Abstract
Background and purpose Joint replacement with metal-on-metal (MOM) bearings have gained popularity in the last decades in young and active patients. However, the possible effects of MOM wear debris and its corrosion products are still the subject of debate. Alongside the potential disadvantages such as toxicity, the influences of metal particles and metal ions on infection risk are unclear.
Methods We reviewed the available literature on the influence of degradation products of MOM bearings in total hip arthroplasties on infection risk.
Results Wear products were found to influence the risk of infection by hampering the immune system, by inhibiting or accelerating bacterial growth, and by a possible antibiotic resistance and heavy metal co-selection mechanism.
Interpretation Whether or not the combined effects of MOM wear products make MOM bearings less or more prone to infection requires investigation in the near future.
Many young patients with painful coxarthrosis want to return to a high level of activity and require an implant that provides durability. The low wear rates of metal-on-metal (MOM) bearings have led to a resurgence in the use of MOM bearings (Wagner and Wagner Citation2000, Silva et al. Citation2005, Pollard et al. Citation2006, Vendittoli et al. Citation2007, Delaunay et al. Citation2008). 35% of all prostheses in the United States in 2006 (Bozic et al. Citation2009) and 16% of all prostheses implanted in Australia from 1999 through 2007 had MOM bearings (CitationGraves et al. 2008).
Metal alloys used in MOM bearings degrade through wear, from corrosion, or by a combination of the two (Yan et al. Citation2006, Jacobs et al. Citation2008). Consequently, MOM bearings produce nanometer- to submicrometer-sized metal particles (Campbell et al. Citation1996, Doorn et al. Citation1998). The high number of these very small particles presents a large cumulative surface area for corrosion. The biological effects of these particles and their corrosion products in the human body are for the most part unclear. Since the renewed interest in MOM bearings, extensive research has been done to determine the consequences of local and systemic exposure to wear particles and accompanying biologically active corrosion products (Amstutz and Grigoris Citation1996). It is well known that metal debris can induce pathological changes such as the release of inflammatory cytokines from macrophages, histiocytosis, fibrosis, and necrosis (Basle et al. Citation1996, Granchi et al. Citation1998, Caicedo et al. Citation2008, Citation2009). Metal debris is also thought to be associated with hypersensitivity and osteolysis (Hallab et al. Citation2000, Citation2010, Goodman Citation2007b, Carr and DeSteiger Citation2008, Huber et al. Citation2009). However, there is very little literature on the bacteriological effects of these degradation products (Anwar et al. Citation2007, Hosman et al. Citation2009). It is therefore unclear whether they can influence the risk of infection.
The Australian and New Zealand joint registries have shown that between 9% and 15% of all total hip arthroplasty (THA) revisions are carried out because of infections related to the primary prosthesis (CitationRothwell et al. 2007, CitationGraves et al. 2008). In cases of infection, bacteria adopt a biofilm mode of growth on the surface of the prosthesis, thus increasing the antibiotic resistance and resulting in major difficulties in treatment (Trampuz and Widmer Citation2006). Removal and replacement of an infected implant is usually required to eliminate the infection (Bozic and Ries Citation2005, Vincent et al. Citation2006). Recent research has suggested that particulate debris of any composition promotes bacterial growth by providing a scaffold for bacterial adhesion and biofilm growth (Anwar et al. Citation2007). On the other hand, high concentrations of metal ions have been shown to have bacteriostatic properties (Hosman et al. Citation2009).
Considering the paucity of publications on the effects of MOM particles on infection, we performed a review of the literature on the influence of MOM wear particles and their corrosion products on the risk of infection.
MOM bearings
History
First-generation MOM hip bearings include prostheses developed in the 1960s, such as the McKee-Farrar, the Ring, the Stanmore, and the Sivash prostheses (McKee and Watson-Farrar Citation1966, Ring Citation1968, Scales and Wilson Citation1969, Sivash Citation1969). Implants from this era survived for more than 25 years because of low wear rates and minimal osteolysis (Amstutz and Grigoris Citation1996). An analysis of 253 Ring MOM hip arthroplasties revealed a cumulative survival rate of 60% after 21 years (Bryant et al. Citation1991). The McKee-Farrar prosthesis performed equally well compared to the Ring arthroplasty, up to 26 years after initial implantation (Schmalzried et al. Citation1996). However, alongside these encouraging durability results, first-generation MOM studies also demonstrated metal wear debris in tissues adjacent to the implants, particularly in prostheses with loose components or impingement (Howie Citation1990). Furthermore, early MOM designs turned out to cause frequent early cup loosening (Schmalzried et al. Citation1996).
First-generation MOM articulations were commonly used until the mid-1970s. Most were abandoned in favor of metal-on-polyethylene (MOP) articulation. The main reason for this change was the introduction of the Charnley low-friction arthroplasty (Charnley Citation1972), which is still one of the most extensively documented hip prostheses in the literature (Callaghan et al. Citation2000, Citation2004, Wroblewski et al. Citation2007). Long-term results of first-generation MOM implants had boosted their popularity and led to the development of second-generation MOM implants in the early 1980s. In addition, polyethylene wear from MOP implants was then hypothesized to cause osteolysis around the implant (Wroblewski Citation1994, Oparaugo et al. Citation2001), which stimulated renewed interest in alternative bearings lacking a MOP interface, such as the second-generation MOM bearings (Brown et al. Citation2002).
Second-generation MOM implants have an improved bearing interface and are composed of alloys with an increased metal hardness. Newly produced bearings therefore have substantially lower rates of wear than highly cross-linked polyethylene (Fisher et al. Citation2006). On the whole, volumetric wear is reduced by 20- to 100-fold compared to MOP implants (Silva et al. Citation2005), suggesting that second-generation MOM prostheses may considerably reduce osteolysis (Sieber et al. Citation1999). Although medium- and long-term clinical results with MOM bearings appeared to have demonstrated excellent durability, recent studies have shown that MOM bearing systems are not refractory to osteolysis (Korovessis et al. Citation2006).
Alloys
For implant alloys worldwide, two nomenclatures are used in parallel to each other (). First of all, ASTM standards with a capital “F” (medical devices) are practiced mainly in the USA (Holzwarth et al. Citation2005). Secondly, ISO standards are accepted in the rest of the world. The 2 approved cobalt-chromium (Co-Cr) alloys contain almost similar amounts of alloying elements. However, there is no information available on the exact content of certain elements such as nickel (Ni) and iron (Fe), as these standards only report maximum amounts.
