Abstract
Among the different techniques available to evaluate blastocyst quality, the most frequently used are those that allow the counting of the number of cells of the two distinct cell lineages present at this stage (trophectoderm or TE and inner cell mass or ICM), through differential staining. The goal of this study was to compare three different methods for the differential staining of mouse blastocysts: a TE selective labelling method using a lectin, a TE permeabilization method based on the use of a detergent, and immunodetection of TE and ICM specific markers. Mouse blastocysts produced by parthenogenetic activation were used to determine and compare the efficiency and the cell counts of each method. The results showed that the TE permeabilization and immunodetection methods were superior, providing equivalent TE, ICM, and total cell counts.
Introduction
The routine application of techniques for the in vitro production and manipulation of mammalian embryos worldwide has led to a demand for methods that can easily evaluate embryo quality to allow embryologists and researchers to select the protocols yielding the best quality embryos. At present, this evaluation is mainly performed through the study of the developmental rates and morphology of embryos cultured until the blastocyst stage and through the analysis of the quality of the blastocysts obtained.
Non-invasive techniques for the evaluation of blastocyst quality are preferred in clinical and agricultural settings to select embryos for transfer. In comparison, invasive techniques, that require lysis or fixation of the blastocysts, are primarily used in research studies as they can provide accurate information [Van Soom et al. Citation2001]. Among these, differential staining techniques, that permit differentiation of the two blastocyst cell populations, TE and ICM [Marikawa and Alarcon Citation2009], are preferred.
The first report of a differential staining method was based on the permeability barrier created by TE [Solter and Knowles Citation1975]. TE cells form an outer epithelial monolayer that seals the embryo and protects the ICM, an aggregate of cells attached to the basal surface of TE, from complement-mediated cell lysis when embryos are incubated with a species-specific antiserum targeted to cell surface antigens [Handyside and Hunter Citation1984]. In this way, only TE cells are stained with a membrane-impenetrable DNA stain, such as propidium iodide (PI), whereas both permeabilized TE cells and intact ICM cells are stained with a membrane-penetrable DNA stain, such as Hoechst. Identification of the two cell populations resolves as the blue ICM cell nuclei (stained only with Hoechst) that can be clearly distinguished from the pink TE cell nuclei (stained both with PI and Hoechst). When antibodies are not commercially available, this method requires the production of an antiserum against cell surface markers, that is usually limited to a single species, and the success of this technique reflects the thermolability of the complement solution.
Several modifications of the original protocol have been developed to circumvent these limitations while retaining differential staining of TE nuclei compared with a label that also recognizes ICM nuclei. Initially, the use of trinitrobenzenesulphonic acid to label cell surface proteins with trinitrophenyl (TNP) groups and of a commercially available universal antiserum that cross-reacts with all TNP-labelled proteins was described [Hardy et al. Citation1989]. Although this technique can be applied to different species, it still requires complement-mediated lysis. Next an antibody-free method that uses a calcium ionophore as a membrane-permeabilizing agent has been described [de la Fuente and King Citation1997]. Since TE permeabilization is not selective, overexposure to the ionophore can lead to ICM permeabilization and staining. Subsequently, TE permeabilization using a detergent has been described [Thouas et al. Citation2001]. It is a cost-effective, faster, and simpler technique that can be applied to many species, but has the same disadvantages as previously described [de la Fuente and King Citation1997].
The use of a commercially available fuorescein-5-isothio-cyanate (FITC)-labeled wheat germ agglutinin (WGA) to stain TE cells has also been described [De La Fuente and King Citation1998]. In this method there is no selective permeabilization of TE cells, but differential staining of TE and ICM cells is also based on their position. Thus, all nuclei are stained with the same dye (PI) but only the outer cells of the blastocyst (TE cells) can be are visualized by FITC-labeled WGA staining.
Recently, immunofluorescence detection of the TE specific transcription factor Cdx2 [Beck et al. Citation1995] and the ICM specific transcription factor Oct4 (Pou5f1) [Palmieri et al. Citation1994] has been described as a new differential staining method [Van Thuan et al. Citation2006]. In this technique, the position of the cells is not relevant to achieve a differential staining and, for the first time, a specific staining of both TE and ICM cells is performed.
