Abstract
Testicular spermatozoa are utilized to achieve pregnancy in couples with severe male factor infertility. Several studies suggest that aneuploidy rates in spermatozoa are elevated at the testicular level in infertile patients compared to ejaculates of normal controls. However, essential data regarding aneuploidy rates between ejaculated and testicular spermatozoa in the same individuals is lacking. The purpose of our study was to compare aneuploidy rates at the testicular and post-testicular level from the same patients with persistently high sperm DNA damage. Ejaculates and testicular biopsies were obtained from eight patients with persistently high DNA damage (>30%). Both ejaculated and testicular samples were analyzed for sperm DNA damage and sperm aneuploidy for chromosomes 13, 18, 21, X, and Y. In addition, semen samples from ten normozoospermic men presenting for fertility evaluation served as a control group. A strong correlation between the alteration of spermatogenesis and chromatin deterioration was observed in our study. In the same individuals, testicular samples showed a significantly lower DNA damage compared to ejaculated spermatozoa (14.9% ± 5.0 vs. 40.6% ± 14.8, P < 0.05), but significantly higher aneuploidy rates for the five analyzed chromosomes (12.41% ± 3.7 vs. 5.77% ± 1.2, P < 0.05). While testicular spermatozoa appear favourable for ICSI in terms of lower DNA damage, this potential advantage could be offset by the higher aneuploidy rates in testicular spermatozoa.
Introduction
Since the introduction of intracytoplasmic sperm injection (ICSI), many cases of severe male infertility can now be successfully treated. In most cases of assisted reproductive techniques (ART), male infertility evaluation is often limited to semen analysis, without taking into account that it can be associated with sperm DNA damage, improper meiotic recombination, epigenetic alterations, and elevated sperm chromosome aneuploidy [Carrell Citation2008b]. There is a renewed interest in the use of tests beyond semen analysis due in part to the increase in the number of reports indicating an elevated risk of congenital anomalies in children born after ART [Bonduelle et al. Citation2002; The ESHRE Group 2007; Verpoest and Tournaye Citation2006]. Higher levels of sperm aneuploidy have been reported in infertile men compared to donors with proven fertility [Egozcue et al. Citation2003]. Sperm chromosome aneuploidies are increased in patients with severe oligoasthenoteratozoospermia (OAT), and have been reported to contribute to chromosomal abnormalities in embryos, repeated IVF/ICSI failures, recurrent pregnancy losses, as well as unexplained infertility [Gianaroli et al. Citation2005; Martin Citation2006].
In addition, significantly different levels of DNA damage in spermatozoa between fertile and infertile men suggests that sperm DNA damage could be used as a predictor of fertility potential [Spano et al. Citation2000; Evenson et al. Citation2002]. Sperm DNA damage ≥ 30% has been shown to be associated with a fair to poor fertility potential using flow-cytometry based SCSA® [Evenson et al. Citation2002]. A reference value of 20% terminal deoxynucleotidyl transferase-mediated deoxyuridine triphosphate nick end labeling (TUNEL)-positive spermatozoa was established to be predictive for ICSI outcomes [Benchaib et al. Citation2003]. Low intra- and inter-observer variability for SCSA® and significant correlation to TUNEL has been reported in a number of studies [Barroso et al. Citation2000; Evenson and Wixon Citation2006].
Several treatment options have been suggested in an attempt to reduce the level of DNA damage, among them, the protective effects of antioxidants on spermatozoa DNA, have been reported [Agarwal et al. Citation2004; Moskovtsev Citation2008]. The protective effects of several antioxidants on the sperm DNA damage have been shown in patients with moderately elevated DNA damage primarily due to oxidative stress [Greco et al. Citation2005a]. However the mechanism of DNA damage is complex and is not limited to oxidative stress [Aitken et al. Citation1998]. The nature of DNA damage, failure of the antioxidant system, or enzyme production in some patients may be responsible for unsuccessful antioxidant treatment in some men, including patients in this group. It has been shown that testicular samples exhibit significantly lower DNA damage compared to ejaculate spermatozoa [Greco et al. Citation2005b; Moskovtsev et al. Citation2010].
Testicular spermatozoa have been utilized to achieve pregnancy in couples with severe male factor infertility, with reported pregnancy rates similar to ejaculated spermatozoa, according to meta-analysis [Nicopoullos et al. Citation2004]. However, it has also been reported that different pregnancy rates could be expected if testicular spermatozoa originated from patients with obstructive azoospermia (OA) or non-obstructive azoospermia (NOA) [Vernaeve et al. Citation2003]. Several studies suggest that testicular spermatozoa have higher aneuploidy rates in patients with different aetiologies of infertility compared to ejaculates of normal controls [Bernardini et al. Citation2000; Levron et al. Citation2001; Burrello et al. Citation2002; Mateizel et al. Citation2002; Palermo et al. Citation2002; Gianaroli et al. Citation2005]. However, the essential data on aneuploidy rates between ejaculated and testicular spermatozoa in the same individuals is lacking. The purpose of this study was to compare aneuploidy rates at the testicular and post-testicular level from the same patients with persistently high sperm DNA damage despite prior three-month oral antioxidant treatment of daily vitamins (C 500 mg, E 100 IUS, folic acid 1 mg) supplemented with 20 mg of zinc and 200 µg selenium.