Table 1. Chemical composition and mechanical properties of CoCr28M06 alloy required by standards ASTM F75 and ISO 5832-4
Wear products
Wear
Wear in bearings can result in scratching and pounding of the surfaces, and eventually erosion of the material. Wear and corrosion are probably the major causes of release of metal into the tissues of MOM patients, and this poses a major concern regarding the use of MOM articulating devices. Linear wear rates range from 5 to 25 μm/year and are dependent on a multitude of factors such as the type of implant and positioning (Onda et al. Citation2008, Shimmin et al. Citation2008, Williams et al. Citation2008b) ().
Table 2. Wear rates of MOM bearing couples defined in different units
Metal particles
Currently, tribological research is being conducted on the exact process of particle generation. Recent tribological investigations have revealed that a nano-crystalline layer 250–400 nm thick is formed on the MOM implant surfaces, containing (amongst others) proteins from the interfacial medium (Pourzal et al. Citation2009). Cracking of this nano-crystalline layer due to surface fatigue has been suggested to be the main mechanism of generation of wear particles (Buscher et al. Citation2005). Abrasive particles of MOM prostheses can cause local damage, resulting in an accelerated release of metal particles and ions (Yan et al. Citation2009).
Germain et al. (Citation2003) emphasized that the nature, size, and amount of particles are important determinants of the biological effects of wear debris on cells in vitro. The reaction of the body is dependent on the characteristics of the particles (). Size analysis of particles isolated from failed arthroplasties has revealed a mean size of 660 nm for polyethylene particles in patients with MOP bearings (Minoda et al. Citation2008) and a size range from 51 to 116 nm for MOM debris (Doorn et al. Citation1996).
Table 3. Size and morphology of wear particles generated by a hip simulator or derived from tissue samples
Metal ions
The first report of visible corrosion of an orthopedic Vitallium implant (consisting of 60% Co and 20% Cr) was published by Weightman et al. in Citation1969. Before this clinical finding, it was generally accepted that Vitallium alloys provided adequate corrosion resistance (Scales et al. Citation1961). However, generation of metal ions is also evident in modern, more corrosion-resistant MOM alloys.
Various mechanisms of corrosion can cause the release of metal ions. One is fretting corrosion due to the movement of articulating surfaces causing damage to one or both surfaces. Disruption of the passive oxide layer causes direct contact with the metal surface, promoting fretting corrosion (), which can be enhanced further by the presence of adhering microorganisms (Muthukumar et al. Citation2003).
Schematic drawing illustrating: a. generation of wear particles; b. a metal alloy (gray scaffold) with an oxidized surface film on the upper surface (molecules marked in red); c. damage to the passive surface film (e.g. by scratching or pounding); d. occurrence of corrosion due to the lack of a protective layer; e. liberation of soluble compounds and wear particles; and f. repassivation of the surfaces including wear particles (arrows).
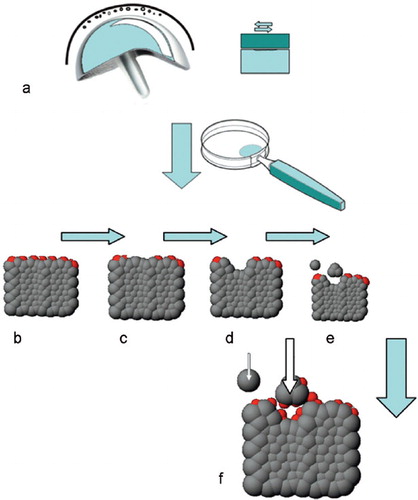
Metal ions of different valencies are released from Co-Cr alloys and their effects vary with the type of oxide compound they form (Virtanen Citation2006) (). Co2+ and Cr3+ ions predominate under physiological conditions because these ions are the most stable at neutral pH. However, no stable Co oxide exists and thus formation of soluble Co ions instead of solid Co oxides is favored. On the other hand, Cr3+ oxides are stable under physiological conditions. In vitro models have shown that the toxic effects of Co-Cr are probably due to Co2+ ions (Rae Citation1975, Garrett et al. Citation1983).
Table 4. Oxidation states of the elements in Co-Cr compounds
Local concentrations
Metal ions may spread throughout the body. Ion levels have been measured in whole blood, serum, erythrocytes, and various solid tissues (Cobb and Schmalzreid Citation2006, Savarino et al. Citation2008, Lazennec et al. Citation2009). Serum Co levels are the most frequently reported metal ion concentrations, and they were found to be 5- to 6-fold higher in patients after MOM implantation than preoperatively (Lazennec et al. Citation2009).
Two well-received consensus papers have described the need to measure metal ion concentrations in the joint fluids of patients with MOM bearings (Amstutz et al. Citation1996, MacDonald et al. Citation2004) in addition to measuring metal concentrations in serum (Vendittoli et al. Citation2007, Savarino et al. Citation2008). Most research into metal ion concentrations does not, however, report the regional or local dissemination, as synovial biopsies are undesirable in otherwise healthy patients (Savarino et al. Citation2008).
There is therefore very little reliable information about the exact local concentrations of Co-Cr around prostheses (). Concentrations of Co ions have been found in the 6–6,000,000 μg/L range. This difference is not only related to alignment and the type of the prosthesis (Onda et al. Citation2008, Shimmin et al. Citation2008, Williams et al. Citation2008b) but also to improving detection methods (Dorr et al. Citation1990).
Table 5. Maximum levels of Co and Cr ions in local tissues of patients with a MOM implant
Wear rates reported in recent hip simulator studies have turned out to show a close correlation with Co ion levels (Williams et al. Citation2008a), but measurement of ion levels in the lubricant of hip simulators resembles only certain aspects of the clinical situation. There is no free exchange between blood and synovial fluid (Dorr et al. Citation1990) and therefore wear products can accumulate in simulator systems, resulting in higher Co-Cr levels than would occur clinically ().
