Abstract
Respiratory allergy triggered by exposure to environmental chemical allergen is a serious problem in many Asian countries and has the potential to cause severe health problems. Here, we aimed to elucidate the pathogenic mechanisms of this disease and develop an in vivo detection method for respiratory allergy induced by environmental chemical allergen. Both BALB/c and NC/Nga mice were sensitized topically for 3 weeks and were then subjected to inhalation challenge with pulverized trimellitic anhydride into particles measuring 2-μm in diameter. On the day after the final challenge, all mice were sacrificed, and IgE levels, immunocyte counts, and cytokine levels in the serum, hilar lymph nodes, and bronchoalveolar lavage fluid were measured. We also monitored the expression of genes encoding pro-inflammatory cytokines in the lung. We found that all endpoints were significantly increased in mice of both strains subjected to trimellitic anhydride inhalation as compared with the respective control groups. However, worsening of respiratory status was noted only in NC/Nga mice. Interestingly, type 2 helper T-cell reactions were significantly increased in BALB/c mice compared with that in NC/Nga mice. In contrast, the number of mast cells, levels of mast cell-related cytokine/chemokines, and production of histamine in NC/Nga mice were significantly higher than those in BALB/c mice. Thus, environmental chemical allergen induced respiratory allergy in NC/Nga mice in terms of functional and inflammatory symptoms. Furthermore, mast cells may be involved in the aggravation of airway allergic symptoms induced by environmental chemical allergens.
Introduction
Owing to the increasing use of many types of chemical substances worldwide, chemical-induced allergies have emerged as a major public health problem (Howarth Citation1998). In particular, the inhalation of compounds associated with fine particulate matter (i.e. PM2.5; <2.5 μm aero-dynamic diameter) is a leading cause of allergic respiratory diseases (Brown & Jason Citation2007; Jeebhay & Quirce Citation2007). Moreover, compounds associated with PM2.5 aggravate respiratory responses to common allergens (Gavett et al. Citation2003; Janssen et al. Citation2003), and some chemicals such as trimellitic anhydride, diphenylmethane-4,4′-diisocyanate (MDI), and glutaraldehyde can induce obvious symptoms of respiratory inflammation, such as asthma (Rattray et al. Citation1994; Pauluhn et al. Citation2005; Fukuyama et al. Citation2008; Nishino et al. Citation2014). However, the exact mechanisms of these chemical-induced respiratory allergic disorders are not fully understood (Rothen-Rutishauser et al. Citation2005; Kimber et al. 2008).
To detect respiratory allergy induced by environmental chemicals, several detection methods have been developed using cell line and animal models. Agius et al. (Citation1991) reported that some chemical structure–activity relationships are associated with induction or aggravation of respiratory allergic responses; thus, it is possible to identify causative agents of respiratory allergy from other structurally related chemicals that are incapable of causing respiratory allergy. However, there is insufficient information available to identify respiratory sensitization potentials from analysis of structure alone.
Alternatively, serum IgE test and cytokine fingerprinting are commonly used in vivo detection methods for respiratory allergy. The total serum IgE test is based on the increase in total serum IgE levels observed during development of respiratory allergy (Kimber & Dearman Citation2002). Mice are sensitized dermally with a chemical allergen, and total serum IgE levels are measured at 14 d after the first chemical sensitization. If mice are exposed to respiratory allergic agents, total serum IgE levels will be significantly increased in a dose-dependent manner (Dearman et al. Citation1992). Cytokine fingerprinting is also based on a dichotomy in T-helper (TH)-1 and TH2 cell responses; respiratory allergens induce increases in the expression of various cytokines, including interleukin (IL)-4, -5, -10, and -13, whereas contact allergens induce interferon (IFN)-γ and tumor necrosis factor (TNF)-α (Dearman et al. Citation2003a,Citationb). These methods can be used to detect respiratory allergens after short exposure periods; however, because these methods do not target the respiratory tract as the specific organ affected by respiratory allergy, false-positive and false-negative results are common (Dearman et al. Citation2003a,Citationb; Plitnick et al. Citation2005).
Therefore, it is necessary to develop a method that specifically detects allergic reactions within the respiratory tract following long-term dermal sensitization and intratracheal or inhaled respiratory challenge. Furthermore, additional optimization and modifications of such a method may be required to allow detection of allergens other than liquid chemical allergens (Fukuyama et al. Citation2009a; Nishino et al. Citation2014). Accordingly, this study was designed to elucidate mechanisms of small particulate chemical-induced respiratory allergic disorders, using a mouse model of chemical-induced respiratory allergy and the well-known chemical allergen TMA. Additionally, this study sought to provide insights into novel detection methods and in vivo models for environ-mental small particulate chemical-induced allergic respiratory disorders.
Materials and methods
Mice
This study used BALB/c mice – a popular strain used in allergy models that is known as a high responder of TH2-mediated immunoreactions including IgE levels (Lin et al. Citation2006) – and NC/Nga mice (Kondo et al. Citation1969) that have been used in models of human atopic dermatitis and allergic airway inflammation (Iwasaki et al. Citation2001aCitationb). Female inbred BALB/c and NC/Nga mice (age 7 week) were purchased from Charles River Japan (Atsugi, Kanagawa, Japan) and acclimated for 1 week before the start of the experiment. All mice were housed individually under controlled lighting (on 7:00–19:00 h), temperature (22 ± 3 °C), humidity (50 ± 20%), ventilation (at least 10 complete fresh-air changes hourly), and SPF conditions. Food (Certified Pellet Diet MF, Oriental Yeast, Tokyo, Japan) and water were available ad libitum. The current study was conducted in accordance with the Animal Care and Use Program of the Institute of Environ-mental Toxicology (IET IACUC Approval nos. AC13098 and 13225).