Results
There was no significant difference in age between patient and control groups (38.8 ± 4.1 vs. 36.5 ± 2.3, respectively). When compared to ejaculates from controls, patients had significantly lower sperm concentration and motility, with higher sperm DNA damage and aneuploidy rates for chromosomes 13, 21, X, and Y in ejaculated spermatozoa, P < 0.05 (; Fig. 1).
Table 1. Comparison of semen analyses, DNA damage, and aneuploidy rates between controls and patients.
When ejaculated and testicular spermatozoa obtained from the same patients were compared, ejaculated spermatozoa showed almost a threefold increase in DNA damage in comparison to testicular samples (40.6 ± 14.8 vs. 14.9 ± 5.0 respectively, P < 0.05). Surprisingly, while testicular spermatozoa had lower DNA damage, we observed significantly higher aneuploidy rates of chromosomes 18, 21, X, Y; as well as total aneuploidy for the five analyzed chromosomes P < 0.05 (). Testicular spermatozoa had more than a threefold increase in aneuploidy rates for sex chromosomes, an almost twofold increase for chromosomes 18 and 21, and more than a twofold increase for total aneuploidy in comparison to ejaculates. The aneuploidy for chromosome 13 was not significantly different between both types of samples.
When the categories of sperm aneuploidy were defined, testicular spermatozoa showed a twofold increase in testicular aneuploidies for disomies 18 and 21, a threefold increase for both nullisomies and disomies for sex chromosomes, and a fourfold increase for diploidies, compared to ejaculated spermatozoa (). Among all types of analyzed samples, most commonly found, were diploidy 18,18/XY, monosomies 0/X, 0/Y, 18/0, 0/21, 13/0, and disomies 18,18/X, 18,18/Y, 18/X,Y, 13/21,21, 13,13/21. The ratio of X to Y chromosomes was not significantly different between ejaculates of controls (1.01 ± 0.22), ejaculates of patients (1.04 ± 0.12), or testicular spermatozoa (1.09 ± 0.13).
Table 2. Comparison of aneuploidy types between ejaculated and testicular spermatozoa obtained from the same individual.
Discussion
Chromosomal abnormalities may arise during gametogenesis of either parent or after fertilization, likely resulting in varying degrees of mosaicism. During and after fertilization, spermatozoa contribute genetic and epigenetic factors that affect embryogenesis including oocyte-activation factors, a haploid set of chromosomes, RNA, undamaged DNA, and competent genome with the exact number of essential genes [Carrell 2008a]. The contribution of a haploid set of chromosomes is crucial. An elevated frequency of unbalanced numbers of chromosomes in spermatozoa was reported in men with failed compared to successful ICSI cycles and can be associated with miscarriages, birth defects, and genetic disorders in newborns [Emery and Carrell Citation2006; Nicopoullos et al. Citation2008]. In contrast, it was also reported that increased frequency of sperm aneuploidy in oligozoospermic and teratozoospermic men did not affect fertilization and pregnancy rates or pregnancy losses after IVF [Colombero et al. Citation1999].
While most infertile men have a normal karyotype, it is known that the incidence of karyotypic chromosome abnormalities is higher in infertile males than in the general population. Azoospermic men have even a higher occurrence of karyotypic chromosomal abnormalities in comparison to men with severely diminished sperm parameters [Dul et al. Citation2010]. Preimplantation genetic diagnosis (PGD) could be used to increase pregnancy rates in women at high risk of aneuploidy, such as patients with advanced maternal age, recurrent IVF failures, and pregnancy losses. The rate of chromosomal abnormalities in embryos screened for PGD was reported to be significantly related to aneuploidy in spermatozoa [Tempest et al. Citation2009]. High incidence of mosaicism in embryos derived from testicular spermatozoa of men with NOA possibly due to compromised centrosome structure, were also reported [Silber at al. 2003]. However, it was noted that randomized controlled trials with large patient populations are needed before PGD becomes a routine procedure in IVF clinics [Shahine and Cedars Citation2006].
While several studies have examined aneuploidy rates in testicular spermatozoa in patients with OA or NOA [Bernardini et al. Citation2000; Levron et al. Citation2001; Burrello et al. Citation2002; Mateizel et al. Citation2002; Palermo et al. Citation2002; Gianaroli et al. Citation2005], the concurrent aneuploidy rates in ejaculated and testicular spermatozoa in an individual are unknown. This is the first study comparing aneuploidy rates in ejaculated and testicular spermatozoa collected from the same men on the day of ICSI treatment.