Table 6. Maximum levels of Co and Cr ions as measured with inductively coupled plasma mass spectroscopy in hip simulator lubricant, catogorized by head size of MOM implant and number of cycles
Influence of MOM wear particles and corrosion products on the immune system
Orthopedic metals and their corrosion products modulate the activities of the immune system by influencing immunocompetent organs and cells by a variety of immunostimulatory and immunosuppressive mechanisms. Metal particles and ions spread throughout the whole body via lymph and blood, and have, for instance, been identified within macrophages in the liver and spleen (Urban et al. Citation2000, Citation2004). The long-term effects of the distant spread and accumulation of wear particles in the liver and spleen are unknown, but indicate that the immune system may be hampered. In this context, it should be emphasized that the mere presence of a foreign body itself already reduces the minimum number of bacteria required to cause infection (Zimmerli et al. Citation1982).
Another important issue is the hypothetical carcinogenesis due to MOM implants and its accompanying occupation of the immune system in combination with the use of immunosuppressive drugs. Ion release has been suspected to increase the risk of DNA damage (Savarino et al. Citation2000, Ladon et al. Citation2004) and it was recently found that Co-Cr nanoparticles can cause DNA damage across a cellular barrier (Bhabra et al. Citation2009). In addition, a reduction in the number of circulating cytotoxic CD8+ T-cells, which are responsible for destroying tumor cells, has been found in patients with a MOM implant (Mabilleau et al. Citation2008, Ogunwale et al. Citation2009). However, epidemiological studies do not allow conclusions regarding the incidence of cancer in patients with MOM implants (Nyren et al. Citation1995, Visuri et al. Citation1996, Dumbleton and Manley Citation2005) and they will not become available in the near future, as such studies would require thousands of patients to be followed for several decades (MacDonald et al. Citation2004).
The spleen
The spleen is an important meeting point between antigenic information transported by the blood and the immunocompetent cells. Because of its central position in the bloodstream and its large blood supply of about 5% of the total blood volume per minute, the spleen will inevitably be exposed to corrosion products of MOM bearings. High concentrations of metal ions (375,000 μg/L Co and 200,000 μg/L Cr) have been shown to cause alterations in spleen architecture and depletion of T4 and B-cells. The immune system and its defense against bacteria may therefore become hampered by metal ions (Ferreira et al. Citation2003).
The liver
The liver is part of the human immune system and it not only contains many immunologically active cells but also detoxifies environmental toxins. Metals cannot be eliminated from tissues by metabolic degradation, but only by renal or gastrointestinal excretion (Cobb and Schmalzreid Citation2006). There is evidence from a recent animal study to suggest that Cr ions can accumulate in the liver (Jakobsen et al. Citation2007). High levels of metal in the body may cause hepatocellular necrosis, as observed after acute ingestion of Cr4+ in humans. Clinically relevant concentrations of Cr4+ (10–25 μM) have been found to inhibit macromolecular syntheses in the liver (Keegan et al. Citation2007).
Immunocompetent cells
Phagocytosing cells such as neutrophils are vital in the host defense against infection. These “first responders in microbial infection” are usually found in infected periprosthetic tissues. However, corrosion products of Co-Cr implant materials have been reported to inhibit the rapid release of reactive oxygen species required for bacterial killing by neutrophils (Shanbhag et al. Citation1992). In vitro studies have also shown that Co-Cr particles induce toxic effects after they are phagocytosed because of the drop in pH within the phagosome (Huk et al. Citation2004). Due to wear debris-induced granulocyte defects, patients with MOM implants may be predisposed to infection at the implant site (Zimmerli et al. Citation1982, Citation1984, Citation2004).
Degradation products, either in the form of metal ions or wear particles, can complex with local proteins and induce an allergic response comparable with a delayed-type hypersensitivity response (type IV), through activation of T-lymphocytes (Davies et al. Citation2005b, Goodman Citation2007a). The histological response in patients with MOM bearings is unique in its kind and is referred to as aseptic lymphocyte-dominated vasculitis-associated lesion (ALVAL) (Willert et al. Citation2005). In addition, a statistically significant reduction in circulating lymphocytes, in particular of CD8+ and T-cells, has been observed in patients with MOM bearings (Mabilleau et al. Citation2008, Ogunwale et al. Citation2009). However, at concentrations of Co and Cr below 5 μg/L, no such reduction was detected. No adverse clinical symptoms have been observed in patients with increased metal ion concentrations in serum (Hart et al. Citation2006).
Influence of MOM degradation products on bacteria
Heavy metal toxicity
Metal ions have been used for centuries to cure infections, and it is conceivable that wear products of MOM prostheses may be toxic to bacteria. There is in vitro and in vivo evidence that wear particles have toxic effects on human cells (Jones et al. Citation1975, Papageorgiou et al. Citation2007, Caicedo et al. Citation2008). In vitro research on influences of Co and Cr ions on bacteria have provided evidence of bacteriostatic effects (Anwar et al. Citation2007), hypothetically involving competition with Fe for uptake in the bacterial cell. Fe is an important nutrient element that is required by specific microbial species that use oxidation of elemental Fe or conversion of Fe2+ to Fe3+ as an energy source for their metabolism. Inhibition of Fe-dependent metabolic activities by Co ions has been shown to lead to growth retardation and cell death in Pseudomonas aeruginosa (Kothamasi and Kothamasi Citation2004).
Within the cells of tissues, nanoparticles are exposed to a series of oxidative mechanisms designed to destroy the foreign body, which leads to the generation of metal ions (Lundborg et al. Citation1992). Reactions with metal ions can lead to generation of free radicals: reactive oxygen species (ROS) and reactive nitrogen species (RNS), which can, in turn, cause cellular dysfunction. ROS and RNS are known to be involved in protein oxidation, leading to their degradation, lipid peroxidation, and DNA damage. Generation of ROS and RNS may, hypothetically, cause toxicity in bacteria as well.
Bacterial growth and biofilm formation
Some recent studies have evaluated the influence of Co and Cr ions and Co-Cr particles on bacterial growth. Co and Cr concentrations of up to 20 μg/L and 9 μg/L, respectively, that have been reported to occur in serum showed no consistent influence on biofilm formation, but higher concentrations of 200,000 μg/L Co and 93,000 Cr μg/L statistically significantly reduced Staphylococcus aureus and CNS planktonic growth and biofilm formation (Hosman et al. Citation2009), suggesting that MOM bearings may be less prone to biofilm formation and subsequent infection. On the other hand, Anwar et al. (Citation2007) showed that wear debris from MOM bearings accelerated the growth of planktonic bacteria. Aggregated particulate debris was suggested to promote growth by providing a scaffold on which biofilm can grow. In addition, it can be hypothesized that nanosized particles scattered throughout a biofilm would enhance the strength of its structure by working as a composite scaffold at the macroscopic level. Moreover, it is also possible that embedded particles in a biofilm might detach and act as carriers of biofilm throughout the joint and body.