Reagents
Trimellitic anhydride (TMA, C9H4O5, >97%), acetone, and olive oil were purchased from Wako Pure Chemical Industries (Osaka, Japan). For dermal sensitization, TMA was dissolved in acetone/olive oil (4:1) to 1.0% (w/v). For inspiratory challenge, TMA was pulverized into particles measuring 2-μm in diameter (single track jet mill, FS-4; Seisin Enterprise Co., Tokyo, Japan). Phosphate-buffered saline (PBS), RPMI 1640 medium, heat-inactivated fetal calf serum (FCS), and Dynabeads Mouse T-Activator CD3/CD28 were ordered from Life Technologies (Tokyo, Japan). The BD OptEIA Mouse IgE ELISA Set for enzyme-linked immunosorbent assays (ELISA) was purchased from BD Pharmingen (Tokyo, Japan). The Imject Immunogen EDC Kit was bought from Pierce Biotechnology (Rockford, IL). Streptavidin-horseradish peroxidase was purchased from BD Pharmingen (Tokyo, Japan). NucleoSpin RNA II, a PrimeScript RT Reagent Kit with gDNA Eraser, and primers were purchased from TaKaRa Bio (Tokyo, Japan).
Mouse BD Fc Block, biotin-conjugated anti-mouse IgE (rat IgG1, clone R35-118), fluorescein isothiocyanate (FITC)-conjugated anti-mouse IgE (rat IgG1, clone R35-72), phyco-erythrin (PE)-conjugated anti-mouse CD62L (rat IgG2a, clone MEL-14), allophycocyanin (APC)-conjugated anti-mouse CD3 (hamster IgG1, clone 145-2C11), PE-Cy7-conjugated anti-mouse CD4 (rat IgG2a, clone RM4-5), APC-Cy7-conjugated anti-mouse CD8 (rat IgG2a, clone 53-6.7), peridinin chlorophyll protein (PerCP)-conjugated anti-mouse CD45R/B220 (rat IgG2a, clone RA3-6B2), APC-Cy7-conjugated anti-mouse CD11c (hamster IgG1, clone HL3), PerCP-Cy5.5-conjugated anti-mouse Gr-1 (rat IgG2b, clone RB6-8C5), FITC-conjugated anti-mouse CD23 (FcɛRII; rat IgG2a, clone B3B4), PE-Cy7-conjugated anti-mouse CD117 (c-Kit; rat IgG2b, clone 2B8), APC-conjugated anti-mouse CD49b (hamster IgG1, clone HMα2), PE-conjugated anti-mouse CD49d (rat IgG2a, clone 9C10), and the BD CBA Mouse Flex Set (cytometric bead array: IL-4, IL-5, IL-6, KC, monocyte chemotactic protein [MCP]-1, and TNFα) were all purchased from BD Pharmingen (Tokyo, Japan). Quantikine ELISA Mouse CCL11/Eotaxin kits were purchased from R&D Systems (Minneapolis, MN) while Histamine EIA kits were from Bertin Pharma (Paris, France).
TMA-induced respiratory allergy model in mice
The TMA-induced respiratory allergy model was used as described previously with minor modifications (Zhang et al. Citation2006; Nishino et al. Citation2014). BALB/c and NC/Nga mice (age, 8 weeks) to groups (n = 8 mice/group) were randomly allocated for dosing or for no treatment (control). In a previous study, it was already demonstrated that the immune responses in either only TMA-sensitized or only TMA-challenged hosts were comparable to those in untreated ones (Fukuyama et al. Citation2008, Citation2009a,Citationb). Therefore, an untreated group was used in this study to indicate the baseline for each endpoint. For sensitization, 1% TMA was applied topically to both ears (25 μl/ear) of each mouse on Days 1–3, 8–10, and 15–17. Two weeks after the final sensitization, each mouse was subjected to inhalation challenge with 0.5 mg TMA/L for three consecutive days using our inhalation exposure system (see below).
One day after the final challenge, all mice were euthanized by exsanguination from the abdominal aorta and posterior vena cava following intraperitoneal injection of pentobarbital sodium (75 mg/kg). Blood samples were taken from the inferior vena cava, and serum samples were assayed for total or TMA-specific IgE levels.
Bronchoalveolar lavage fluid (BALF) was collected by cannulating the trachea and lavaging both lungs three times each with 1 ml PBS supplemented with 1% FCS. The first BALF fraction from each animal was centrifuged at 350 × g for 5 min. The supernatant of the first BALF fraction was collected for later measures of chemokine levels. The cell pellets of all three fractions/mouse were used for differential cell ratio determinations (note: total lavage volumes isolated from untreated mice and treated mice were comparable). The lavaged lung from each mouse was removed and immediately evaluated for any red coloration (semi-quantitatively evaluation of clinical symptoms). Each set of lungs was then divided for use in histology (immediately fixed; see protocol below) and gene expression assays (immediately flash-frozen in liquid N2 and stored at −80 °C until RNA was isolated; see protocol below).
Hilar lymph nodes (LN) from each mouse were isolated and pooled in RPMI 1640 medium (Life Technologies, Tokyo, Japan). Single-cell suspensions were prepared by passage of the LN through a sterile 70-μm nylon cell strainer in 1 m RPMI 1640 supplemented with 5% FCS. Single-cell suspensions were subsequently used to analyze levels of memory helper T-cells and IgE+ B-cells, as well as cytokine production.
Inhalation exposure
TMA inhalation exposure was performed as described earlier with an original nose-only system (total volume, 31.2 L; Tokiwa Kagakukikai Co., Ltd., Tokyo, Japan) and a turntable-type dust feeder (DF-3, turntable TA31; Sibata Scientific Technology Ltd., Tokyo, Japan) (Nishino et al. Citation2014). Mice were exposed to 0.5 mg TMA/L dust continuously for 30 min daily. The mass median aerodynamic diameter and geometric standard deviation were monitored using a dust sampler (MCI-500 sampler; Tokyo Dylec Corp., Tokyo, Japan) and kept at ≈4.0 μm and 2.0, respectively, throughout the inhalation exposures. Four hours after respiratory challenge, each mouse was carefully observed for appearance, posture, behavior, respiration, consciousness, and clinical symptoms (abnormal respiratory sound) of each mouse were evaluated semi-quantitatively (0 = no sign, 1–4 = increasingly severe signs) in a blinded fashion.
