology), the Higher Institute of Biotechnology of Beja (Animal Biotechnology Department) and The National institute of Genetic Engineering and Biotechnology of Tehran.
Disclosure statement
The authors report no conflicts of interest. The authors alone are responsible for the content and writing of the paper.
References
- Abbès S, Ben Salah-Abbès J, Ouanes Z,Hetta MM, Ibrahim M, Bacha H, Abdel-Wahhab MA, Oueslati R. 2008. Efficacy of Tunisian montmorillonite to bind Aflatoxin in vitro and to ameliorate the physiological alteration in vivo. Appl Clay Sci. 42:151–157.
- Abbès S, Ben Salah-Abbès J, Jebali R, Ben Younes R, Oueslati R. 2015. Interaction of AFB1 and fumonisin B1 in mice causes immunotoxicity and oxidative stress: Possible protective role using lactic acid bacteria. J Immunotoxicol. 14:1–9.
- Abbès S, Ben Salah-Abbès J, Ouanes Z, Houas Z, Othman O, Bacha H, Abdel-Wahhab MA, Oueslati R. 2006. Preventive role of phyllosilicate clay on immunological and biochemical toxicity of zearalenone in Balb/c mice. Intl Immunopharmacol. 6:1251–1258.
- Abbès S, Ben Salah-Abbès J, Sharafi H, Akbari Noghabi K, Oueslati R. 2012. Inter-action of Lactobacillus plantarum MON03 with Tunisian Montmorillonite clay and ability of the composite to immobilize Zearalenone in vitro and counteract immunotoxicity in vivo. Immunopharmacol Immunotoxicol. 34:944–950.
- Abbès S, Ben Salah-Abbès J, Sharafi H, Oueslati R, Noghabi KA. 2013. Lactobacillus paracasei BEJ01 prevents immunotoxic effects during chronic zearalenone exposure in Balb/c mice. Immunopharmacol Immunotoxicol. 35:341–348.
- Abbès S, Ben Salah-Abbès J, Jebali R, Ben Younes R, Oueslati R. 2016. Interaction of aflatoxin B1 and fumonisin B1 in mice causes immunotoxicity and oxidative stress: possible protective role using lactic acid bacteria. J Immunotoxicol. 13:46–54.
- Abbès S, Ben Salah-Abbès J, Abdel-Wahhab MA, Oueslati R. 2010. Immunotoxicological and biochemical effects of aflatoxins in rats prevented by Tunisian Montmorillonite with reference to HSCAS. J Immunopharmacol Immunotoxicol. 32:514–522.
- Bata A, Lasztity R. 1999. Detoxification of mycotoxin-contaminated food and feed by microorganisms. Trends Food Sci Techol. 10:223–228.
- Berek L, Petri IB, Mesterhazy A, Teren J, Molanr J. 2001. Effects of mycotoxins on human immune functions in vitro. Toxicol in Vitro 15:25–30.
- Bhatnagar D, Ehrlich KC, Cleveland TE. 2003. Molecular genetic analysis and regulation of aflatoxin biosynthesis. Appl Microbiol Biotechnol. 61:83–93.
- Bondy G, Pestka JJ. 2000. Immunomodulation by fungal toxins. J Toxicol Environ Health. 3:109–143.
- Bursian SJ, Aulerich RJ, Cameron JK, Ames NK, Steficek BA. 1992. Efficacy of hydrated sodium calcium alumino silicate in reducing the toxicity of dietary zearalenone to mink. J Appl Toxicol. 12:85–90.
- Council for Agricultural Science and Technology. 2003. Mycotoxins: Risks in plant, animal, and human systems. Ames (IA): Council for Agricultural Science and Technology.
- Diaz DE, editor. 2005. The Mycotoxin Blue Book. Nottingham (UK): Nottingham University Press.
- El-Nezami H, Kankaanpaa P, Salminen S, Ahokas J. 1998. Ability of dairy strains of lactic acid bacteria to bind a common food carcinogen, AFB1. Food Chem Toxicol. 36:321–326.
- El-Nezami H, Mykkanen H, Kankaanpaa P, Salminen S, Ahokas J. 2000. Ability of Lactobacillus and Propionibacterium strains to remove AFB1 from chicken duodenum. J Food Protect. 63:549–552.
- El-Nezami HS, Chrevatidis A, Auriola S, Salminen S, Mykkanen H. 2002. Removal of common Fusarium toxins in vitro by strains of Lactobacillus and Propionibacterium. Food Addit Contam. 19:680–686.