Our results are in agreement with several studies that reported a significant increase in aneuploidy rates in testicular spermatozoa in comparison to ejaculates of normal controls [Bernardini et al. Citation2000; Levron et al. Citation2001; Burrello et al. Citation2002; Mateizel et al. Citation2002; Palermo et al. Citation2002; Rodrigo et al. Citation2004; Gianaroli et al. Citation2005] with an overall level of testicular sperm aneuploidy of 11.01% ± 9.72 (). For instance, testicular aneuploidy rates in patients with NOA were 5.8% for sex chromosomes and 3.2% for chromosome 18 [Mateizel et al. Citation2002]. This is comparable to the testicular aneuploidy rates we observed in patients with high DNA damage, 5.49% for sex chromosomes and 2.07% for chromosome 18. Higher aneuploidy rates in our study for chromosomes 21, X, and Y are in agreement with others, as these chromosomes have a higher tendency to nondisjunction [Carrell et al. Citation2003; Rodrigo et al. Citation2004]. Reduction of recombination and the presence of a single end terminal chiasma between the X and Y at meiosis might be responsible for this observation [Hassold et al. Citation1991; Egozcue et al. Citation2003; Martin Citation2006]. Aneuploidy rates might be even higher in earlier germ-cells such as primary or secondary spermatocytes, reported to be 39% in patients with hypospermatogenesis, 43.5% in men with maturation arrest, and 29.1% in patients with OA [Huang et al. Citation1999].
Figure 1. FISH on sperm AneuVysion LSI (13/21) probe. FISH probes: 13-green, 21-orange.
Normal spermatozoa with haploid number of chromosomes: A) (13/21). Spermatozoa with chromosome aneuploidies: B) disomy 21 (13/21,21); C) nullisomy 13 (0/21); and D) nullisomy 13 with disomy 21 (0/21,21). Excluded: E) overlapping cells and F) not hybridized.
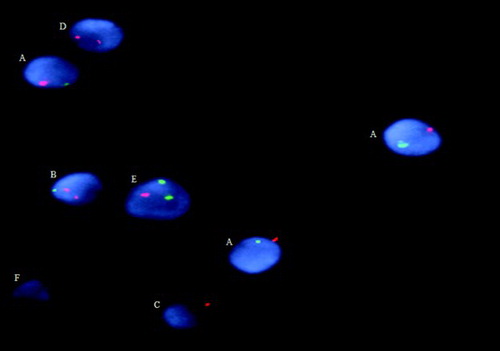
Table 3. Literature review on aneuploidy rates in testicular spermatozoa in comparison to ejaculates of fertile donors or normal controls.
Much higher rates of aneuploidy for chromosomes 1, 17 (43.8%), and sex chromosomes (55.9%) were reported in testicular samples of three patients with NOA [Bernardini et al. Citation2000]. The exceptionally high number of aneuploidy in the study by Bernardini et al. might relate to the small number of spermatozoa analyzed, variation in scoring criteria such as differentiation between dimorphic spermatozoa and immature germ cells, as stated by authors.
In contrast, similar rates of aneuploidies were also reported in testicular samples from infertile patients compared to ejaculate of normal controls [Viville et al. Citation2000; Martin et al. Citation2003; Dakouane et al. Citation2005]. In a study by Martin et al.[2003] in six patients with NOA the mean testicular aneuploidy rate for sex chromosomes was 2.37%, however, in one patient aneuploidy was as high as 8.12%. Curiously, despite NOA, all their cases had normal levels of FSH and more than 9,000 spermatozoa available for analysis from each biopsy [Martin et al. Citation2003]; it appears that those patients did not have severe defects of spermatogenesis. In the study by Dakouane and colleagues, aneuploidy for chromosomes 18, X, and Y were at 1.1% for ten patients with OA, similar to the 1.29% aneuploidy rates they observed in testes from 35 deceased men. However, in four subjects with arrested spermatogenesis, aneuploidy was as high as 14.28% indicating a strong relationship of aneuploidy to an abnormal testicular microenvironment [Dakouane et al. Citation2005]. Similar aneuploidy rates for chromosomes 1, X/Y were reported in 11 patients with CBAVD (6.8% ± 3.9) in comparison to two controls (4.4% and 5.4%) [Viville et al. Citation2000], however aneuploidy in their controls was noticeably higher than in previous reports. Some of the variability between studies may be explained by the small number of subjects, limited number of spermatozoa available for evaluation in patients with NOA, as well as the different aetiologies of azoospermia.