Serum IgE measures
Total IgE levels in serum were measured using commercial ELISA kits according to protocols of the manufacturer. TMA-specific IgE levels in serum were measured using an Imject Immunogen EDC Kit and ELISA, as described previously (Fukuyama et al. Citation2009b).
Lung histology
Lung harvested as above were fixed in 10% formalin, and embedded in paraffin. Sections (5-μm thick) were then prepared and affixed to slides, stained with hematoxylin–eosin and toluidine blue, and imaged using an AZ100 Multizoom microscope (Nikon, Tokyo, Japan) with a 40× objective. Hematoxylin and eosin-stained sections were evaluated in a blinded manner – using a semi-quantitative scoring approach (0 = no influx, no edema; 1–3 = high influx, prominent edema) – to measure cell influx and edema. Toluidine blue-stained sections were also analyzed in a blinded manner – using a semi-quantitative scoring approach (0 = no influx; 1–3 = high influx) – to measure influx of granulated mast cells.
Gene expression in lung
Total RNA was extracted from accessory lobes using a NucleoSpin RNA II kit according to protocols of the manufacturer. Isolated RNA was then reverse transcribed with a PrimeScript RT Reagent Kit (TaKaRa Bio, Tokyo, Japan) with gDNA Eraser according to manufacturer protocols. Resulting cDNA were amplified using a quantitative real-time reverse transcription polymerase chain reaction (qRT-PCR) and a Thermal Cycler Dice system (TaKaRa Bio, Tokyo, Japan). Data acquired for each sample were normalized to expression levels of Actb (β-actin; housekeeping gene). The PCR primers for CCL11, IL4, IL5, TNF, and Actb are listed in .
Table 1. Primers used in the study.
Flow cytometric analyses of BALF and LN cells
To avoid non-specific binding, isolated BALF or LN cells (106 cells) were first incubated with 1 μg Mouse BD Fc Block solution for 5 min at 4 °C, and then incubated with monoclonal antibodies (at manufacturer recommended levels) for 30 min at 4 °C in the dark. Thereafter, the cells were washed twice with 5% FCS in PBS, re-suspended at 106 cells/tube in PBS, and immediately analyzed on a FACSVerse flow cytometer (BD Pharmingen, Tokyo, Japan) using an associated FACSuite program. To analyze antigen expression, 5000 and 20 000 events were acquired for, respectively, the BALF and LN samples. BALF cells were differentiated as shown in . In brief, highly, mildly, or non-granulated cells were first separated based on Gr-1 expression. Fc receptor-1 and C-kit double-positive highly granulated cells were then differentiated as granulated mast cells. On the other hand, highly granulated cells with only C-kit expression were isolated/defined as neutrophils. Mildly granulated cells were then differentiated into eosinophils, macrophages, and basophils based on expression of Fc-receptor 1, CD49b, and/or CD49d, respectively.
Cytokine, chemokine, and histamine levels in BALF
Cytokine, chemokine, and histamine levels in BALF were measured using an equal volume of supernatant from the first BALF. Levels of cytokines and chemokines (IL-4, IL-5, IL-6, KC, MCP-1, and TNFα) were measured using a cytometric bead array according to protocols of the manufacturer. Levels of eotaxin and histamine were each measured by ELISA, according to protocols of the manufacturer.
LN cytokine measures
To stimulate T-cell receptor signaling, single-cell suspensions recovered from LN (at 106 cells/well of 48-well plates) were cultured with Dynabeads Mouse T-Activator CD3/CD28 (25 μg/well) antibodies (Life Technologies, Tokyo, Japan) for 24 h at 37 °C in a 5% CO2 atmosphere. Levels of IL-4, IL-5, and IL-6 in the well supernatants (cell culture medium) were then measured using a cytometric bead array.
Statistical analyses
Statistical significance of differences between each group was estimated at 5% and 1% probability levels. Data were transformed logarithmically to show variances. Analysis of variance (ANOVA) was then used to evaluate the results. When ANOVA results were significant, the differences between groups were assessed by means of ANOVA followed by a Tukey–Kramer test. All data are expressed as means ± SD. All data were analyzed using Prism 4 software (GraphPad, San Diego, CA).
Results
Clinical signs associated with TMA treatments
All mice were carefully observed for their appearance, posture, behavior, and respiration just after TMA challenge. There were no abnormal clinical signs in TMA-treated BALB/c mice. However, abnormal respiratory sounds and labored respiration were noted in TMA-treated NC/Nga mice ().
Table 2. Clinical observations.
Effects of inspiratory TMA induction on lung appearance and lung tissue
To examine whether TMA challenge affected lung inflammation in the two mouse strains, lung appearance and histological features of airway inflammation in lung tissues were monitored. Although only weak or moderate features of lung abnormalities were noted in the TMA-treated BALB/c mice, moderate or severe features of abnormalities were seen in the TMA-treated NC/Nga mice (). In addition, hyperplasia of the alveolar wall, alveolar edema, and infiltration of inflammatory cells into the lung were significantly induced by TMA challenge in the NC/Nga mice ().
Figure 1. Effects of inspiratory TMA induction on lung appearance and lung tissue. (A) Score of red color in the lungs. Grade was determined by semi-quantitative examination (0 = no change, 1 = weak, 2 = moderate, 3 = severe changes). Data shown are means ± SD (n = 8/group). (B) Histological scores of hematoxylin–eosin-stained lung sections. Data were evaluated in blinded manner for measures of cell influx and edema using a semi-quantitative scoring approach (0 = no influx, no edema; 1–3 cell layers = high influx, prominent edema). Data shown are means ± SD. (C) Representative histological features with hematoxylin–eosin-stained sections in each group. The histological features of the BALB/c (control and TMA-treated) and NC/Nga (control group) mice shown had a score = 0, while those histological features of the NC/Nga (TMA-treated) mouse shown had a score = 3. Values significantly different from control group, or between TMA-treated NC/Nga mice and TMA-treated BALB/c mice (**p < 0.01).