Heavy metal resistance
Bacteria have co-existed with abundantly found toxic heavy metals since the beginning of life. Thus, it was essential for bacteria to develop mechanisms of metal resistance. Bacterial resistance to metal toxicity is not only an environmentally important phenomenon but also has clinical implications for metal-bacterium interactions in MOM patients. Bacterial resistance mechanisms differ widely (Silver and Misra Citation1988) and are currently the subject of extensive studies. There are enzyme oxidases and reductases to convert metal ions from more toxic species to less toxic species (Caccavo Jr et al. Citation1994, Cervantes et al. Citation2001, Lloyd and Lovley Citation2001, Kamaludeen et al. Citation2003). There is also the possibility of binding heavy metals in the bacterial cell wall (Komeda et al. Citation1997). Blocking of cellular uptake is also an option by altering the uptake pathway. Once the toxic heavy metal has reached the cytoplasm, it can be pumped out again by a high-efflux system (Nies et al. Citation1989, Nies Citation1995). Efflux pumps are the major group of resistance systems currently known.
Co-selection of antibiotic and metal resistance
There is growing concern that metal contamination may function as a selective agent in the proliferation of antibiotic resistance (Baker-Austin et al. Citation2006). It is hypothesized that antibiotic-resistant bacteria can be maintained in the environment owing to the co-regulation of resistance pathways (Baker-Austin et al. Citation2006, Wright et al. Citation2006). These co-selection mechanisms include co-resistance (with different determinants of resistance being present on the same genetic element) and cross-resistance (with the same genetic determinant being responsible for a conjoint resistance to antibiotics and metals). Co-resistance to multiple metals and antibiotics has been described in clinical isolates of Staphylococcus species (Ug and Ceylan Citation2003), but the most common co-resistance involves Cr, Pb, and penicillin. Co and Cr increase the sensitivity of staphylococci to penicillin, whereas sensitivity to tetracycline becomes less (Mnatsakanov Citation1967). The mechanisms behind this co-selection are currently being investigated.
It has been found that reduction of the permeability of bacteria causes Co and Ag resistance through a mechanism similar to that responsible for inhibiting β-lactam, ciprofloxacin, tetracycline, and chloramphenicol from entering the bacterium (Silver and Phung Citation1996, Ruiz et al. Citation2003). On top of this reduced permeability, a rapid efflux mechanism is also used to prevent Co, Cu, Zn, Cd, and Ni from entering the micro-organism, similar to the mechanism of resistance to ß-lactam, tetracycline, and chloramphenicol (Levy Citation2002, Nies Citation2003). The clinical incidence of co-selection mechanisms of resistance factors in pathogenic bacteria for antibiotics and heavy metals, and also their clinical implications, still remain unknown.
Conclusions
Unfortunately, long-term clinical data on infection rates for MOM bearings are not yet available and therefore actual clinical influences on infection cannot be evaluated. To assess the clinical influence of bearing type on infection risk, studies will require thousands of patients to be followed for several decades. Such data may soon become available from national joint registries, and their evaluation will shed light on the net influence of bearing type on infection risk. However, this review suggests that wear particles and their corrosion products may have an influence on the risk of infection by hampering the immune system, by inhibiting or accelerating bacterial growth, and by possible antibiotic resistance and metal resistance mechanisms involving co-selection. Whether this influence results in an increase in clinical infection rates or in a decrease has not yet been investigated.
AH and DN designed the study. AH conducted literature searches and drafted the manuscript. AH, SB, HvdM, HB, and DN contributed to the analysis and the manuscript.
No competing interests declared.
- Amstutz HC, Grigoris P. Metal on metal bearings in hip arthroplasty. Clin Orthop (Suppl) 1996; (329):S11-S34.
- Amstutz HC, Campbell P, McKellop H, Schmalzreid TP, Gillespie WJ, Howie D, Jacobs J, Medley J, Merritt K. Metal on metal total hip replacement workshop consensus document. Clin Orthop (Suppl) 1996; (329):S297-S303.
- Anwar HA, Aldam CH, Visuvanathan S, Hart AJ. The effect of metal ions in solution on bacterial growth compared with wear particles from hip replacements. J Bone Joint Surg (Br) 2007; 89:1655-9.
- Baker-Austin C, Wright MS, Stepanauskas R, McArthur JV. Co-selection of antibiotic and metal resistance. Trends Microbiol 2006; 14:176-82.
- Basle MF, Bertrand G, Guyetant S, Chappard D, Lesourd M. Migration of metal and polyethylene particles from articular prostheses may generate lymphadenopathy with histiocytosis. J Biomed Mater Res 1996; 30:157-63.
- Bhabra G, Sood A, Fisher B, Cartwright L, Saunders M, Evans WH, Surprenant A, Lopez-Castejon G, Mann S, Davis SA, Hails LA, Ingham E, Verkade P, Lane J, Heesom K, Newson R, Case CP. Nanoparticles can cause DNA damage across a cellular barrier. Nat Nanotechnol 2009; 4:876-83.
- Bozic KJ, Ries MD. The impact of infection after total hip arthroplasty on hospital and surgeon resource utilization. J Bone Joint Surg (Am) 2005; 87:1746-51.
- Bozic KJ, Kurtz S, Lau E, Ong K, Chiu V, Vail TP, Rubash HE, Berry DJ. The epidemiology of bearing surface usage in total hip arthroplasty in the United States. J Bone Joint Surg (Am) 2009; 91:1614-20.
- Brien WW, Salvati EA, Betts F, Bullough P, Wright T, Rimnac C, Buly R, Garvin K. Metal levels in cemented total hip arthroplasty. A comparison of well-fixed and loose implants. Clin Orthop 1992; (276):66-74.
- Brown SR, Davies WA, DeHeer DH, Swanson AB. Long-term survival of McKee-Farrar total hip prostheses. Clin Orthop 2002; (5):157-63.