Independent evaluation of three approaches was undertaken to test the efficacy of each towards achieving differential mouse blastocyst TE/ICM staining and the cell counts obtained. Blastocysts obtained 96 hours after parthenogenetic activation were used because synchronic activation ensured uniformity. The TE permeabilization method, based on the use of a detergent [Thouas et al. Citation2001], the TE selective labelling method, based on the use of a fluorescence-conjugated lectin [De La Fuente and King Citation1998], and the immunodetection method, based on the use of TE and ICM antibody specific markers [Van Thuan et al. Citation2006] were then compared. The results presented below should provide a basis to select the most appropriate differential staining method depending on the characteristics of the study.
Results and Discussion
A total of 53 blastocysts at 96 hours post-activation were processed by the TE selective labeling method (). Although it was possible to guess the location of ICM cells, as the blastocyst region with lower intensity green fluorescence and more densely packed red nuclei, differentiation of the two blastocyst cell types was not clear enough to allow the differential cell counting in any of the images obtained. Several optimization strategies were attempted but were not successful. These included changing the fixative solution and incubation time, and even using a cell-permeable nuclear stain to avoid the permeabilization step. In contrast, both the TE permeabilization and immunodetection methods proved useful for the differential staining of mouse blastocysts. As shown in and , clear images were obtained with sufficient resolution to permit the direct counting of individual TE and ICM cells. Using the TE permeabilization method, ICM nuclei were stained blue whereas the nuclei of permeabilized TE cells were stained both blue and red, turning pink in the merged images (). In the immunodetection method, TE nuclei were stained green and nuclei of ICM cells were stained red, due to the combination of secondary antibodies used ().
Figure 1. Blastocyst stained using the trophectoderm (TE) selective labeling method. A1) TE cells labeled with Alexa Fluor 488-conjugated wheat germ agglutinin (WGA). Arrowhead shows inner cell mass (ICM) location, where green fluorescence intensity is lower. A2) TE and ICM nuclei staining with propidium iodide. A3) merged A1 and A2 images.
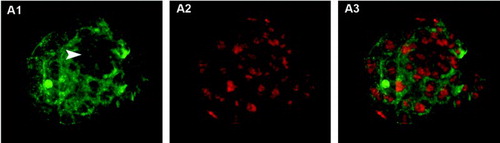
Figure 2. Blastocysts stained using trophectoderm (TE) permeabilization. Column 1 (A1, B1, C1) shows all blastocyst nuclei stained with Hoechst 33258 (blue). Column 2 (A2, B2, C2) shows TE nuclei stained with propidium iodide (PI, red). Column 3 (A3, B3, C3) shows merged images where TE nuclei are pink and inner cell mass (ICM) nuclei are blue. A1-A3) correctly stained blastocyst. Arrowhead points to the condensed chromatin of the second polar body and arrow indicates a dividing TE cell. B1-B3) incorrectly stained blastocyst showing poor PI staining, probably due to insufficient permeabilization of TE cells. C1-C3) incorrectly stained blastocyst showing PI staining in all nuclei, probably due to excessive cell permeabilization.
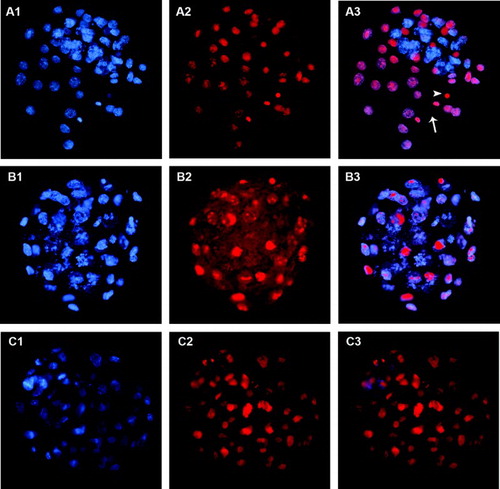
Figure 3. Blastocyst stained using the immunodetection method. A1) Hoechst 33258 staining of all nuclei. A2) Cdx2 staining of trophectoderm (TE cells). A3) Oct4 staining of inner cell mass (ICM cells). A4) merged A2 and A3 images. White arrowheads indicate four dividing TE cells where Cdx2 spreads over the whole cytoplasm. The yellow arrowhead indicates a nucleus coexpressing Cdx2 and Oct4.
