Abstract
The aim of this study is to prepare nonwoven fabrics carrying silver nanoparticles (AgNPs), and to investigate their antibacterial activities and cytotoxicities in parallel. AgNPs were impregnated from their nanoemulsions onto two commercially available nonwoven fabrics: pure-cotton fabrics (PCF) and polyester/viscous fabrics (PVF), by a simple adsorption (dipping) and were then heat stabilized. PCF exhibited stronger antibacterial effects on both Staphylococcus aureus and Escherichia coli. In-vitro cell culture studies demonstrated that AgNPs nanoemulsions and also fabrics carrying them were cytotoxic on L929-fibroblasts in all concentrations used here (6.25–400 ppm) in different extends. Only the fabrics loaded with AgNPs using nanoemulsion with the lowest concentration of 6.25 ppm exhibited low cytotoxicity but were still antibacterial.
Introduction
The emergence of bacterial resistance to antibiotics following widespread clinical and veterinary usage has made antibiotics much less effective means that we are now facing the threat of these very dangerous/powerful enemies – pathogenic bacteria – resistant to most or all available antibiotics (Livermore Citation2004). Thus, exploring alternative approaches to develop antibacterial agents is an urgent task gaining tremendous attraction as expected/needed.
Silver has been in use since ancient times in the forms of metallic silver, silver nitrate, silver sulfadiazine, etc., initially to make water potable initially and then many years for the treatment of burns, wounds and several bacterial infections (Klasen Citation2000, Landsdown Citation2002, Castellano et al. Citation2007, Rai et al. Citation2009). They have been replaced by antibiotics in time, however recently it appears that the interest has returned back to silver – especially in nanoparticle form mainly due to its high surface area to volume ratio and also other unique chemical and physical properties (Morones et al. Citation2005, Gong et al. Citation2007, Kim et al. Citation2007, Ales et al. Citation2009, Rai et al. Citation2009, Chaloupka et al. Citation2010).
Silver ions are highly reactive on almost all kinds of bacteria, there are different scenarios about their action: it has been mostly agreed that they can bind to proteins both on the bacterial wall and in the cytoplasm and cause denaturation which brings macro structural changes leading to cell distortion and death. They can also bind to bacterial ribosomes, genomic DNA, RNAs and several proteins which inhibit bacterial replication (Landsdown Citation2002, Castellano et al. Citation2007). Silver nanoparticles (AgNPs) may also interact with proteins on bacterial wall and enter the bacterial cell in their free forms (nanoemulsions). They preferably attack the respiratory chain, cell division finally leading to cell death. However, most likely silver ions released from their surfaces determine their bactericidal activity when they are immobilized or entrapped on/in carrier matrices such as fabrics, gels, etc. (Feng et al. Citation2000, Sondi and Salopek-Sondi Citation2007, Morones et al. Citation2005, Song et al. Citation2006, Shrivastava et al. Citation2007).
Antibacterial properties of both AgNPs and their immobilized forms on a series of bacteria (mainly E.coli and S.aureus, but also others) have been investigated by several groups (Richard et al. Citation2002, Morones et al. Citation2005, Panacek et al. Citation2006, Durán et al. Citation2007, Gong et al. Citation2007, Jun et al. Citation2007, Kim et al. Citation2007, Lee et al. Citation2007, Pal et al. Citation2007, Perelshtein et al. Citation2008, Ruparelia et al. Citation2008, Guzman et al. Citation2008, Shahroukh et al. 2009, Ales et al. Citation2009, Venesa et al., Citation2009, Chaloupka et al. Citation2010, Ravindra et al. Citation2010, Petrus et al. Citation2011, El-Shishtawy et al. Citation2011, Xu et al. Citation2011, El-Rafiea et al. Citation2012, Osório et al. Citation2012, Pivec et al. Citation2012). There are similar but also controversial reports, which are understandable because both materials and experimental (test) conditions are quite different. For instance, type of bacteria and/or their species; inoculation densities of bacteria (relative to the amount of AgNPs used); AgNPs type, size/shape, the material properties carrying them; incubation period, temperature, pH and ionic strength are all different, therefore most of the time these results are not even comparable.