In the study presented above, testicular spermatozoa had a lower degree of DNA damage than ejaculated spermatozoa, which supports the previous reports [Greco et al. Citation2005b; Moskovtsev et al. Citation2010]. In the study by Greco and colleagues, testicular spermatozoa had DNA damage of 4.8% compared to 23.6% of ejaculated spermatozoa. The pregnancy rate after ICSI with testicular spermatozoa was 44% versus 6% using ejaculated spermatozoa and the implantation rate was 22% versus 2% (one pregnancy which spontaneously aborted), respectively [Greco et al. Citation2005b].
Testicular germ cell apoptosis occurs normally and continuously throughout life. Apoptosis during spermatogenesis is responsible for the removal of up to 75% of potential spermatozoa. This helps to maintain an adequate Sertoli cell to germ cell ratio to support normal spermatogenesis [Sinha Hikim and Swerdloff Citation1999]. The inadequate support or loss of support by Sertoli cells may magnify the effect of DNA damage in spermatozoa, which would increase with the time of spermatozoa release from the support cells and could explain the lower degree of DNA damage in testicular spermatozoa [Tesarik et al. Citation2004].
The degree of aneuploidy in testicular spermatozoa observed in our patients with persistent high DNA damage was similar to the levels of aneuploidy reported in patients with non-obstructive azoospermia (NOA) [Levron et al. Citation2001; Mateizel et al. Citation2002; Palermo et al. Citation2002; Gianaroli et al. Citation2005], indicating a possible similarity in the nature of impaired spermatogenesis in these two groups of patients. Compromised testicular endocrine environment and incomplete maturation of spermatozoa might in part explain elevated testicular aneuploidy [Rodrigo et al. Citation2011]. Any alterations in spermatogenesis could lead to vulnerability of chromosomes to meiotic errors during cell division and proliferation [Egozcue et al. Citation2003]. Defects in meiotic pairing and recombination could cause meiotic arrest manifested as azoospermia or chromosomal non-disjunction, leading to the production of unbalanced spermatozoa [Martin Citation2006; Topping et al. Citation2006]. Defective recombination has also been reported to activate meiotic checkpoints and initiate the apoptotic cascade associated with sperm DNA damage [Gonsalves et al. Citation2004]. In addition, mis-segregated chromosomes may themselves trigger the apoptotic process [Dobles et al. Citation2000].
Sperm DNA damage may be part of a genomic surveillance mechanism to control or maintain normal production of spermatozoa and to inactivate and reject spermatozoa with altered or an unbalanced genome [Muriel et al. Citation2007]. This theory might explain the strong relationship between aneuploidy and sperm DNA damage that we observed in our study, and as reported by others [Carrell et al. Citation2003; Muriel et al. Citation2007]. While germ-cell apoptosis would eliminate unbalanced cells at earlier stages of spermatogenesis, some cells would be able to escape this potential control mechanism leading to continued presence of aneuploidy in some mature testicular or ejaculated spermatozoa [Hassold et al. Citation1991]. While testicular spermatozoa appear favourable for ICSI in terms of lower DNA damage, this potential advantage could be offset by the higher aneuploidy rates we found in testicular spermatozoa. A randomized controlled study is needed to confirm our results of increased aneuploidy in testicular spermatozoa in a larger patient population with different aetiologies of male infertility.
Materials and Methods
Selection of Subjects
The study was approved by the Institutional Research Ethics Boards of both Sunnybrook Health Sciences Centre and Mount Sinai Hospital. Patients with persistently high sperm DNA damage after an ineffective course of oral antioxidant treatment were referred to ICSI with testicular spermatozoa. The three-month course of antioxidant treatment of daily vitamins (C 500 mg, E 100 IUS, folic acid 1 mg) supplemented with 20 mg of zinc and 200 µg selenium was administered as previously reported [Moskovtsev et al. Citation2010]. Ejaculated and testicular spermatozoa were obtained immediately prior to ICSI treatment, however the number of subjects was limited to eight patients who met the inclusion criteria for the study and had excess testicular samples which could be utilized for sperm DNA fragmentation and aneuploidy assessments.
Semen samples were collected by masturbation on site after 2 to 5 d of sexual abstinence. The semen was allowed to liquefy for at least 20 min and was analyzed within 60 min of collection. A routine semen analysis was performed with evaluation of semen volume, sperm concentration, and sperm motility according to WHO [1999] guidelines. Testicular spermatozoa were extracted using a standard open testicular spermatozoa extraction (TESE) technique under local anaesthesia as previously described [Schlegel Citation1999]. No complications related to TESE procedure were seen in the patients. In addition, semen samples from ten normozoospermic men, presenting to the Andrology Laboratory for fertility evaluation were included in the study as a control group. All chemicals were obtained from Sigma-Aldrich Ltd. (Oakville, Ontario, Canada) unless otherwise stated.