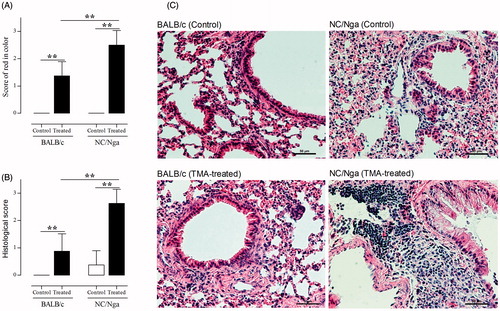
Comparison of mast cell functions between BALB/c and NC/Nga mice in TMA-treated respiratory allergy model
To assess allergic airway inflammation in the respiratory system, the percentages of lymphocytes, eosinophils, neutrophils, granulated mast cells, and basophils in the BALF were first measured (). Lymphocyte and eosinophil percentages were significantly increased (p < 0.01) in TMA-treated groups compared with those in control groups; there were no significant differences in the percentages of these immunocytes between TMA-treated NC/Nga and -treated BALB/c mice. On the other hand, the percentages of neutrophils, granulated mast cells, and basophils in NC/Nga mice were significantly enhanced by TMA as compared with those in control mice and in TMA-treated BALB/c mice. No differences in percentages of neutrophils, granulated mast cells, and basophils were noted in TMA-treated versus control BALB/c mice. To confirm the granulated mast cells numbers in lung tissues, toluidine blue-stained lung sections were also analyzed (). Significant increases were noted in the number of mast cells in the BALF and the infiltration of granulated mast cells in the lung tissues following TMA treatment in NC/Nga mice compared with in the BALB/c mice.
Figure 2. Percentages of neutrophils, mast cells, and basophils in the BALF of mice in various treatment groups. (A) Lymphocytes, (B) eosinophils, (C) neutrophils, (D) mast cells, and (E) basophil percentages among all BALF cells. Data shown are means ± SD (n = 8/group). Values were significantly different from the control group, or between TMA-treated NC/Nga mice and TMA-treated BALB/c mice are indicated (**p < 0.01). (F) Representative strategy for differentiating BALF cells by flow cytometry.
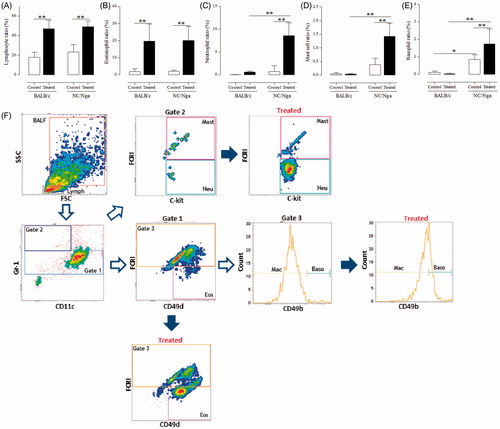
Figure 3. Influx of granulated mast cells in lung induced by TMA in NC/Nga and BALB/c mice. (A) Histological analysis of toluidine-blue sections in lung. Granulated mast cells were analyzed in a blinded manner to measure influx of granulated mast cells using a semi-quantitative scoring approach (0 = no influx; 1–3 = high influx). Data shown are means ± SD (n = 8/group). Values were significantly different from the control group, or between TMA-treated NC/Nga mice and TMA-treated BALB/c mice are indicated (**p < 0.01). (B) Representative histological features with toluidine blue-stained sections in each group.
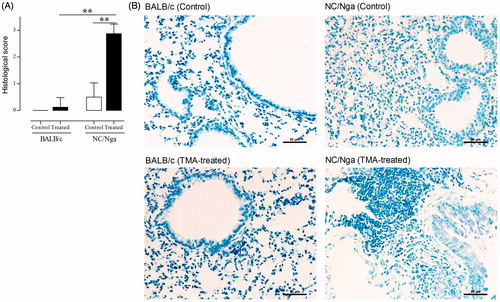
When the secretion of mast cell-related cytokines and histamine into the BALF was measured, it was found that the levels of IL-5, TNFα, MCP-1, eotaxin-1/CCL11, and histamine were significantly increased in TMA-treated NC/Nga mice compared with levels noted in the BALF of control and/or TMA-treated BALB/c mice (). In addition, analysis of lung tissue expression of CCL11, IL5, and TNF genes revealed that their expression levels were significantly higher in TMA-treated NC/Nga mice than in control and TMA-treated BALB/c mice ().
Figure 4. Histamine- and mast cell-related cytokine release induced by TMA in NC/Nga and BALB/c mice. Levels of (A) histamine (nM), (B) eotaxin-1 (nM), (C) IL-5 (pg/ml), (D) TNFα (pg/ml), and (E) MCP-1 (pg/ml) in BALF. Data shown are means ± SD (n = 8/group). Values significantly were different from the control group, or between TMA-treated NC/Nga mice and TMA-treated BALB/c mice are indicated (**p < 0.01).
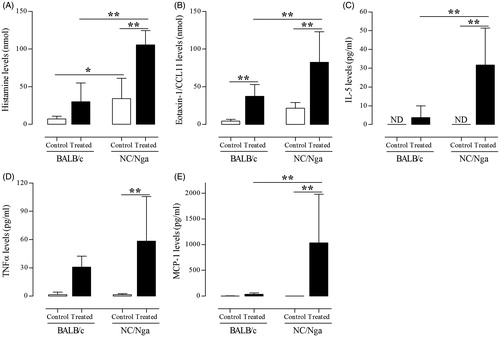
Figure 5. TMA-induced effects on mast cell-related expression of genes encoding cytokines in NC/Nga and BALB/c mice. (A) CCL11, (B) IL-5, and (C) TNF gene expression in lung tissues. Gene expression values are presented as increase in expression compared with value for Actb, and are reported as means ± SD (n = 8/group). Values were significantly different the control group, or between TMA-treated NC/Nga mice and TMA-treated BALB/c mice are indicated (**p < 0.01).
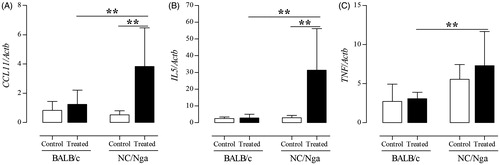
Prominent enhancement of the TH2 response was observed in TMA-induced BALB/c mice
To examine whether TMA challenge affected the TH2 response in the two mouse strains, total and TMA-specific IgE levels in serum were measured (). Contrary to the mast cell-related responses, total serum IgE levels were significantly increased in TMA-treated BALB/c mice compared with those in the TMA-treated NC/Nga group (p < 0.01).