- Fan Y, Zhao L, Ji C, Li X, Jia R, Xi L, Zhang J, Ma Q. 2015. Protective effects of Bacillus subtilis ANSB060 on serum biochemistry, histopathological changes and anti-oxidant enzyme activities of broilers fed moldy peanut meal naturally contaminated with aflatoxins. Toxins. 7:3330–3343.
- Gratz S. 2007. Aflatoxin binding by probiotics. Experimental studies on intestinal aflatoxin transport, metabolism and toxicity. Doctoral dissertation, School of Public Health and Clinical Nutrition, Clinical Nutrition and Food Health Research Centre, University of Kuopio, Finland.
- Gratz S, Mykkanen H, El-Nezami H. 2005. Aflatoxin B1 binding by a mixture Lactobacillus of and Propionibacterium: In vitro vs. ex vivo. J Food Prot. 68:2470–2474.
- Haskard C, El-Nezami H, Kankaanpaa PE, Salminen SE, Ahokas JT. 2001. Surface binding of AFB1 by lactic acid bacteria. Appl Environ Microbiol. 67:2086–3091.
- Hassan A, Abdel-Aziem S, El-Nekeety A, Abdel-Wahhab M. 2014. Panax ginseng extract modulates oxidative stress, DNA fragmentation and up-regulates gene expression in rats subchronically treated with AFB1 and fumonisin B1. Cytotechnology. 5:861–871.
- Hosono A, Tanabe T, Otani H. 1990. Binding properties of lactic acid bacteria isolates from kefir milk with mutagenic amino acid pyrolyzates. Milchwissenschaft. 45:647–651.
- Jebali R, Abbès S, Ben Salah-Abbès J, Ben Younes R, Haouas Z, Oueslati R. 2014. Ability of Lactobacillus plantarum MON03 to mitigate Aflatoxins (B1 and M1) immunotoxicities in mice. J Immunotoxicol. 12:290–299.
- Karagezian MK. 2000. Effect of the mycotoxin zearalenone on the metabolism of membrane phospholipids of rat lymphocytes. Ukr Biokhim Zh. 72:105–109.
- Kim HS, Hausman DB, Compton MM, Dean RG, Martin RJ, Hausman GJ, Hartzell DL, Baile CA. 2000. Induction of apoptosis by all-trans-retinoic acid and C2-ceramide treatment in rat stromal-vascular cultures. Biochem Biophys Res Commun. 270:76–80.
- Kuiper-Goodman T, Scott PM, Watanabe H. 1987. Risk assessment of the mycotoxin zearalenone. Regul Toxicol Pharmacol. 7:253–306.
- Lahtinen SJ, Haskard CA, Ouwehand AC, Salminen SJ, Ahokas JT. 2004. Binding of aflatoxin B1 to cell wall components of Lactobacillus rhamnosus strain GG. Food Addit Contam. 21:158–164.
- Lim HW, Hillsamer P, Banham AH, Kim CH. 2005. Direct suppression of B-cells by CD4+CD25+ regulatory T-cells. J Immunol. 175:4180–4183.
- Liu DL, Yao DS, Liang R, Ma L, Cheng WQ, Gu LQ. 1998. Detoxification of AFB1 by enzymes isolated from Armillariella tabescens. Food Chem Toxicol. 36:563–574.
- Long XD, Huang HD, Huang XY, Yao JG, Xia Q. 2015. XPC codon 939 poly-morphism is associated with susceptibility to DNA damage induced by AFB1 exposure. Intl J Clin Exp Med. 8:1197–204.
- Marisa HN, Das C. 2003. A review on biological control and metabolism of aflatoxin. Crit Rev Food Sci. 43:245–264.
- Mihich E. 2007. Anti-cancer drug-induced immunomodulation and cancer therapeutics. Curr Cancer Ther Rev. 3:174–193
- Mocchegiani E, Corradi A, Santarelli L, Tibaldi A, DeAngelis E, Borghetti P, Bonomi A, Fabris N, Cabassi E. 1998. Zinc, thymic endocrine activity and mitogen responsiveness (PHA) in piglets exposed to maternal aflatoxicosis B1 and G1. Vet Immunol Immunopathol. 62:245–260.
- Motomura M, Toyomasu T, Mizuno K, Shinozawa T. 2003. Purification and characterization of aflatoxin degradation enzyme from Pleurotus ostreatus. Microbiol Res.158:237–242.