Sperm DNA fragmentation assessment by TUNEL
A slide based TUNEL technique for the assessment of sperm DNA damage was performed as previously described [Benchaib et al. Citation2003; Moskovtsev et al. Citation2010]. Briefly, 10 µL of washed in PBS samples were spread out over the slides. Slides were processed for TUNEL using the In-Situ Cell Death Detection POD Kit (Roche Diagnostics GmbH, Penzberg, Germany) following manufacturer's instructions. Samples were fixed in 4% paraformaldehyde, blocked, and permeabilized in 0.1% Triton X-100 and 0.1% sodium citrate. Slides were incubated with the TUNEL reaction mixture of enzyme and labeling solutions following incubation with converter-POD in the dark humidified chamber and revealed with DAB chromogen (DAB Metal Enhanced Substrate Set, Roche Diagnostics GmbH) and counterstained with 0.5% methyl green. The sperm with fragmented DNA had nuclei stained in brown, while the nuclei of undamaged cells were stained light green. Two to five hundred cells were analyzed on each slide using X 100 oil immersion objective of a clinical microscope (Olympus Corporation, Tokyo, Japan).
The small amounts of testicular biopsies were processed as described previously for TUNEL assay [Moskovtsev et al. Citation2010]. Testicular cell suspensions were then smeared on a slide and processed for the TUNEL assay as for ejaculated spermatozoa.
Sperm aneuploidy assessment by FISH
Semen samples were thawed at 37°C, washed twice in PBS by centrifugation at 1,200g for 10 min then fixed in −20°C Carnoy's solution (3:1 methanol/acetic acid). Slides were prepared by applying 10 µL of the sample and allowed to air dry. They were then dehydrated in a series of increasing concentrations of ethylene alcohol (70%, 85%, and 100%) for 2 min each. Sperm decondensation and denaturation was completed by incubation of slides with 0.5M NaOH at room temperature for 4 min [Pellestor et al. Citation2003]. Sperm aneuploidy was assessed with the AneuVysion Multicolour DNA Probe Kit (Vysis CEP 18/X, Y - alpha satellite/LSI 13/21) (Abbott Laboratories, Abbott Park, IL, USA) following the manufacturer's instructions. Briefly, centromere enumerating probe (CEP) detects alpha satellite sequences in the centromeric regions of chromosomes 18, X, and Y; while LSI detects chromosome regions 13q14 and 21q22.13- 21q22.2. Slides were hybridized at 37°C for 16 h in ThermoBrite Slide Processing System (Iris International Inc, Westwood, MA, USA). A series of post-hybridization washes were performed to remove unbound DNA probe. Total nuclear DNA was counterstained with DAPI (Abbott Laboratories).
Testicular biopsies were processed for the FISH evaluation as follows: tissue was processed with fine scissors, further dissected using fine gauge needles, tubules were squeezed under X 20 magnification of a stereomicroscope (Leica MZ9.5, Heerbrugg, Switzerland), 10 µL of cell suspension were smeared on the slide and processed for FISH in the same manner as for ejaculated spermatozoa. Slides were visualized using an Olympus BX61 fluorescent microscope (Olympus Corporation, Tokyo, Japan) with oil immersion objective X 100 and dual band-pass filter system and equipped with DIC (Chroma Technology Corp, Bellows Falls, VT, USA) and Hamamatsu C8484-15 digital camera (Hamamatsu Corporation, Hamamatsu City, Japan). On average, more ejaculated spermatozoa were evaluated from the control group (1768 ± 188), than ejaculates of patients (816 ± 160) or testicular spermatozoa (409 ± 93); the numbers were sufficient to provide 80% power at alpha of 0.017 to detect statistical differences among three analyzed groups. Hybridization rates were not statistically significant between two analyzed types of samples: 92% for ejaculate and 87% for testicular spermatozoa.
Aneuploidies in spermatozoa were scored according to previously described criteria for somatic nuclei [Blanco et al. Citation1996]. Briefly, two signals for the same chromosome were separated from each other by at least a single domain. Unbalanced spermatozoa were classified as disomic (two fluorescent signals with similar size and intensity for the analyzed chromosome), diploid (presence of two signals for each of the analyzed chromosomes), or nullisomic (absence of a signal for one chromosome with presence of signal for concurrently analyzed chromosome). Each semen sample was analyzed by two sets of FISH probes for chromosomes 18/X,Y and 13/21. For the first probe set the following types of aneuploidies were observed: nullisomy for chromosome 18: 0/X; 0/Y; 0/X,X; 0/Y,Y; 0/X,Y; nullisomy for sex chromosomes: 18/0; 1818/0; disomy for chromosome 18: 18,18/0; 18,18/X; 18,18/Y; disomy for sex chromosomes: 18/X,Y; 18/X,X; 18/Y,Y; 0/X,Y; 0/X,X; 0/Y,Y; diploidies were reported separately and included 18,18/X,Y; 18,18/X,X; 18,18/Y,Y. For the second probe, nullisomy for chromosome 13: 0/21; 0/21,21; nullisomy for chromosome 21: 13/0; 13,13/0; disomy for chromosome 13: 13,13/21;13,13/0; disomy for chromosome 21: 13/21,21; 0/21,21; diploidies 13,13/21,21 were recorded separately. Only mature spermatozoa with the presence of the sperm tail visualized by fluorescence or DIC and an elliptical head shape were included in the analysis. Overlapping cells and spermatozoa with no fluorescent signals (not hybridized) were excluded from analysis ().