Figure 6. Effect of TMA on TH2 responses in BALB/c and NC/Nga mice. (A) Total serum IgE levels (pg/ml) and (B) TMA-specific IgE titres in serum. (C) IL-4, (D) IL-6, and (E) KC levels (all pg/ml) in BALF. (F) IL4 gene expression in lung tissues. Data shown are means ± SD (n = 8/group). Gene expression values are presented as increase in expression compared with the value for Actb. Values were significantly different from the control group, or between TMA-treated NC/Nga mice and TMA-treated BALB/c mice are indicated (**p < 0.01).
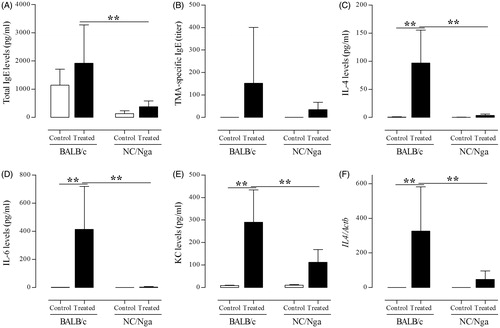
Next, secretion of TH2-related cytokines/chemokines in the BALF was examined. It was found that levels of IL-4, IL-6, and KC were significantly increased (p < 0.01) in TMA-treated NC/Nga mice compared with levels in control and TMA-treated BALB/c mice (). Consistent with the results of IgE and TH2-related cytokine secretion, IL4 gene expression in lung tissues was significantly up-regulated by TMA in BALB/c mice compared with that in control and TMA-treated NC/Nga mice ().
Effects of inspiratory TMA induction on hilar LN activation
To assess effects of inspiratory TMA induction on hilar LN activation, the numbers of memory helper T-cells and IgE+ B-cells, as well as production of pro-inflammatory cytokines like IL-4, IL-5, and IL-6, was measured (). Compared with that in the respective control mice, memory helper T-cell counts in the hilar LN were significantly increased in TMA-treated groups (p < 0.01). The number of IgE+ B-cells was increased slightly in TMA-treated NC/Nga mice compared with in control mice. In contrast, the number of IgE+ B-cells was markedly increased in TMA-treated BALB/c mice compared with in control and TMA-treated NC/Nga mice (p < 0.01). Expression levels of IL-4 and IL-6 [which activate B-cells] were significantly increased (p < 0.01) in hilar LN from BALB/c and TMA-treated NC/Nga mice. Additionally, the level of IL-5 was significantly increased in TMA-treated NC/Nga mice (p < 0.01) compared with that in the control (p < 0.01) and TMA-treated BALB/c mice (p < 0.05).
Figure 7. Impact of inspiratory TMA induction on hilar lymph node activation. Cell counts for (A) memory helper T-cells and (B) IgE+ B-cells in LN. (C) Representative histograms of IgE+ B-cells from LN of mice. (D) IL-4, (E) IL-5, and (F) IL-6 production (pg/ml) in LN from mice. Data shown are means ± SD (n = 8/group). Values significantly different from that of control group, or between TMA-treated NC/Nga mice and TMA-treated BALB/c mice are indicated (*p < 0.05, **p < 0.01).
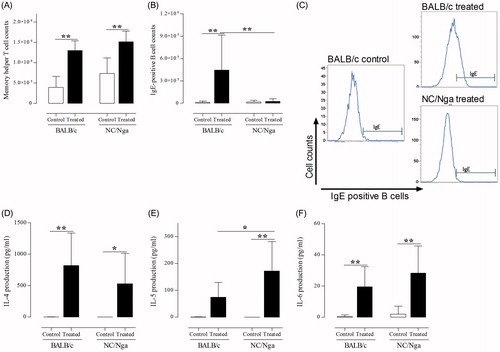
Discussion
In the present study, it was seen that environmental chemical allergen (formulated as a dry powder) induced respiratory allergic reactions that could be detected by our original protocol in both BALB/c and NC/Nga mice, and they showed the intriguing difference of allergic responses. Concretely speaking, there were almost no abnormalities on clinical observations, necropsies, and pathological findings in the lungs in TMA-induced BALB/c mice; however, exposure of NC/Nga mice to TMA resulted in significant increases in abnormal clinical signs, redness of the lung, and inflammatory features of lung tissues.
Within the past decade, new testing methods for respiratory allergy induced by environmental chemicals have been proposed in several guidelines, including the Globally Harmonized System (GHS) and the Regulation concerning the Registration, Evaluation, Authorization and Restriction of Chemicals (REACH). However, currently established testing methods have many limitations, and more appropriate methods are still required (United Nations Citation2011; European Union Citation2013). This study showed that the major respiratory allergic factors, such as IgE levels, eosinophil rates, and pro-inflammatory cytokine production, were significantly increased in TMA-treated mice compared with those in untreated control mice. These responses have been commonly observed in both patient with respiratory allergy and in animal models of respiratory allergy (Karol Citation1994; Maestrelli et al. Citation2009). Thus, this protocol could be an effective alternative model for identification of allergic respiratory disorders induced by environmental small particulate chemicals based on inflammatory responses in the lung.
Indeed, few reports have described methods combining a sensitization phase and inhalation challenge because of the requirements for intricate equipment, long experimentation times, and high costs (Arts & Kuper Citation2007). Thus, our protocol represents a substantial advancement as a novel method composed of both the sensitization and inhalation challenge phases, as well as examination of lung inflammation. Moreover, this protocol is expected to facilitate assessment of risk for respiratory sensitization.