One of the most important points in these studies – worth to note – is the design of these studies which have mostly attempted to demonstrate the antibacterial performances of their systems – both the AgNPs and the materials carrying them. Silver in almost all forms is toxic on mammalian cells. The cytotoxicity on humans, animals, and of course on environments is a critical issue. This was the main rationale to design the study presented here. We have used commercially available AgNPs and immobilized (impregnated) them onto two different nonwoven fabrics, and have investigated both their antibacterial performances and cytotoxicities on mammalian cells in culture by following rather well-defined/classical techniques in both areas, in parallel and presented here.
Materials and methods
Preparation of antibacterial fabrics
Two different types of commercially available nonwoven fabrics, a pure (100%) cotton fabrics (PCF) and a polyester/viscous fabrics 50/50 mixed material (PVF), were used and obtained from Nikoo group (Tehran, Iran). AgNPs were purchased from Nanocolloid (PRChina) which were in a size range of 3–18 nm (reported by the company).
Several impregnation approaches have been investigated to load these AgNPs onto the nonwoven fabrics in the initial studies (Moghtader Citation2012). The most simple and successful approach was “dipping” in other terms “adsorption from aqueous nanoemulsions”, therefore the results obtained only with this technique were reported here. In a typical procedure, AgNPs nanoemulsions containing different amounts of nanoparticles (in the range of 6.25–400 ppm) were prepared by dilution of the stoke solution with a concentration of 4000 ppm. Nonwoven fabrics were cut into disk-shaped samples with a diameter of 6mm and then dipped into these nanoemulsions which were then shaken in an incubator at a constant temperature of 37°C for 24 h. The fabrics were taken from the media, rinsed/washed with distilled water several times and then autoclaved for dry-sterilization – which was applied also for thermal stabilization – of the adsorbed AgNPs – for stronger attachment to the polymer matrix – at 120°C for 30 min.
Antibacterial tests
Bacterial suspensions containing Staphylococcus aureus as the model gram-positive and Escherichia coli as the model gram-negative bacteria were used after proper dilutions from the stoke suspensions. They were first used as suspensions to obtain the antibacterial power of the AgNPs that we have used in this study. The test tubes containing the same amount of the target bacteria (about 1.5 × 108) in Mueller Hinton Bras and varying amounts of AgNPs were incubated at 37°C for 24 h. The tubes were examined with naked eyes and the concentration of the first test tube showing the change from opaque to transparent (the lowest concentration causing bacterial death) was obtained, which was defined as “minimum inhibition concentration” (MIC). In the parallel tests, 100 μL suspensións – taken from each tube – were transfered to bacterial dishes containing Muller Hinton Agar layers (the thicknesses were about 4–5 mm) which were then incubated at 37°C for 24 h. The dishes were examined with naked eyes and the concentration of the first test dish showing no bacterial growth (the lowest concentration causing bacterial death) was selected, which was defined as “minimum bacterial concentration” (MBC).
In order to obtain antibacterial activities of the AgNPs-loaded fabrics, a conventional “disk diffusion assay” was applied in which the target bacteria (about 1.5 × 108) were placed evenly onto the Muller Hinton Agar layers in the bacterial dishes. Then fabrics carrying different amounts of AgNPs were placed onto the top of the agar layers. One (in the central part) or more disks were placed in each plate. Disks carrying no AgNPs were also used as positive control in these tests. They were then incubated in an oven at 37°C for 24 h. The anti-biogram disk diameters were measured and reported as a measure of antibacterial activity. A minimum of three parallel tests were performed for each type of nonwoven fabrics, and the average and standard deviations were demonstrated.