Data was analyzed using the SPSS 19.0 statistical computer software (SPSS Inc., Chicago, IL, USA). The Shapiro-Wilk test was used to verify normality of the data. It was concluded that the data was normally distributed and ANOVA was an appropriate test for our data analysis followed by t-tests for pairwise comparisons. Thus a one way ANOVA analysis followed by t-tests for pairwise comparisons were used to compare differences in DNA damage and aneuploidy rate of ejaculated and testicular spermatozoa between patients and controls. Results are expressed as mean ± SD.
Declaration of interest: The authors report no conflicts of interest. The authors alone are responsible for the content and writing of the paper.
Abbreviations
ART: | = | assisted reproductive techniques |
CEP: | = | centromere enumerating probe |
DAB: | = | diaminobenzidine |
DAPI: | = | 4',6-diamidino-2-phenylindole |
CBAVD: | = | congenital bilateral absence of the vas deferens |
DIC: | = | differential interference contrast |
DNA: | = | deoxyribonucleic acid |
FISH: | = | fluorescence in situ hybridization |
FSH: | = | follicle-stimulating hormone |
ICSI: | = | intracytoplasmic sperm injection |
IVF: | = | in vitro fertilization |
LSI: | = | locus specific identifier |
NOA: | = | non-obstructive azoospermia |
OA: | = | obstructive azoospermia |
OAT: | = | oligoasthenoteratozoospermia |
PBS: | = | phosphate buffer saline |
PGD: | = | preimplantation genetic diagnosis |
POD: | = | peroxidase |
RNA: | = | ribonucleic acid |
SD: | = | standard deviation |
TESE: | = | testicular spermatozoa extraction |
TUNEL: | = | terminal deoxynucleotidyl transferase-mediated deoxyuridine triphosphate nick end labeling |
WHO: | = | World Health Organization. |
References
- Agarwal, A., Nallella, K.P., Allamaneni, S.S. and Said, T.M. (2004) Role of antioxidants in treatment of male infertility: an overview of the literature. Reprod Biomed Online 8:616–627.
- Aitken, R.J., Gordon, E., Harkiss, D., Twigg, J.P., Milne, P., Jennings, Z. and Irvine, D.S. (1998) Relative impact of oxidative stress on the functional competence and genomic integrity of human spermatozoa. Biol Reprod 59:1037–46.
- Barroso, G., Morshedi, M. and Oehninger, S. (2000) Analysis of DNA fragmentation, plasma membrane translocation of phosphatidylserine and oxidative stress in human spermatozoa. Hum Reprod 15:1338–1344.
- Benchaib, M., Braun, V., Lornage, J., Hadj, S., Salle, B., Lejeune, H. and Guérin, J.F. (2003) Sperm DNA fragmentation decreases the pregnancy rate in an assisted reproductive technique. Hum Reprod 18:1023–1028.
- Bernardini, L., Gianaroli, L., Fortini, D., Conte, N., Magli, C., Cavani, S., (2000) Frequency of hyper-hypohaploidy and diploidy in ejaculate, epididymal and testicular germ cells of infertile patients. Hum Reprod 15:2165–2172.
- Blanco, J., Egozcue, J. and Vidal, F. (1996) Incidence of chromosome 21 disomy in human spermatozoa as determined by fluorescent in-situ hybridization. Hum Reprod 11:722–726.
- Bonduelle, M., Van, A.E., Joris, H., Keymolen, K., Devroey, P., Van Steirteghem, A. and Liebaers, I. (2002) Prenatal testing in ICSI pregnancies: incidence of chromosomal anomalies in 1586 karyotypes and relation to sperm parameters. Hum Reprod 7:2600–2614.
- Burrello, N., Calogero, A.E., De Palma, A., Grazioso, C., Torrisi, C., Barone, N., (2002) Chromosome analysis of epididymal and testicular spermatozoa in patients with azoospermia. Eur J Hum Genet 10:362–366.
- Carrell, D.T. (2008a) Contributions of spermatozoa to embryogenesis: assays to evaluate their genetic and epigenetic fitness. Reprod Biomed Online 16:474–484.
- Carrell, D.T. (2008b) The clinical implementation of sperm chromosome aneuploidy testing: pitfalls and promises. J Androl 29:124–133.
- Carrell, D.T., Wilcox, A.L., Lowy, L., Peterson, C.M., Jones, K.P., Erickson, L., (2003) Elevated sperm chromosome aneuploidy and apoptosis in patients with unexplained recurrent pregnancy loss. Obstet Gynecol 101:1229–1235.