The present study also sought to elucidate mechanisms of small particulate chemically-induced respiratory allergic disorders by comparing effects in BALB/c and NC/Nga mice that have different allergic response profiles, using our original protocol and pulverized TMA. Interestingly, abnormal clinical signs and inflammatory reactions in the lung were only noted in TMA-treated NC/Nga mice. NC/Nga mice are a popular animal model for allergic dermatitis and exhibit intense scratching behavior and severe inflammation of the skin compared with responses in BALB/c, ICR, or C3H/HeN mice (Hashimoto et al. Citation2006). In addition, Iwasaki et al. (Citation2001a, Citationb) reported that NC/Nga mice sensitized with ovalbumin (OVA) by intranasal challenge exhibited increased eosinophil counts in the lungs compared with in those of BALB/c mice subjected to the treatment. Therefore, the NC/Nga model was most appropriate for the present protocol.
The study here next investigated the key triggers that provoked aggravation of clinical signs and lung inflammation in TMA-treated NC/Nga mice. Interestingly, Iwasaki et al. (Citation2001a, Citationb) previously showed that levels of eosinophils were up-regulated in the lungs of OVA-induced NC/Nga mice. Interestingly, there were no significant changes in eosinophil populations or in levels of eotaxin that is secreted from eosinophils, between NC/Nga and BALB/c mice in the current study. On the contrary, the percentages of neutrophils, mast cells, and basophils were significantly enhanced by TMA in NC/Nga mice compared with in TMA-treated BALB/c mice. Each of these three cell types are known to participate in the allergic inflammatory reaction, and marked increases in these cells have been found in airways of patients with severe asthma (Monteseirin Citation2009; Stone et al. Citation2010). Individually, the increases in neutrophil counts are correlated with the progression of persistent airflow limitation in asthma (Shaw et al. Citation2007). Mast cells are central to the pathogenesis of allergic diseases, function to release lipid mediators, cytokines/chemokines, histamine, and TNFα. Activation of mast cell leads to activation of FcɛRI by polyvalent allergens, resulting in rapid synthesis and release of lipid mediators, sneezing, coughing, bronchospasm, edema, and mucous secretion in the respiratory tract (Stone et al. Citation2010). Basophils are also known to release histamine after activation and have been identified in cutaneous and pulmonary late-phase allergic responses (Sullivan & Locksley Citation2009; Stone et al. Citation2010). Based on data here, these mediators are likely to be key players aggravating the ambient chemical allergen-induced respiratory allergic reaction.
In addition, in this study, we were able to differentiate mast cells in the BALF though they should exist in the submucosal tissues. We had first hypothesized these cells were basophils. Basophils also play a similar role as mast cells in regard to IgE binding via FcɛRI and releasing histamine; it thus made sense to us that up-regulated IgE and histamine secretion in BALF were potentially associated with basophils. However, when the BALF cells were correctly differentiated using FACS and adequate markers based on previously described methods (Nabe et al. Citation2013), there was clearly a mast cell population present characterized by highly expressed Gr-1, c-kit and FcɛRI. However, there was also a different population that could be characterized as basophils based on expression of CD49b and Gr-1, but not c-kit. These results indicated that some mast cells could be flushed into the BAL. This could mean that this surprising outcome (i.e. mast cells in BALF) could eventually be useful as a marker to predict the allergic symptoms.
This study next examined the expression of cytokines/chemokines and the production of histamine in the BALF and/or lung tissues. The data showed that mast cell-related cytokines (IL-5, TNFα, and MCP-1) and histamine secretion into the BALF were significantly increased in TMA-treated NC/Nga mice compared with in TMA-treated BALB/c mice. Moreover, IL5 and TNF gene expression levels were increased in lung tissues from NC/Nga mice compared with in tissues from the BALB/c mice. IL-5, a prominent cytokine produced by mast cells, has been shown to play a critical role in eosinophil development and B-cell activation (Amin, Citation2012). TNFα, another major cytokine released by mast cells, can up-regulate adhesion molecules on endothelial and epithelial cells (Stone et al. Citation2010). MCP-1 is released from epithelial tissue [including airway smooth muscle layer] and directly induces pulmonary mast cell degranulation (Campbell et al. Citation1999). Histamine, which can facilitate the characteristic symptoms of allergy (e.g. mucous secretion), is produced by mast cells and basophils and has effects on the smooth muscle, endothelial cells, and nerve endings (Stone et al. Citation2010).
Marked degranulation of mast cells and basophils has been observed in the epidermis of NC/Nga mice in an atopic dermatitis model; this indicated that mast cells and basophils played a key role in aggravation of atopic dermatitis-like skin lesions in NC/Nga mice (Yamamoto et al. Citation2007). However, no studies have compared the numbers of mast cells or basophils between NC/Nga mice and any other strains of mice. In the current study, it was demonstrated that the numbers of mast cells and basophils in the BALF of NC/Nga mice were significantly greater than those in the BALF of BALB/c mice. These phenomena were confirmed using toluidine blue staining of lung tissues. To the best of our knowledge, this is the first report to demonstrate the increased numbers of mast cells and basophils in the NC/Nga mouse model as compared with in other strains of mice, and the data here strongly suggested that these cells were responsible for induction of respiratory allergy in this model.
Because TMA is recognized as a TH2-type allergen and several reports have shown that topical TMA exposure leads to increased TH2 cytokine secretion in mice, this study next examined TH2-type allergic reactions in the NC/Nga model. Contrary to the results concerning neutrophil, mast cell, and basophil activation, total or specific IgE levels in serum were markedly increased in TMA-treated BALB/c mice compared with those in NC/Nga mice. Consistent with IgE levels, the release of pro-inflammatory TH2 cytokines and chemokines (i.e. IL-4, IL-6, and KC) into the BALF was also significantly increased by TMA. These analyses also indicated a greater expression of the IL4 gene in lung tissues of TMA-treated BALB/c mice than in the tissues from the NC/Nga mice.
Lastly, to clarify the responses in BALF and lung tissues, this study examined local lymph nodes (LN). Consistent with the results from the BALF and lung tissues, mast cell-derived IL-5 production was increased in the LN of NC/Nga mice compared with that in LN of BALB/c mice. Moreover, IgE+ B-cell counts and IL-4 production were induced in LN following TMA exposure in BALB/c mice compared with in the NC/Nga mice LN. These data suggested to us that the outcomes from the BALF and lung tissues were representative of results in the host LN.