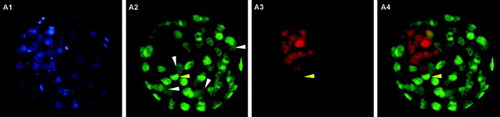
The efficiencies of the TE permeabilization and immunodetection methods were high and varied from 87.9 to 100%. As summarized in , significant statistical differences were noted between them (p < 0,0001). All of the blastocysts processed by immunodetection were well suited for differential cell counting, whereas some of the blastocysts processed for the TE permeabilization method had to be discarded due to a poor red staining (B) that prevented clear identification and thus obscured counting of individual TE cells, or to non-specific staining of all blastocyst cells (C). In this method, differential staining is based simply on the position of the two cell populations in the blastocyst (the outer TE cells are permeabilized and the inner ICM cells are not) and depends on an incubation of only 12 seconds. Thus, it is not unexpected that even very small variations of incubation time could yield different degrees of TE permeabilization affecting the quality of differential staining. Inefficient staining of approximately 20% of mouse and bovine blastocysts has been previously reported [Thouas et al., Citation2001], in accord with the data (10%-26.3%) presented in .
Table 1. Efficiencies of the TE permeabilization and immunodetection methods for the differential staining of mouse blastocysts.
Both staining methods provided equivalent results for TE, ICM, and total cell number in almost all replicates and in the pooled data (). Only two replicates showed significant differences in the number of ICM cells between the two methods. However, taking into account the sample size of each replicate, these differences could probably be attributed to differences in ICM cell number between the embryos processed in each method. This assumption is supported by the fact that no preferences towards higher or lower numbers of ICM cells were observed after using the TE permeabilization or immunodetection method.
Table 2. Blastocyst cell counts for the TE permeabilization and immunodetection methods.
The correlation between the outcomes of the TE permeabilization method, based on cell position, and the immunodetection method, based on transcription factor expression, can be related to the cellular and molecular mechanisms leading to the establishment of TE and ICM lineages during mouse embryonic development. The external or internal location of blastomeres at the 32-cell stage in relation to the developing embryo defines their commitment towards the TE or ICM lineage, respectively. At the blastocyst stage, external cells form a monolayer of epithelial cells that surrounds the embryo and constitutes the TE, whereas internal cells form an aggregate attached to the basal surface of the TE and constitute the ICM [Marikawa and Alarcon Citation2009]. Internal and external cells do not only differ morphologically but also molecularly, as lineage-specific transcription factors are differentially expressed in these two cell populations. For example, Cdx2 [Beck et al. Citation1995] and Oct4 (Pou5f1) [Palmieri et al. Citation1994] are specifically located in the nucleus of TE and ICM cells, respectively. Their interactions during blastocyst formation are thought to reinforce TE and ICM fates [Marikawa and Alarcon Citation2009; Yamanaka et al. Citation2006]. Because TE cells are tightly connected through junctional complexes, they create a tightly sealed layer which protects the internal ICM cells from the detergent used in the TE permeabilization method and, as a result, only TE cells, positive for Cdx2 in the immunodetection method, are stained by both PI and Hoechst. In contrast, non-permeabilized ICM cells, positive for Oct4 in the immunodetection method, can only be stained by Hoechst in the TE permeabilization method.
It should be noted that coexpression of Cdx2 and Oct4 markers was detected in 1 to 9 cells per blastocyst in 46% of the blastocyts processed by the immunodetection method, where the nuclei were stained both green and red (). Coexpression has also been described by Wang et al. [2010]. It has been reported that Oct4 is initially expressed in all cells of the 4/8-cell stage embryo, but upon TE differentiation Cdx2 expression becomes stronger in the external blastomeres. Cdx2 gradually represses the expression of Oct4 in the TE until its disappearance [Palmieri et al. Citation1994; Yamanaka et al. Citation2006]. Thus, the cells that coexpress Cdx2 and Oct4 are likely TE-committed cells with residual Oct4 expression, and for this reason were considered as TE cells when counting cells.