- Brown C, Williams S, Tipper JL, Fisher J, Ingham E. Characterisation of wear particles produced by metal on metal and ceramic on metal hip prostheses under standard and microseparation simulation. J Mater Sci Mater Med 2007;18) 819-27.
- Bryant MJ, Mollan RA, Nixon JR. Survivorship analysis of the Ring hip arthroplasty. J Arthroplasty (6 Suppl) 1991: S5-10.
- Buscher R, Tager G, Dudzinski W, Gleising B, Wimmer MA, Fischer A. Subsurface microstructure of metal-on-metal hip joints and its relationship to wear particle generation. J Biomed Mater Res Part B-Applied Biomaterials 2005; 72B:206-14.
- Caccavo F, Jr., Lonergan DJ, Lovley DR, Davis M, Stolz JF, McInerney MJ. Geobacter sulfurreducens sp. nov., a hydrogen- and acetate-oxidizing dissimilatory metal-reducing microorganism. Appl Environ Microbiol 1994; 60:3752-9.
- Caicedo M, Jacobs JJ, Reddy A, Hallab NJ. Analysis of metal ion-induced DNA damage, apoptosis, and necrosis in human (Jurkat) T-cells demonstrates Ni2+ and V3+ are more toxic than other metals: Al3+, Be2+, Co2+, Cr3+, Cu2+, Fe3+, Mo5+, Nb5+, Zr2+. J Biomed Mater Res A 2008; 86:905-13.
- Caicedo MS, Desai R, McAllister K, Reddy A, Jacobs JJ, Hallab NJ. Soluble and particulate Co-Cr-Mo alloy implant metals activate the inflammasome danger signaling pathway in human macrophages: A novel mechanism for implant debris reactivity. J Orthop Res 2009; 27:847-54.
- Callaghan JJ, Albright JC, Goetz DD, Olejniczak JP, Johnston RC. Charnley total hip arthroplasty with cement. Minimum twenty-five-year follow-up. J Bone Joint Surg (Am) 2000; 82:487-97.
- Callaghan JJ, Templeton JE, Liu SS, Pedersen DR, Goetz DD, Sullivan PM, Johnston RC. Results of Charnley total hip arthroplasty at a minimum of thirty years. A concise follow-up of a previous report. J Bone Joint Surg (Am) 2004; 86:690-5.
- Campbell P, Doorn P, Dorey F, Amstutz HC. Wear and morphology of ultra-high molecular weight polyethylene wear particles from total hip replacements. Proc Inst Mech Eng [H ] 1996; 210:167-74.
- Carr AM, DeSteiger R. Osteolysis in patients with a metal-on-metal hip arthroplasty. ANZ J Surg 2008; 78:144-7.
- Catelas I, Bobyn JD, Medley JB, Krygier JJ, Zukor DJ, Petit A, Huk OL. Effects of digestion protocols on the isolation and characterization of metal-metal wear particles. I. Analysis of particle size and shape. J Biomed Mater Res 2001; 55:320-9.
- Cervantes C, Campos-Garcia J, Devars S, Gutierrez-Corona F, Loza-Tavera H, Torres-Guzman JC, Moreno-Sanchez R. Interactions of chromium with microorganisms and plants. FEMS Microbiol Rev 2001; 25:335-47.
- Charnley J. The long-term results of low-friction arthroplasty of the hip performed as a primary intervention. J Bone Joint Surg (Br) 1972; 54:61-76.
- Cobb AG, Schmalzreid TP. The clinical significance of metal ion release from cobalt-chromium metal-on-metal hip joint arthroplasty. Proc Inst Mech Eng [H ] 2006; 220:385-98.
- Davies AP, Sood A, Lewis AC, Newson R, Learmonth ID, Case CP. Metal-specific differences in levels of DNA damage caused by synovial fluid recovered at revision arthroplasty. J Bone Joint Surg (Br) 2005a; 87:1439-44.
- Davies AP, Willert HG, Campbell PA, Learmonth ID, Case CP. An unusual lymphocytic perivascular infiltration in tissues around contemporary metal-on-metal joint replacements. J Bone Joint Surg (Am) 2005b; 87:18-27.
- Delaunay CP, Bonnomet F, Clavert P, Laffargue P, Migaud H. THA using metal-on-metal articulation in active patients younger than 50 years. Clin Orthop 2008; (466):340-6.
- Dobbs HS, Minski MJ. Metal ion release after total hip replacement. Biomaterials 1980; 1:193-8.
- Doorn PF, Campbell PA, Amstutz HC. Metal versus polyethylene wear particles in total hip replacements. A review. Clin Orthop (Suppl) 1996; (329):S206-S216.
- Doorn PF, Campbell PA, Worrall J, Benya PD, McKellop HA, Amstutz HC. Metal wear particle characterization from metal on metal total hip replacements: transmission electron microscopy study of periprosthetic tissues and isolated particles. J Biomed Mater Res 1998; 42:103-11.
- Dorr LD, Bloebaum R, Emmanual J, Meldrum R. Histologic, biochemical, and ion analysis of tissue and fluids retrieved during total hip arthroplasty. Clin Orthop 1990; (261):82-95.
- Dumbleton JH, Manley MT. Metal-on-Metal total hip replacement: what does the literature say? J Arthroplasty 2005; 20:174-88.
- Evans EM, Freeman MA, Miller AJ, Vernon-Roberts B. Metal sensitivity as a cause of bone necrosis and loosening of the prosthesis in total joint replacement. J Bone Joint Surg (Br) 1974; 56-:626-42.
- Ferreira ME, de Lourdes PM, Garcia e Costa, Sousa JP, de Carvalho GS. Comparative study of metallic biomaterials toxicity: a histochemical and immunohistochemical demonstration in mouse spleen. J Trace Elem Med Biol 2003; 17:45-9.
- Firkins PJ, Tipper JL, Saadatzadeh MR, Ingham E, Stone MH, Farrar R, Fisher J. Quantitative analysis of wear and wear debris from metal-on-metal hip prostheses tested in a physiological hip joint simulator. Biomed Mater Eng 2001; 11:143-57.
- Fisher J, Jin Z, Tipper J, Stone M, Ingham E. Tribology of alternative bearings. Clin Orthop 2006; (453):25-34.