- Peltonen K, El-Nezami H, Haskard C, Ahogas J, Salminen S. 2001. AFB1 binding by dairy strains of lactic acid bacteria and bifidobacteria. J Dairy Sci. 84:2152–2156.
- Pestka JJ, Tai JH, Witt MF, Dixon DE, Forsell JH. 1987. Suppression of immune response in the B6C3F1 mouse after dietary exposure to Fusarium mycotoxins deoxynivalenol (vomitoxin) and zearalenone. Food Chem Toxicol. 25:297–304.
- Philips TD, Sarr AB, Grand PG. 1995. Selective chemisorption and detoxification of aflatoxins by phyllosilicate clay. Nat Toxins 3:204–213.
- Pierides M, El-Nezami H, Peltonen K, Salminen S, Ahokas J. 2000. Ability of dairy strains of lactic acid bacteria to bind AFM1 in a food model. J Food Protect. 63:645–650.
- Raju M, Devegowda G. 2000. Influence of esterified glucomannan on performance and organ morphology, serum biochemistry and hematology in broiler exposed to individual and combined mycotoxicosis (aflatoxin, ochratoxin, and T-2 toxin). Br Poult Sci. 41:640–650.
- Segura JA, Barbero LG, Marques J. 2000. Ehrlich ascites tumor unbalances splenic populations and reduces responsiveness of T-cells to Staphylococcus aureus endotoxin B stimulation. Immunol Lett. 74:111–115.
- Sell S, Xu KL, Huff WE, Kabena LF, Harvery RB, Dunsford HA. 1998. Aflatoxin exposure produces serum α-fetoprotein elevations and marked oval cell proliferation in young male Peking ducklings. Pathology. 30:34–39.
- Shetty P, Jespersen L. 2006. Saccharomyces cerevisiae and lactic acid bacteria as potential mycotoxin decontaminating agents. Trends Food Sci Technol. 17:48–55.
- Smith JE, Lewis CW, Anderson JG, Solomons GL. 1994. Mycotoxins in human nutrition and health. European Commission Directorate: General XII for science, research and development; EUR 16048 EN.
- Styriak I, Conková E, Kmet V, Böhm J, Razzazi E. 2001. Use of yeast for microbial degradation of some selected mycotoxins. Mycotoxin Res. 17:24–27.
- Swamy HV, Smith TK, Karrow NA, Boermans HJ. 2004. Effects of feeding blends of grains naturally contaminated with Fusarium mycotoxins on growth and immunological parameters of broiler chickens. Poult Sci. 83:533–543.
- Tanaka T, Mizukami S, Hasegawa-Baba Y, Onda N, Sugita-Konishi Y, Yoshida T, Shibutani M. 2015. Developmental exposure of AFB1 reversibly affects hippocampal neurogenesis targeting late-stage neural progenitor cells through suppression of cholinergic signaling in rats. Toxicology. 2:59–69.
- van Rensburg SJ, Cook-Mozaffari P, van Schalkwyk DJ, van der Watt JJ, Purchase IF. 1985. Hepatocellular carcinoma and dietary aflatoxin in Mozambique and Transkei. Br J Cancer. 51:713–726.
- Wang J, Tang L, Glenn TC, Wang JS. 2015. Aflatoxin B1-induced compositional changes in gut microbial communities of male F344 rats. Toxicol Sci. doi: 10.1093/toxsci/kfv259.
- Wogan GN. 1992. Aflatoxin as risk factors for hepatocellualar carcinoma in humans. Cancer Res. 52:2114–2118.
- Yiannikouris A, Francois J, Poughon L, Dussap CG, Bertin G, Jeminet G, Jouany JP. 2004. Adsorption of zearalenone by β-D-glucans in the Saccharomyces cerevisiae cell wall. J Food Protect. 67:1195–1200.
- Zhang M, Fan X, Fang B, Zhu C, Zhu J, Ren F. 2015. Effects of Lactobacillus salivarius Ren on cancer prevention and intestinal microbiota in 1,2-dimethylhydrazine-induced rat model. J Microbiol. 53:398–405.
- Zhang S, Wang Q, Li WF, Wang HY, Zhang HJ. 2004. Enhanced anti-tumor immunity by murine cytokine activated T-lymphocytes after co-cultured with bone marrow derived dendritic cells pulsed with whole tumor lysates. Leukemia Res. 28:1085–1088.