Cytotoxicity tests
Cytotoxicities of both the AgNPs nanoemulsions and AgNPs-loaded fabrics were investigated in three parallel tests. The first one was the so-called “cell proliferation assay” in which a real-time analyzer (SP instrument, Roche, Germany) was used to monitor cellular responses after inoculation with both nanoparticles and fabrics. L929 fibroblast cell line was used and 10 × 103 cells were put in each well of the e-plate 96 (Roche, Germany) containing DMEM-F12 without L-glutamine but with 10% FCS and 1% antibiotic (penicillin–streptomycin, Serva, Israel). The plates were kept in a CO2 incubator (at 37°C in 5% CO2) for 19 h. Then different amounts of AgNPs (6.25–400 ppm) and fabrics loaded with different amounts of AgNPs were placed in the wells. The control wells were similar but contain no material. Note that minimum three wells were used for each type of material for statistical analysis. Cell attachment, proliferation and growth were recorded at every 10 min over 48 h automatically. The control wells were similar but contain no material.
In the second test group, L929 fibroblast cells were put in each well of the classical 96-well plates (10 × 103 cells per well) containing DMEM-F12 with L-glutamine this time and with 10% FCS and 1% antibiotic (penicillin–streptomycin, Serva, Israel). The plates were kept in a CO2 incubator (at 37°C in 5% CO2) for 24 h. The cell culture media were replaced with the fresh ones on the cells attached to the bottom of the wells. Then different amounts of AgNPs (6.25–400 ppm) and dressings loaded with different amounts of AgNPs were placed in the wells. The control wells were similar but contain no material. Note that minimum three wells were used for each type of material for statistical analysis. After incubation for another 48 h, 15 μL water-soluble WST-1(2-(4-iodophenyl)-3(4-nitrophenyl)-5-(2,4-disulfophenyl)-2h-tetrazolium) (Roche, Germany) was added into each well, and incubated for another 4 h. The plates were read in a standard ELISA Microplate Reader (Biotek, Gene 5 Power Wave XS2, USA) at 440 nm and 630 nm.
In the third group, in order to observe necrotic cells the following protocol was applied: L929 fibroblast cells were put in each well of the classical 48-well plates (10 × 103 cells per well) containing DMEM-F12 with L-glutamine, 10% FCS and 1% antibiotic (penicillin–streptomycin, Serva, Israel). The plates were kept in a CO2 incubator (at 37°C in 5% CO2) for 24 h. Then, different amounts of AgNPs (6.25–400 ppm) and fabrics loaded with different amounts of AgNPs were placed in the wells and incubated at the same condition for another 24 h. The attached cells and the suspension were collected separately, washed with FCS (Biological industries, USA) and stained by treating with a double staining solution containing 2 μg/ml Hoechst dye (33342, Sigma-Aldrich, Germany), 2 μg/mL propidium iodide (Sigma-Aldrich, Germany), and 100 μg/mL DNAse free-RNAse for 15 min at room temperature. Then, 10–50 μL of the cell suspension smeared onto a glass slide for examination of fluorescence microscope (Lieca DMI6000, Germany). The nuclei of normal cells were stained blue fluorescence of low intensity with the Hoechst dye. The nuclei of necrotic cells were stained red with propidium iodide which could cross the wall of the necrotic cells lacking the plasma membrane integrity while not penetrating through the non-necrotic cell wall. The numbers of necrotic cells were obtained by counting 10 randomly chosen microscopic field using a 20x objective with a Fluorescence inverted microscope (Leica DMI6000, Germany) with FITC filters. The data were expressed as the ratio of necrotic cells to normal cells.
Results and discussion
Fabrics loaded with AgNPs
The SEM micrographs of the AgNPs show that they are quite monosized but the average sizes are above 30nm (much larger than what is reported by the company: 3–18nm) (). They look like agglomerates in this picture but this was due to the drying procedure done prior to SEM studies.