Generally speaking, BALB/c mice are one of the most useful mouse strains available for studies of the TH2-type allergic diseases because they are sensitive to TH2-type allergens and are able to provoke the production of IgE and related cytokines (Lin et al. Citation2006; Nishino et al. Citation2014). Consistent with this, the study here found there was a marked up-regulation of TH2 responses in TMA-induced BALB/c mice; however, there were fewer clinical signs or inflammatory features in the lung tissues than expected. On the other hand, obvious clinical symptoms of respiratory allergy in TMA-induced NC/Nga mice were also observed. Nonetheless, IgE and TH2 cytokine production were significantly lower in these mice than in BALB/c mice. As such, TH2-type responses, which commonly align with respiratory allergic endpoints, were insufficient here to predict clinical disease. Therefore, rather than TH2-based events, it would seem that neutrophils, mast cells, and basophils could drive the symptoms and inflammation associated with ambient chemical allergen-induced respiratory allergy.
NC/Nga mice are considered the first mouse model of chronic atopic dermatitis, as originally reported by Matsuda et al. (Citation1997). However, the development of a model mimicking skin lesions observed in human atopic diseases is still needed. To the best of our knowledge, the present study is the first to demonstrate that neutrophils, mast cells, and basophils induce dual clinical and pro-inflammatory effects in particulate matter-induced respiratory allergy by inhalation exposure in a mouse model. Thus, these findings provide a basis for further studies of the mechanisms of respiratory allergy caused by environmental chemical allergen and evaluation of the utility of NC/Nga mice for studies of atopic diseases.
Conclusions
Our original protocol could detect particulate matter-induced respiratory allergy. In addition, typical symptoms and inflammation of the respiratory tract, which are observed in human respiratory allergic diseases, were markedly induced in NC/Nga mice compared with in BALB/c mice. The data here also indicated that increased numbers of neutrophils, mast cells, and basophils in NC/Nga mice played a key role mediating these effects; these data were further supported by changes in the secretion of related cytokines and histamine. These factors may play a key role of predicting clinical symptoms of respiratory allergy; nevertheless, an important conclusion reached here was that many of the endpoints commonly used to assess allergic disease did not predict clinical symptoms. However, it is important to note that this mouse model only represented the acute phase of respiratory allergy. Further studies are necessary to examine chronic responses of respiratory allergy and causes of strain differences in order to fully elucidate the mechanisms underlying environmental particulate matter-induced respiratory allergy.
Acknowledgements
The authors wish to thank Dr. Y. Takizawa of the Institute of Environmental Toxicology (Uchimoriya-machi 4321, Joso-shi, Ibaraki 303-0043, Japan) for technical assistance. We would also like to thank Editage (www.editage.jp) for help in the English language editing.
Disclosure statement
The authors declare no conflicts of interest. The authors alone are responsible for the content of this manuscript.
References
- Agius RM, Nee J, McGovern B, Robertson A. 1991. Structure activity hypotheses in occupational asthma caused by low molecular weight substances. Ann Occup Hyg. 35:129–137.
- Amin K. 2012. The role of mast cells in allergic inflammation. Respir Med. 106:9–14.
- Arts JH, Kuper CF. 2007. Animal models to test respiratory allergy of low molecular weight chemicals: A guidance. Methods. 41:61–71.
- Brown MM, Jason LA. 2007. Functioning in individuals with chronic fatigue syndrome: increased impairment with co-occurring multiple chemical sensitivity and fibromyalgia. Dynam Med. 6:6.
- Campbell EM, Charo IF, Kunkel SL, Strieter RM, Boring L, Gosling J, Lukacs NW. 1999. MCP-1 mediates cockroach allergen-induced bronchial hyper-reactivity in normal but not CCR2−/− mice: Role of mast cells. J Immunol. 163:2160–2167.
- Dearman RJ, Basketter DA, Kimber I. 1992. Variable effects of chemical allergens on serum IgE concentration in mice. Preliminary evaluation of a novel approach to identification of respiratory sensitizers. J Appl Toxicol. 12:317–323.
- Dearman RJ, Betts CJ, Humphreys N, Flanagan BF, Gilmour NJ, Basketter DA, Kimber I. 2003a. Chemical allergy: considerations for the practical application of cytokine profiling. Toxicol Sci. 71:137–145.
- Dearman RJ, Skinner RA, Humphreys NE, Kimber I. 2003b. Methods for identification of chemical respiratory allergens in rodents: Comparisons of cytokine profiling with induced changes in serum IgE. J Appl Toxicol. 23:199–207.
- European Union. Evaluation and Authorization of Chemicals (REACH). [updated 1 July 2013]. Available from: http://eur-lex.europa.eu/legal-content/EN/TXT/PDF/?uri=CELEX:31967L0548&from=EN.
- Fukuyama T, Ueda H, Hayashi K, Tajima Y, Shuto Y, Saito T, Harada T, Kosaka T. 2008. Use of long-term dermal sensitization followed by intratracheal challenge method to identify low-dose chemical-induced respiratory allergic responses in mice. Toxicol Lett. 181:163–170.
- Fukuyama T, Tajima Y, Ueda H, Hayashi K, Shutoh Y, Harada T, Kosaka T. 2009a. Allergic reaction induced by dermal and/or respiratory exposure to low-dose phenoxyacetic acid, organophosphorus, and carbamate pesticides. Toxicology. 261:152–161.
- Fukuyama T, Tajima Y, Ueda H, Hayashi K, Shutoh Y, Saito T, Harada T, Kosaka T. 2009b. Investigation of the chemical-induced selective Type II (TH2) allergic response in mice: Effect of length of sensitizing phase. J Immunotoxicol. 6:75–83.
- Gavett SH, Haykal-Coates N, Copeland LB, Heinrich J, Gilmour MI. 2003. Metal composition of ambient PM2.5 influences severity of allergic airways disease in mice. Environ Health Perspect. 111:1471–1477.
- Hashimoto Y, Arai I, Takano N, Tanaka M, Nakaike S. 2006. Induction of scratching behavior and dermatitis in various strains of mice co-habiting with NC/Nga mice with chronic dermatitis. Br J Dermatol. 154:28–33.