Fixed blastocysts processed by the immunodetection method can be stored in blocking solution at 4°C for at least 6 months before staining. This can be advantageous because it permits one to accumulate embryos from several replicated experiments to be processed later. For the same reason, distinction and/or counting of TE/ICM cells can be easily combined with other analyses. These could include other important embryo quality markers like the apoptotic cell ratio through the staining of active caspase-3 [Wydooghe et al. Citation2011], or the expression of other transcription factors, such as Brg1 [Wang et al. Citation2010], in studies of cell-fate regulation. In comparison, the TE permeabilization method is rapid and less-expensive. Key to the successful staining in the TE permeabilization method is quickly moving the embryos in and out of the permeabilization solution. However, embryos cannot be accumulated and must be stained immediately.
The TE permeabilization and immunodetection methods are highly efficient and repeatable for the differential staining of mouse blastocysts, yielding equivalent counts for TE, ICM, and total blastocyst cells. The TE permeabilization method may prove better suited for routine analysis of blastocyst cell numbers in those cases where blastocysts are produced in large numbers because, although it is not the most efficient, it is rapid, simple, and inexpensive. In comparison the immunodetection method may prove optimal in those cases where limited and valuable blastocysts have to be analyzed because, although being more expensive and requiring more time, it is extremely efficient and ensures positive results in all embryos processed.
Materials and Methods
Unless otherwise indicated, all reagents were purchased from Sigma (Spain).
Collection of mouse oocytes and parthenogenetic activation
Animal care and procedures were conducted according to the protocols approved by the Ethics Committee on Animal and Human Research of the Universitat Autònoma de Barcelona and by the Departament de medi Ambient i Habitatge of the Generalitat de Catalunya. Hybrid B6CBAF1 (C57Bl/6xCBA/J) female mice (age 7 to 12 w) were used as oocyte donors. Females were induced to superovulate by intraperitoneal injection of 5 IU of pregnant mare serum gonadotropin (PMSG; Intervet, Spain) followed 48h later by 5 IU of human chorionic gonadotropin (hCG; Farma-Lepori, Spain). Mature oocytes were collected from the oviducts 14 to 15 h after hCG administration in Hepes-buffered Potassium Simplex Optimized Medium (KSOMH) [Biggers et al. Citation2000] and treated with 300 U/ml hyaluronidase at 37°C until dispersion of cumulus cells. Denuded oocytes were then washed and kept in drops of Potassium Simplex Optimized Medium (KSOM) (MR-106-D, Millipore, Spain) [Biggers et al. Citation2000] covered with mineral oil at 37°C under 5% CO2 for 4h before their activation. Parthenogenetic activation was performed by incubation of the oocytes for 6h (37°C, 5% CO2) in 10 mM SrCl2 in Ca2+free-CZB medium [Chatot et al. Citation1989] supplemented with 5 µg/ml cytochalasin B to prevent second polar body extrusion. After activation, oocytes showing two visible pronuclei were cultured in KSOM until the blastocyst stage.
Blastocyst differential staining and cell counts
Embryos that reached the blastocyst stage 96h post-activation, which were more or less equally expanded because of the simultaneous parthenogenetic activation, were used for the differential staining of TE and ICM cells, using three previously described methods as explained below. In each experiment, blastocysts were divided into two to three groups in order to perform the different staining methods in parallel.