- Garrett R, Wilksch J, Vernon-Roberts B. Effects of cobalt-chrome alloy wear particles on the morphology, viability and phagocytic activity of murine macrophages in vitro. Aust J Exp Biol Med Sci 1983; 61(Pt 3):355-69.
- Germain MA, Hatton A, Williams S, Matthews JB, Stone MH, Fisher J, Ingham E. Comparison of the cytotoxicity of clinically relevant cobalt-chromium and alumina ceramic wear particles in vitro. Biomaterials 2003; 24:469-79.
- Goodman SB. Wear particles, periprosthetic osteolysis and the immune system. Biomaterials 2007a; 28:5044-8.
- Goodman SB. Wear particles, periprosthetic osteolysis and the immune system. Biomaterials 2007b; 28:5044-8.
- Granchi D, Cenni E, Ciapetti G, Savarino L, Stea S, Gamberini S, Gori A, Pizzoferrato A. Cell death induced by metal ions: necrosis or apoptosis? J Mater Sci Mater Med 1998; 9:31-7.
- Graves S, Davidson D, de Steiger R, Tomkins A, Ryan P, Griffith L, McDermott B, Pratt N, Miller L, Stanford T. Australian Orthopaedic Association, National Joint Replacement Registry, Anual Report 2008, Hip and Knee Arthroplasty, September 1999 to December 2007.
- Hallab NJ, Mikecz K, Jacobs JJ. A triple assay technique for the evaluation of metal-induced, delayed-type hypersensitivity responses in patients with or receiving total joint arthroplasty. J Biomed Mater Res 2000; 53:480-9.
- Hallab NJ, Caicedo M, Epstein R, McAllister K, Jacobs JJ. In vitro reactivity to implant metals demonstrates a person-dependent association with both T-cell and B-cell activation. J Biomed Mater Res A 2010; 92:667-82.
- Hart AJ, Hester T, Sinclair K, Powell JJ, Goodship AE, Pele L, Fersht NL, Skinner J. The association between metal ions from hip resurfacing and reduced T-cell counts. J Bone Joint Surg (Br) 2006; 88:449-54.
- Holzwarth U, Thomas P, Kachler W, Goske J, Schuh A. Metallurgical differentiation of cobalt-chromium alloys for implants. Orthopade 2005; 34:1046-51.
- Hosman AH, van der Mei HC, Bulstra SK, Busscher HJ, Neut D. Metal-on-metal bearings in total hip arthroplasties: Influence of cobalt and chromium ions on bacterial growth and biofilm formation. J Biomed Mater Res A 2009; 88:711-6.
- Howie DW. Tissue response in relation to type of wear particles around failed hip arthroplasties. J Arthroplasty 1990; 5:337-48.
- Huber M, Reinisch G, Trettenhahn G, Zweymuller K, Lintner F. Presence of corrosion products and hypersensitivity-associated reactions in periprosthetic tissue after aseptic loosening of total hip replacements with metal bearing surfaces. Acta Biomater 2009; 5:172-80.
- Huk OL, Catelas I, Mwale F, Antoniou J, Zukor DJ, Petit A. Induction of apoptosis and necrosis by metal ions in vitro. J Arthroplasty 2004; 19:84-7.
- Jacobs JJ, Campbell PA, Konttinen T. How has the biologic reaction to wear particles changed with newer bearing surfaces? J Am Acad Orthop Surg (Suppl 1) 2008; 16:S49-S55.
- Jakobsen SS, Danscher G, Stoltenberg M, Larsen A, Bruun JM, Mygind T, Kemp K, Soballe K. Cobalt-chromium-molybdenum alloy causes metal accumulation and metallothionein up-regulation in rat liver and kidney. Basic Clin Pharmacol Toxicol 2007; 101:441-6.
- Jones DA, Lucas HK, O'Driscoll M, Price CH, Wibberley B. Cobalt toxicity after McKee hip arthroplasty. J Bone Joint Surg (Br) 1975; 57:289-96.
- Kamaludeen SP, Megharaj M, Juhasz AL, Sethunathan N, Naidu R. Chromium-microorganism interactions in soils: remediation implications. Rev Environ Contam Toxicol 2003; 178:93-164.
- Keegan GM, Learmonth ID, Case CP. Orthopaedic metals and their potential toxicity in the arthroplasty patient: A review of current knowledge and future strategies. J Bone Joint Surg (Br) 2007; 89:567-73.
- Komeda H, Kobayashi M, Shimizu S. A novel transporter involved in cobalt uptake. Proc Natl Acad Sci U S A 1997; 94:36-41.
- Korovessis P, Petsinis G, Repanti M, Repantis T. Metallosis after contemporary metal-on-metal total hip arthroplasty. Five to nine-year follow-up. J Bone Joint Surg (Am) 2006; 88:1183-91.
- Kothamasi D, Kothamasi S. Cobalt interference in iron-uptake could inhibit growth in Pseudomonas aeruginosa. World J Microbiol Biotech 2004; 20:755-8.
- Ladon D, Doherty A, Newson R, Turner J, Bhamra M, Case CP. Changes in metal levels and chromosome aberrations in the peripheral blood of patients after metal-on-metal hip arthroplasty. J Arthroplasty 2004; 19:78-83.
- Lazennec JY, Boyer P, Poupon J, Rousseau MA, Roy C, Ravaud P, Catonne Y. Outcome and serum ion determination up to 11 years after implantation of a cemented metal-on-metal hip prosthesis. Acta Orthop 2009; 80:168-73.
- Leslie I, Williams S, Brown C, Isaac G, Jin Z, Ingham E, Fisher J. Effect of bearing size on the long-term wear, wear debris, and ion levels of large diameter metal-on-metal hip replacements-An in vitro study. J Biomed Mater Res B Appl Biomater 2008; 87:163-72.
- Leslie IJ, Williams S, Brown C, Anderson J, Isaac G, Hatto P, Ingham E, Fisher J. Surface engineering: A low wearing solution for metal-on-metal hip surface replacements. J Biomed Mater Res B Appl Biomater 2009; 90B:558-65.
- Levy SB. Active efflux, a common mechanism for biocide and antibiotic resistance. Symp Ser Soc Appl Microbiol 2002; (31):65S-71S.
- Lloyd JR, Lovley DR. Microbial detoxification of metals and radionuclides. Curr Opin Biotechnol 2001; 12:248-53.