- Howarth PH. 1998. Is allergy increasing? Early life influences. Clin Exp Allergy. 28:2–7.
- Iwasaki T, Tanaka A, Itakura A, Yamashita N, Ohta K, Matsuda H, Onuma M. 2001a. Atopic NC/Nga mice as model for allergic asthma: Severe allergic responses by single intranasal challenge with protein antigen. J Vet Med Sci. 63:413–419.
- Iwasaki T, Tanaka A, Matsuda H, Onuma M. 2001b. Atopic NC/Nga mice as a model for allergic asthma: Cytokine profiles and eosinophil productivity of bone marrow. J Vet Med Sci. 63:471–474.
- Janssen NA, Brunekreef B, van Vliet P, Aarts F, Meliefste K, Harssema H, Fischer P. 2003. Relationship between air pollution from heavy traffic and allergic sensitization, bronchial hyper-responsiveness, and respiratory symptoms in Dutch school children. Environ Health Perspect. 111:1512–1518.
- Jeebhay MF, Quirce S. 2007. Occupational asthma in the developing and industrialized world: a review. Int J Tuberc Lung Dis. 11:122–133.
- Karol MH. 1994. Animal models of occupational asthma. Eur Respir J. 7:555–568.
- Kimber I, Agius R, Basketter DA, Corsini E, Cullinan P, Dearman RJ, Gimenez-Arnau E, Green-well L, Hartung T, Kuper F, et al. 2007. Chemical respiratory allergy: Opportunities for hazard identification and characterization. Report and recommendations of ECVAM Workshop 60. Alt Lab Anim. 35:243–265.
- Kimber I, Dearman RJ. 2002. Chemical respiratory allergy: Role of IgE antibody and relevance of route of exposure. Toxicology. 181:311–315.
- Kondo K, Nagami T, Teramoto S. 1969. Differences in hematopoietic death among inbred strains of mice. In: Bond PV, Sugahara T, editors. Comparative cellular and species radiosensitivity. Tokyo: Igaku Shoin Ltd. pp. 20–29.
- Lin JY, Chen ML, Chiang BL, Lin BF. 2006. Ganoderma tsugae supplementation alleviates bronchoalveolar inflammation in airway sensitization and challenge mouse model. Int Immunopharmacol. 6:241–251.
- Maestrelli P, Boschetto P, Fabbri LM, Mapp CE. 2009. Mechanisms of occupational asthma. J Allergy Clin Immunol. 123:531–542.
- Matsuda H, Watanabe N, Geba GP, Sperl J, Tsudzuki M, Hiroi J, Matsumoto M, Ushio H, Saito S, Askenase PW, et al. 1997. Development of atopic dermatitis-like skin lesion with IgE hyper-production in NC/Nga mice. Int Immunol. 9:461–466.
- Monteseirin J. 2009. Neutrophils and asthma. J Invest Allergol Clin Immunol. 19:340–354.
- Nabe T, Matsuya K, Akamizu K, Fujita M, Nakagawa T, Shioe M, Kida H, Takiguchi A, Wakamori H, Fujii M, et al. 2013. Roles of basophils and mast cells infiltrating the lung by multiple antigen challenges in asthmatic responses of mice. Br J Pharmacol. 169:462–476.
- Nishino R, Fukuyama T, Watanabe Y, Kurosawa Y, Ueda H, Kosaka T. 2014. Effect of mouse strain in a model of chemical-induced respiratory allergy. Exp Anim. 63:435–445.
- Plitnick LM, Loveless SE, Ladics GS, Holsapple MP, Smialowicz RJ, Woolhiser MR, Anderson PK, Smith C, Selgrade MJ. 2005. Cytokine mRNA profiles for isocyanates with known and unknown potential to induce respiratory sensitization. Toxicology. 207:487–499.
- Pauluhn J, Woolhiser MR, Bloemen L. 2005. Repeated inhalation challenge with diphenyl-methane-4,4’-diisocyanate in Brown Norway rats leads to a time-related increase of neutrophils in bronchoalveolar lavage after topical induction. Inhal Toxicol. 17:67–78.
- Rattray NJ, Botham PA, Hext PM, Woodcock DR, Fielding I, Dearman RJ, Kimber I. 1994. Induction of respiratory hypersensitivity to diphenylmethane-4,4’-diisocyanate (MDI) in guinea pigs. Influence of route of exposure. Toxicology. 88:15–30.
- Rothen-Rutishauser BM, Kiama SG, Gehr P. 2005. A three-dimensional cellular model of human respiratory tract to study the interaction with particles. Am J Respir Cell Mol Biol. 32:281–289.
- Shaw DE, Berry MA, Hargadon B, McKenna S, Shelley MJ, Green RH, Brightling CE, Wardlaw AJ, Pavord ID. 2007. Association between neutrophilic airway inflammation and airflow limitation in adults with asthma. Chest. 132:1871–1875.
- Stone KD, Prussin C, Metcalfe DD. 2010. IgE, mast cells, basophils, and eosinophils. J Allergy Clin Immunol. 125:S73–80.
- Sullivan BM, Locksley RM. 2009. Basophils: Non-redundant contributor to host immunity. Immunity. 30:12–20.
- United Nations. 2011. Globally Harmonized System of Classification and Labeling of Chemicals (GHS): 4th Revised Edition. [updated 2011] New York and Geneva, United Nations, Available from: http://www.unece.org/fileadmin/DAM/trans/danger/publi/ghs/ghs_rev04/English/ST-SG-AC10-30-Rev4e.pdf.
- Yamamoto M, Haruna T, Yasui K, Takahashi H, Iduhara M, Takaki S, Deguchi M, Arimura A. 2007. A novel atopic dermatitis model induced by topical application with Dermatophagoides farinae extract in NC/Nga mice. Allergol Intl. 56:139–148.
- Zhang XD, Andrew ME, Hubbs AF, Siegel PD. 2006. Airway responses in Brown Norway rats following inhalation sensitization and challenge with trimellitic anhydride. Toxicol Sci. 94:322–329.