In the TE selective labeling method, based on the use of a lectin, the protocol described by De La Fuente and King [1998] was used. First, the zona pellucida was removed with 0.5% pronase in protein-free KSOMH, and then zona-free blastocysts were exposed to 100 µg/ml Alexa Fluor 488-labeled WGA (W11261; Molecular Probes, Inc., USA) in PBS for 20 min at 39°C. After that, blastocysts were incubated in 100% ethanol for 15 min for a first fixation, followed by a permeabilization in PBS containing 0.25% Tween 20, 0.4% bovine serum albumin (BSA) and 5 µg/ml PI for 8 min, all at room temperature. Finally, they were rinsed for 20 to 30 s in PBS before overnight fixation in 100% ethanol at 4°C. Embryos were then washed in a drop of glycerol and mounted onto a glass microscope slide in a microdrop of glycerol.
In the TE permeabilization method, based on the use of a detergent, the protocol described by Thouas et al. [2001] was followed. Blastocysts were first incubated for 12 s in a permeabilization solution consisting of 1% Triton X-100 and 100 µg/ml PI in KSOMH. They were then quickly transferred to a fixative solution of 100% ethanol with 25 µg/ml Hoechst 33258 (H1398, Molecular Probes, Inc.) and stored at 4°C overnight. Finally, embryos were washed in a drop of glycerol and mounted onto a glass microscope slide in a microdrop of glycerol.
The immunodetection method, based on the detection of TE and ICM specific markers, was adapted from Van Thuan et al. [2006]. Blastocysts were fixed for 15 min in 4% paraformaldehyde and incubated overnight at 4°C in a blocking solution containing 0.5% Triton X-100, 3% goat serum, and 0.2% sodium azide in a PBS solution. Incubation with a 1:100 dilution of a mouse monoclonal anti-Cdx2 primary antibody (MU392A-UC; BioGenex, USA) was also performed overnight at 4°C, and then embyos were incubated with 6 µg/ml chicken anti-mouse Alexa Fluor 488 secondary antibody (A21200; Molecular Probes, Inc.) for 2 h at room temperature. To label ICM cells, embryos were next incubated in a 1:50 dilution of rabbit polyclonal anti-Oct4 antibody (sc9081; SantaCruz Biotechnology, Inc., Germany) for 1.5 h at room temperature and then with 6 µg/ml goat anti-rabbit Alexa Fluor 594 secondary antibody (A11037; Molecular Probes, Inc.) for a subsequent 30 min at room temperature. Samples were then washed in blocking solution after incubation with either primary or secondary antibodies. Finally, embryos were mounted onto a glass microscope slide in a microdrop of Vectashield Mounting Medium (H-1000; Vector Laboratories, Inc., USA) containing 10 µg/ml Hoechst 33258 as a nuclear counterstain.
In all cases, a little pressure was exerted on the mounted samples in order to flatten the blastocysts to obtain a 2D projection of the blastocyst's cells to facilitate cell counting without the use of a confocal microscope. Samples were examined with an Olympus Bx41 (Olympus, Spain) epifluorescence microscope fitted with specific filters and an image capture and analyzing system (Isis software version 5.4.5, Metasystems, USA). ImageJ software (Image J 1.45q, Wayne Rasband, National Institutes of Health, USA) was used for cell counting.
Statistical analysis
Experiments were repeated six times on separate days. The efficiency of the staining methods was calculated considering the number of processed blastocysts in which TE and ICM cells were properly stained and cell counts could be performed. Data were analyzed using Fisher exact's test for efficiencies and Mann-Witney test for mean cell number comparisons using the GraphPad InStatTM program (version 3.05 for Windows 95, GraphPad Software, USA). Values with a p < 0.05 were considered statistically significant.
Abbreviations
BSA: | = | bovine serum albumin |
DNP: | = | Dinitrophenol |
FITC: | = | fuorescein-5-isothiocyanate |
hCG: | = | human chorionic gonadotropin |
ICM: | = | inner cell mass |
KSOM: | = | potassium simplex optimized medium |
KSOMH: | = | Hepes-buffered KSOM |
PI: | = | propidium iodide |
PMSG: | = | pregnant mare serum gonadotropin |
TE: | = | trophectoderm |
TNP: | = | trinitrophenol |
WGA: | = | wheat germ agglutinin. |
Acknowledgments
We thank Jonatan Lucas for his technical assistance and the staff at the Servei d'Estabulari of the Universitat Autònoma de Barcelona for taking care of the mice.