Representative optical pictures of the nonwoven fabrics and SEM micrographs of fibers are shown in . Macroscopically, both nonwoven fabrics look similar; however, PCF exhibit somewhat flat fiber structure with rectangular cross-sections and have quite rough surfaces, while PVF have circular cross sections and rather smooth/shiny surfaces. gives representative SEM micrographs of the fibers after AgNPs adsorption. It was interesting to observe that AgNPs were rather evenly adsorbed/distributed on the PCF while they were somewhat accumulated at different parts of the PVF surfaces. This difference may be explained not only with the differences of surface chemistries (both AgNPs and fabrics) but also – most likely – with the differences on relative surface roughness and shape of these fibers (see also ). It should be noted that both fabrics were washed/rinsed with distilled water several times to ensure the removal of weakly attached (not heat stabilized) AgNPs from the surfaces before use.
Antibacterial properties
In the first group of experiments, antibacterial properties of AgNPs were investigated both in suspensions and in bacterial dishes using both S.aureus and E.coli. The MIC and MBC values were about the same for both bacteria and were around 3 and 6 ppm. Note that the same dilutions were applied for both bacteria, these were the concentrations in those specific tubes, which were high-enough values (strong antibacterial effects). There are quite a number of studies related to antibacterial effects of AgNPs on different bacteria in which some of the MIC and MBC values reported are higher and some of them are lower than our findings (Morones et al. Citation2005, Panacek et al. Citation2006, Kim et al. Citation2007, Guzman et al. Citation2008, Rai et al. Citation2009, Petrus et al. Citation2011). Some have reported similar values for different bacteria, some of them have reported lower/higher values for different species. They were almost in the same concentration range for the strains of both S.aureus and E.coli that we have used in this study. These are somewhat expected results because types of both AgNPs and bacteria are different, and the experimental conditions are different.
Representative optical photographs in give the results obtained in a typical “disk diffusion assay” in which antibacterial properties of the fabrics carrying AgNPs were tested with two different bacteria S.aureus and E.coli. Note that the white disk at the center is the nonwoven fabric. The darker layer surrounding the fabric disk – the so-called “anti-biogram disk” – shows the dead cell region which is formed due to antibacterial effect of the AgNPs on the fabrics. As seen in these plates, the anti-biogram disk diameter decreases when the amount of AgNPs loaded onto the dressing disks is decreased (from 1 to 5). The Plate #6 contains only the nonwoven cotton fabric (no AgNPs loading); therefore, no bacterial loss was observed as expected.
Figure 4. Representative optical photographs showing the results obtained in a “disk diffusion assay”. (A) for S. aureus and (B) for E. coli. The plates numbered from 1 to 5 contain nonwoven cotton-based fabrics loaded with different amounts of AgNPs. The Plate #6 carries only the PCF fabric without any AgNPs loading.
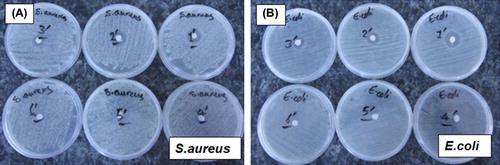
gives the anti-biogram data obtained in the “disk diffusion assays” in which two different fabrics – PCF and PVF – containing different amounts of AgNPs and two different bacteria, S.aureus and E.coli. Each value represents the average of mínimum three parallel sets of study and the standard deviations. In the control experiments in which only fabrics (without any AgNPs loading) were used, no antibacterial activity was observed. There were still bacteria underneath the fabrics (attached to the surface of both dishes and fabrics) when we removed the fabrics from the surface carefully, after incubation. The antibacterial effect decreased with the decrease in AgNPs loading as expected. The fabrics loaded with the lowest AgNPs concentration of 6.25 ppm also exhibited quite notable antibacterial effects. Both fabrics were more effective on E.coli which is expected and similar to most of the findings reported in the literature (Feng et al. Citation2000, Panacek et al. Citation2006, Castellano et al. Citation2007, Lee et al. Citation2007, Rai et al. Citation2009, Shahrokh and Emtiazi Citation2009, Xu et al. Citation2011, El-Rafiea et al. Citation2012). Note that gram-positive bacteria (e.g., S.aureus) have more peptidoglycan than gram-negative bacteria (e.g., E.coli) because of their thicker cell walls, and because peptidoglycan is negatively charged and silver ions are positively charged, more silver may get trapped by peptidoglycan layers (not entered into the cytoplasm) in gram-positive bacteria than in gram-negative bacteria. Therefore, higher antibacterial effects are usually observed on gram-negative bacteria as we have also seen in our studies demonstrated here.