- Colombero, L.T., Hariprashad, J.J., Tsai, M.C., Rosenwaks, Z. and Palermo, G.D. (1999) Incidence of sperm aneuploidy in relation to semen characteristics and assisted reproductive outcome. Fertil Steril 72:90–96.
- Dakouane, M., Bicchieray, L., Bergere, M., Albert, M., Vialard, F. and Selva, J. (2005) A histomorphometric and cytogenetic study of testis from men 29-102 years old. Fertil Steril 83:923–928.
- Dobles, M., Liberal, V., Scott, M.L., Benezra, R. and Sorger, P.K. (2000) Chromosome missegregation and apoptosis in mice lacking the mitotic checkpoint protein Mad2. Cell 101:635–645.
- Dul, E.C., van Ravenswaaij-Arts, C.M., Groen, H., van Echten-Arends, J. and Land, J.A. (2010) Who should be screened for chromosomal abnormalities before ICSI treatment? Hum Reprod 25:2673–2677.
- Egozcue, J., Blanco, J., Anton, E., Egozcue, S., Sarrate, Z. and Vidal, F. (2003) Genetic analysis of sperm and implications of severe male infertility-a review. Placenta 24:S62–65.
- Emery, B.R. and Carrell, D.T. (2006) The effect of epigenetic sperm abnormalities on early embryogenesis. Asian J Androl 8:131–142.
- Evenson, D. and Wixon, R. (2006) Meta-analysis of sperm DNA fragmentation using the sperm chromatin structure assay. Reprod Biomed Online 12:466–472.
- Evenson, D.P., Larson, K.L. and Jost, L.K. (2002) Sperm chromatin structure assay: its clinical use for detecting sperm DNA fragmentation in male infertility and comparisons with other techniques. J Androl 23:25–43.
- Gianaroli, L., Magli, M.C., Cavallini, G., Crippa, A., Nadalini, M., Bernardini, L., (2005) Frequency of aneuploidy in sperm from patients with extremely severe male factor infertility. Hum Reprod 20:2140–2152.
- Gonsalves, J., Sun, F., Schlegel, P.N., Turek, P.J., Hopps, C.V., Greene, C., (2004) Defective recombination in infertile men. Hum Mol Genet 13:2875–2883.
- Greco, E., Iacobelli, M., Rienzi, L., Ubaldi, F., Ferrero, S. and Tesarik, J. (2005a) Reduction of the incidence of sperm DNA fragmentation by oral antioxidant treatment. J Androl 26:349–53.
- Greco, E., Scarselli, F., Iacobelli, M., Rienzi, L., Ubaldi, F., Ferrero, S., (2005b) Efficient treatment of infertility due to sperm DNA damage by ICSI with testicular spermatozoa. Hum Reprod 20:226–230.
- Hassold, T.J., Sherman, S.L., Pettay, D., Page, D.C. and Jacobs, P.A. (1991) XY chromosome nondisjunction in man is associated with diminished recombination in the pseudoautosomal region. Am J Hum Genet 49:253–260.
- Huang, W.J., Lamb, D.J., Kim, E.D., de Lara, J., Lin, W.W., Lipshultz, L.I. and Bischoff, F.Z. (1999) Germ-cell nondisjunction in testes biopsies of men with idiopathic infertility. Am J Hum Genet 64:1638–1645.
- Levron, J., Aviram-Goldring, A., Madgar, I., Raviv, G., Barkai, G. and Dor, J. (2001) Sperm chromosome abnormalities in men with severe male factor infertility who are undergoing in vitro fertilization with intracytoplasmic sperm injection. Fertil Steril 76:479–484.
- Martin, R.H. (2006) Meiotic chromosome abnormalities in human spermatogenesis. Reprod Toxicol 22:142–147.
- Martin, R.H., Greene, C., Rademaker, A.W., Ko, E. and Chernos, J. (2003) Analysis of aneuploidy in spermatozoa from testicular biopsies from men with nonobstructive azoospermia. J Androl 24:100–103.
- Mateizel, I., Verheyen, G., Van Assche, E., Tournaye, H., Liebaers, I. and Van Steirteghem, A. (2002) FISH analysis of chromosome X, Y and 18 abnormalities in testicular sperm from azoospermic patients. Hum Reprod 17:2249–2257.
- Moskovtsev, S.I. (2008) Management of patients with high sperm DNA damage. Indian J Med Res 127:101–103.
- Moskovtsev, S.I., Jarvi, K., Mullen, J.B., Cadesky, K.I., Hannam, T. and Lo, K.C. (2010) Testicular spermatozoa have significantly lower DNA damage compared to ejaculated spermatozoa in patients with unsuccessful oral antioxidant treatment. Fertil Steril 93:1142–1146.