Declaration of interest: This work was supported by the Spanish Ministerio de Ciencia e Innovación (AGL 2011-23784) and the Generalitat de Catalunya (2009 SGR 282). AM is a predoctoral fellow of the Universitat Autònoma de Barcelona. The authors report no conflicts of interest.
Author contributions: Conceived the study and designed the experiments: EI; Performed the experiments: AM; Analyzed the data: AM, EI, JS; Wrote the manuscript: EI, AM; All authors revised the manuscript and approved the final version.
References
- Beck, F., Erler, T., Russell, A. and James, R. (1995) Expression of cdx-2 in the mouse embryo and placenta: Possible role in patterning of the extra-embryonic membranes. Dev Dyn 204:219–227.
- Biggers, J.D., McGinnis, L.K. and Raffin, M. (2000) Amino acids and preimplantation development of the mouse in protein-free potassium simplex optimized medium. Biol Reprod 63:281–293.
- Chatot, C.L., Ziomek, C.A., Bavister, B.D., Lewis, J.L. and Torres, I. (1989) An improved culture medium supports development of random-bred 1-cell mouse embryos in vitro. J Reprod Fertil 86:679–688.
- de la Fuente, R. and King, W.A. (1997) Use of a chemically defined system for the direct comparison of inner cell mass and trophectoderm distribution in murine, porcine and bovine embryos. Zygote 5:309–320.
- De La Fuente, R. and King, W.A. (1998) Developmental consequences of karyokinesis without cytokinesis during the first mitotic cell cycle of bovine parthenotes. Biol Reprod 58:952–962.
- Handyside, A.H. and Hunter, S. (1984) A rapid procedure for visualising the inner cell mass and trophectoderm nuclei of mouse blastocysts in situ using polynucleotide-specific fluorochromes. J Exp Zool 231:429–434.
- Hardy, K., Handyside, A.H., and Winston, R.M. (1989) The human blastocyst: Cell number, death and allocation during late preimplantation development in vitro. Development 107:597–604.
- Marikawa, Y. and Alarcon, V.B. (2009) Establishment of trophectoderm and inner cell mass lineages in the mouse embryo. Mol Reprod Dev 76:1019–1032.
- Palmieri, S.L., Peter, W., Hess, H. and Scholer, H.R. (1994) Oct-4 transcription factor is differentially expressed in the mouse embryo during establishment of the first two extraembryonic cell lineages involved in implantation. Dev Biol 166:259–267.
- Solter, D. and Knowles, B.B. (1975) Immunosurgery of mouse blastocyst. Proc Nat Acad Sci 72:5099–5102.
- Thouas, G.A., Korfiatis, N.A., French, A.J., Jones, G.M. and Trounson, A.O. (2001) Simplified technique for differential staining of inner cell mass and trophectoderm cells of mouse and bovine blastocysts. Reprod Biomed Online 3:25–29.
- Van Soom, A., Vanroose, G. and de Kruif, A. (2001) Blastocyst evaluation by means of differential staining: A practical approach. Reprod Domest Anim 36:29–35.
- Van Thuan, N., Wakayama, S., Kishigami, S., Ohta, H., Hikichi, T., Mizutani, E., (2006) Injection of somatic cell cytoplasm into oocytes before intracytoplasmic sperm injection impairs full-term development and increases placental weight in mice. Biol Reprod 74:865–873.
- Wang, K., Sengupta, S., Magnani, L., Wilson, C.A., Henry, R.W. and Knott, J.G. (2010) Brg1 is required for Cdx2-mediated repression of Oct4 expression in mouse blastocysts. PloS One 5:e10622.
- Wydooghe, E., Vandaele, L., Beek, J., Favoreel, H., Heindryckx, B., De Sutter, P., (2011) Differential apoptotic staining of mammalian blastocysts based on double immunofluorescent CDX2 and active caspase-3 staining. Anal Biochem 416:228–230.
- Yamanaka, Y., Ralston, A., Stephenson, R.O. and Rossant, J. (2006) Cell and molecular regulation of the mouse blastocyst. Dev Dyn 235:2301–2314.