- Lundborg M, Falk R, Johansson A, Kreyling W, Camner P. Phagolysosomal pH and dissolution of cobalt oxide particles by alveolar macrophages. Environ Health Perspect 1992; 97:153-7.
- Mabilleau G, Kwon YM, Pandit H, Murray DW, Sabokbar A. Metal-on-metal hip resurfacing arthroplasty: a review of periprosthetic biological reactions. Acta Orthop 2008; 79:734-47.
- MacDonald SJ, Brodner W, Jacobs JJ. A consensus paper on metal ions in metal-on-metal hip arthroplasties. J Arthroplasty 2004; 19:12-6.
- McKee GK, Watson-Farrar J. Replacement of arthritic hips by the McKee-Farrar prosthesis. J Bone Joint Surg (Br) 1966; 48:245-59.
- Milosev I, Remskar M. In vivo production of nanosized metal wear debris formed by tribochemical reaction as confirmed by high-resolution TEM and XPS analyses. J Biomed Mater Res A 2008; 91:1100-10.
- Minoda Y, Kobayashi A, Sakawa A, Aihara M, Tada K, Sugama R, Iwakiri K, Ohashi H, Takaoka K. Wear particle analysis of highly crosslinked polyethylene isolated from a failed total hip arthroplasty. J Biomed Mater Res B Appl Biomater 2008; 86B:501-5.
- Mnatsakanov ST. Effect of cobalt chloride on the antibiotic sensitivity of Staphylococcus. Antibiotiki 1967; 12:161-2.
- Muthukumar N, Rajasekar A, Ponmariappan S, Mohanan S, Maruthamuthu S, Muralidharan S, Subramanian P, Palaniswamy N, Raghavan M. Microbiologically influenced corrosion in petroleum product pipelines--a review. Indian J Exp Biol 2003; 41:1012-22.
- Nies DH. The cobalt, zinc, and cadmium efflux system CzcABC from Alcaligenes eutrophus functions as a cation-proton antiporter in Escherichia coli. J Bacteriol 1995; 177:2707-12.
- Nies DH. Efflux-mediated heavy metal resistance in prokaryotes. FEMS Microbiol Rev 2003; 27:313-39.
- Nies DH, Nies A, Chu L, Silver S. Expression and nucleotide sequence of a plasmid-determined divalent cation efflux system from Alcaligenes eutrophus. Proc Natl Acad Sci U S A 1989; 86:7351-5.
- Nyren O, McLaughlin JK, Gridley G, Ekbom A, Johnell O, Fraumeni JF, Jr., Adami HO. Cancer risk after hip replacement with metal implants: a population-based cohort study in Sweden. J Natl Cancer Inst 1995; 87:28-33.
- Ogunwale B, Schmidt-Ott A, Meek RM, Brewer JM. Investigating the immunologic effects of CoCr nanoparticles. Clin Orthop 2009; (467):3010-6.
- Onda K, Nagoya S, Kaya M, Yamashita T. Cup-neck Impingement Due to the Malposition of the Implant as a Possible Mechanism for Metallosis in Metal-on-metal Total Hip Arthroplasty. Orthopedics 2008; 31:396.
- Oparaugo PC, Clarke IC, Malchau H, Herberts P. Correlation of wear debris-induced osteolysis and revision with volumetric wear-rates of polyethylene: a survey of 8 reports in the literature. Acta Orthop Scand 2001; 72:22-8.
- Papageorgiou I, Yin Z, Ladon D, Baird D, Lewis AC, Sood A, Newson R, Learmonth ID, Case CP. Genotoxic effects of particles of surgical cobalt chrome alloy on human cells of different age in vitro. Mutat Res 2007; 619:45-58.
- Pollard TC, Baker RP, Eastaugh-Waring SJ, Bannister GC. Treatment of the young active patient with osteoarthritis of the hip. A five- to seven-year comparison of hybrid total hip arthroplasty and metal-on-metal resurfacing. J Bone Joint Surg (Br) 2006; 88:592-600.
- Postel M, Langlais F. The wear and tear of stellite total hip prostheses: in vivo studies and clinical results. Rev Chir Orthop Reparatrice Appar Mot 1977; (63 Suppl 2):84-94.
- Pourzal R, Theissmann R, Morlock M, Fischer A. Micro-structural alterations within different areas of articulating surfaces of a metal-on-metal hip resurfacing system. Wear 2009; 267:689-94.
- Rae T. A study on the effects of particulate metals of orthopaedic interest on murine macrophages in vitro. J Bone Joint Surg (Br) 1975; 57:444-50.
- Reinisch G, Judmann KP, Lhotka C, Lintner F, Zweymuller KA. Retrieval study of uncemented metal-metal hip prostheses revised for early loosening. Biomaterials 2003; 24:1081-91.
- Ring PA. Complete replacement arthroplasty of the hip by the ring prosthesis. J Bone Joint Surg (Br) 1968; 50:720-31.
- Rothwell AG, Hobbs T, Frampton C. New Zealand Orthopaedic Association, The New Zealand Joint Registry, Nine Year Report, January 1999 to December 2007.
- Ruiz N, Montero T, Hernandez-Borrell J, Vinas M. The role of Serratia marcescens porins in antibiotic resistance. Microb Drug Resist 2003; 9:257-64.
- Savarino L, Stea S, Granchi D, Visentin M, Ciapetti G, Donati ME, Rollo G, Zinghi G, Pizzoferrato A, Montanaro L, Toni A. Sister chromatid exchanges and ion release in patients wearing fracture fixation devices. J Biomed Mater Res 2000; 50:21-6.
- Savarino L, Padovani G, Ferretti M, Greco M, Cenni E, Perrone G, Greco F, Baldini N, Giunti A. Serum ion levels after ceramic-on-ceramic and metal-on-metal total hip arthroplasty: 8-year minimum follow-up. J Orthop Res 2008; 26:1569-76.
- Scales JT, Wilson JN. Some aspects of the development of the Stanmore total hip joint prosthesis. Reconstr Surg Traumatol 1969; 11:20-39.
- Scales JT, Winter GD, Shirley HT. Corrosion of orthopaedic implants. Smith-Petersen type hip nails. Br Med J 1961; 2:478-82.
- Schmalzried TP, Peters PC, Maurer BT, Bragdon CR, Harris WH. Long-duration metal-on-metal total hip arthroplasties with low wear of the articulating surfaces. J Arthroplasty 1996; 11:322-31.