- Muriel, L., Goyanes, V., Segrelles, E., Gosálvez, J., Alvarez, J.G. and Fernandez, J.L. (2007) Increased aneuploidy rate in sperm with fragmented DNA as determined by the sperm chromatin dispersion (SCD) test and FISH analysis. J Androl 28:38–49.
- Nicopoullos, J.D., Gilling-Smith, C., Almeida, P.A., Homa, S., Nice, L., Tempest, H. and Ramsay, J.W. (2008) The role of sperm aneuploidy as a predictor of the success of intracytoplasmic sperm injection? Hum Reprod 23:240–50.
- Nicopoullos, J.D.M., Ramsay, J.W., Almeida, P.A. and Gilling-Smith, C. (2004) Assisted reproduction in the azoospermic couple. BJOG 111:1190–1203.
- Palermo, G.D., Colombero, L.T., Hariprashad, J.J., Schlegel, P.N. and Rosenwaks, Z. (2002) Chromosome analysis of epididymal and testicular sperm in azoospermic patients undergoing ICSI. Hum Reprod 17:570–575.
- Pellestor, F., Andréo, B., Taneja, K. and Williams, B. (2003) PNA on human sperm: a new approach for in situ aneuploidy estimation. Eur J Hum Genet 11:337–41.
- Rodrigo, L., Rubio, C., Mateu, E., Simón, C., Remohí, J., Pellicer, A. and Gil-Salom, M. (2004) Analysis of chromosomal abnormalities in testicular and epididymal spermatozoa from azoospermic ICSI patients by fluorescence in-situ hybridization. Hum Reprod 19:118–123.
- Rodrigo, L., Rubio, C., Peinado, V., Villamon, R., Al-Asmar, N., Remohi, J., (2011) Testicular sperm from patients with obstructive and nonobstructive azoospermia: aneuploidy risk and reproductive prognosis using testicular sperm from fertile donors as control samples. Fertil Steril 95:1005–1012.
- Schlegel, P.N. (1999) Testicular sperm extraction: microdissection improves sperm yield with minimal tissue excision. Hum Reprod 4:131–135.
- Shahine, L.K. and Cedars, M.I. (2006) Preimplantation genetic diagnosis does not increase pregnancy rates in patients at risk for aneuploidy. Fertil Steril 85:51–56.
- Silber, S., Escudero, T., Lenahan, K., Abdelhadi, I., Kilani, Z. and Munné, S. (2003) Chromosomal abnormalities in embryos derived from testicular sperm extraction. Fertil Steril 79:30–38.
- Sinha Hikim, A.P. and Swerdloff, R.S. (1999). Hormonal and genetic control of germ cell apoptosis in the testis. Rev Reprod 4:38–47.
- Spano, M., Bonde, J.P., Hjollund, H.I., Kolstad, H.A., Cordelli, E. and Leter, G. (2000) Sperm chromatin damage impairs human fertility. The Danish First Pregnancy Planner Study Team. Fertil Steril 73:43–50.
- Tempest, H.G., Abogrein, A., Cheng, S.Y., Gillott, D.J., Handyside, A.H., Thornhill, A.R. and Griffin, D.K. (2009) Should we routinely test for sperm aneuploidy prior to ICSI? Hum. Reprod 24( suppl 1):13–i14.
- Tesarik, J., Ubaldi, F., Rienz,i L., Martinez, F., Iacobelli, M., Mendoza, C. and Greco, E. (2004) Caspase-dependent and -independent DNA fragmentation in Sertoli and germ cells from men with primary testicular failure: relationship with histological diagnosis. Hum Reprod 19:254–61.
- The ESHRE Capri Workshop Group (2007) Intracytoplasmic sperm injection (ICSI) in 2006: Evidence and Evolution. Hum Reprod 13:515–526.
- Topping, D., Brown, P., Judis, L., Schwartz, S., Seftel, A., Thomas, A. and Hassold, T. (2006) Synaptic defects at meiosis I and non-obstructive azoospermia. Hum Reprod 21:3171–3177.
- Vernaeve, V., Tournaye, H., Osmanagaoglu, K., Verheyen, G., Van Steirteghem, A. and Devroey, P. (2003) Intracytoplasmic sperm injection with testicular spermatozoa is less successful in men with nonobstructive azoospermia than in men with obstructive azoospermia. Fertil Steril 79:529–533.
- Verpoest, W. and Tournaye, H. (2006) ICSI: hype or hazard? Hum Fertil 9:81–92.
- Viville, S., Warter, S., Meyer, J.M., Wittemer, C., Loriot. M., Mollard. R. and Jacqmin, D. (2000) Histological and genetic analysis and risk assessment for chromosomal aberration after ICSI for patients presenting with CBAVD. Hum Reprod 15:1613–1618.
- World Health Organization (1999) WHO laboratory manual for the examination of human semen and sperm-cervical mucus interaction, 4th ed. Cambridge University Press, Cambridge, UK.