- Shanbhag A, Yang J, Lilien J, Black J. Decreased neutrophil respiratory burst on exposure to cobalt-chrome alloy and polystyrene in vitro. J Biomed Mater Res 1992; 26:185-95.
- Shimmin A, Beaule PE, Campbell P. Metal-on-metal hip resurfacing arthroplasty. J Bone Joint Surg (Am) 2008; 90:637-54.
- Sieber HP, Rieker CB, Kottig P. Analysis of 118 second-generation metal-on-metal retrieved hip implants. J Bone Joint Surg (Br) 1999; 81:46-50.
- Silva M, Heisel C, Schmalzried TP. Metal-on-metal total hip replacement. Clin Orthop 2005; (430):53-61.
- Silver S, Misra TK. Plasmid-mediated heavy metal resistances. Annu Rev Microbiol 1988; 42:717-43.
- Silver S, Phung LT. Bacterial heavy metal resistance: new surprises. Annu Rev Microbiol 1996; 50:753-89.
- Sivash KM. The development of a total metal prosthesis for the hip joint from a partial joint replacement. Reconstr Surg Traumatol 1969; 11:53-62.
- Smethurst E, Waterhouse RB. Causes of failure in total hip prostheses. J Mater Sci 1977; 12:1781-92.
- Stilling M, Nielsen KA, Soballe K, Rahbek O. Clinical comparison of polyethylene wear with zirconia or cobalt-chromium femoral heads. Clin Orthop 2009; (467):2644-50.
- Tipper JL, Firkins PJ, Ingham E, Fisher J, Stone MH, Farrar R. Quantitative analysis of the wear and wear debris from low and high carbon content cobalt chrome alloys used in metal on metal total hip replacements. J Mater Sci Mater Med 1999; 10:353-62.
- Trampuz A, Widmer AF. Infections associated with orthopedic implants. Curr Opin Infect Dis 2006; 19:349-56.
- Ug A, Ceylan O. Occurrence of resistance to antibiotics, metals, and plasmids in clinical strains of Staphylococcus spp. Arch Med Res 2003; 34:130-6.
- Urban RM, Jacobs JJ, Tomlinson MJ, Gavrilovic J, Black J, Peoc'h M. Dissemination of wear particles to the liver, spleen, and abdominal lymph nodes of patients with hip or knee replacement. J Bone Joint Surg (Am) 2000; 82:457-76.
- Urban RM, Tomlinson MJ, Hall DJ, Jacobs JJ. Accumulation in liver and spleen of metal particles generated at nonbearing surfaces in hip arthroplasty. J Arthroplasty 2004; 19:94-101.
- Vendittoli PA, Ganapathi M, Lavigne M. Blood and urine metal ion levels in young and active patients after Birmingham hip resurfacing arthroplasty. J Bone Joint Surg (Br) 2007; 89:989-90.
- Vincent KR, Vincent HK, Lee LW, Weng J, Alfano AP. Outcomes after inpatient rehabilitation of primary and revision total hip arthroplasty. Arch Phys Med Rehabil 2006; 87:1026-32.
- Virtanen S. Metal release mechanisms in hip replacement. Acta Orthop 2006; 77:695-6.
- Visuri T, Pukkala E, Paavolainen P, Pulkkinen P, Riska EB. Cancer risk after metal on metal and polyethylene on metal total hip arthroplasty. Clin Orthop (Suppl) 1996; 329:S280-S289.
- Wagner M, Wagner H. Medium-term results of a modern metal-on-metal system in total hip replacement. Clin Orthop 2000; (379):123-33.
- Weightman BO, Zarek JM, Bingold AC. Corrosion of a cobalt-chromium-molybdenum orthopaedic implant. A preliminary report. Med Biol Eng 1969; 7:679.
- Willert HG, Buchhorn GH, Gobel D, Koster G, Schaffner S, Schenk R, Semlitsch M. Wear behavior and histopathology of classic cemented metal on metal hip endoprostheses. Clin Orthop (Suppl) 1996; (329):S160-S186.
- Willert HG, Buchhorn GH, Fayyazi A, Flury R, Windler M, Koster G, Lohmann CH. Metal-on-metal bearings and hypersensitivity in patients with artificial hip joints. A clinical and histomorphological study. J Bone Joint Surg (Am) 2005; 87:28-36.
- Williams S, Hardaker C, Leslie IJ, Foughran F, Isaac G, Fisher J. Validation of ion level analysis as method to assess in vitro total hip replacement wear. Tribology 2008a; 2:104-8.
- Williams S, Leslie I, Isaac G, Jin Z, Ingham E, Fisher J. Tribology and wear of metal-on-metal hip prostheses: influence of cup angle and head position. J Bone Joint Surg Am (Suppl 3) 2008b; 90:111-7.
- Wright MS, Loeffler PG, Stepanauskas R, McArthur JV. Bacterial tolerances to metals and antibiotics in metal-contaminated and reference streams. FEMS Microbiol Ecol 2006; 58:293-302.
- Wroblewski BM. Osteolysis due to particle wear debris following total hip arthroplasty: the role of high-density polyethylene. Instr Course Lect 1994; 43:289-94.
- Wroblewski BM, Siney PD, Fleming PA. Charnley low-friction arthroplasty: survival patterns to 38 years. J Bone Joint Surg (Br) 2007; 89:1015-8.
- Yan Y, Neville A, Dowson D. Understanding the role of corrosion in the degradation of metal-on-metal implants. Proc Inst Mech Eng [H ] 2006; 220:173-81.
- Yan Y, Neville A, Dowson D, Williams S, Fisher J. Effect of metallic nanoparticles on the biotribocorrosion behaviour of Metal-on-Metal hip prostheses. Wear 2009; 267:683-68.
- Zimmerli W, Waldvogel FA, Vaudaux P, Nydegger UE. Pathogenesis of foreign body infection: description and characteristics of an animal model. J Infect Dis 1982; 146:487-97.
- Zimmerli W, Lew PD, Waldvogel FA. Pathogenesis of foreign body infection. Evidence for a local granulocyte defect. J Clin Invest 1984; 73:1191-200.
- Zimmerli W, Trampuz A, Ochsner PE. Prosthetic-joint infections. N Engl J Med 2004; 351:1645-54.