Table I. Anti-biogram data obtained in the “disk diffusion assays” for PCF and PVF containing different amounts of AgNPs and for two different bacteria: S. aureus and E.coli.
The PCF exhibited higher antibacterial effects than those observed with the PVF. Most probably due to surface properties of cotton fibers, we were able to adsorb more AgNPs and these nanoparticles attached more strongly to their rough surfaces at the heat stabilization step (see also ). On the contrary, it was not easy to adsorb AgNPs on the smoother/shiny surfaces of PVF, and they were most probably washed away during the cleaning step. As a result more pronounced antibacterial effects were observed for the PCF compared to those observed for the PVF.
Cytotoxicities
In the first group of cell culture tests (“cell proliferation assay”), L929 fibroblast cells were incubated with both AgNPs nanoemulsions with different concentrations and fabrics carrying different amounts of AgNPs using a real-time analyzer as mentioned before. illustrates the changes in the cell index indicating proliferation rate of L929 fibroblast cells, which were recorded every 10 min for 48 h. Note that the cells were first incubated and then the materials were put in each well about the 19th hour.
Figure 5. Real-time monitoring of interaction of L929 fibroblast cells in culture with: (A) AgNPs nanoemulsion with different concentrations; (B) PCF loaded with AgNPs; and (C) PVF loaded with AgNPs. The numbers on the graphs represent: (0) only medium or fabric (no AgNPs); and (I)–(VII) AgNPs concentrations: 6.25, 12.5, 25, 50, 100, 200 and 400 ppm, respectively.
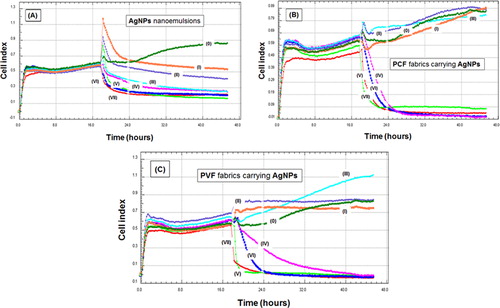
shows the responses to AgNPs nanoemulsion with different concentrations. Note that the peaks at 19th hour are only electrical noises due to sudden loading to the wells – not related to cell responses. There was an increase in the wells in which there was no material – only the medium; therefore, there was a steady increase in the cell counts. However, the anti-proliferative effects of AgNPs were obvious; they were relatively low with the lowest concentration of 6.25 ppm but increased significantly with the increase in the nanoparticle concentration.
There are similarities in the responses of fibroblasts to two different fabrics impregnated with AgNPs using the same nanoemulsion concentrations, PCF and PVF, , respectively. The fabrics loaded with AgNPs emulsions with concentrations of 25 ppm and lower do not cause any significant anti-proliferation effects on fibroblasts; especially in the case of PCF, cell proliferations were almost the same as the control wells. Both PCF and PVF loaded using AgNPs nanoemulsions with higher concentrations were highly cytotoxic and caused a rapid loss of cells in the wells. This behavior was more pronounced in the case of PCF loaded with the same concentrations which is parallel to the antibacterial effects observed for both types of fabrics. Most probably the amounts of AgNPs adsorbed onto PCF surfaces were higher and more stable – not washed out during the cleaning processes.
In the second test group, L929 fibroblast cells were first incubated with AgNPs emulsions (6.25–400 ppm) and fabrics carrying them in 96-well first at 37°C in 5% CO2 for 24 h, and another 4 h after addition of tetrazolium salts, and then viable cell percentages which were analyzed with an ELISA Reader. As seen in , almost all materials caused cell death, which was much significant in the cases of free AgNPs in the nanoemulsions, and increased with the increase in their concentration. PCF were less cytotoxic than PVF, especially at the lower AgNPs loadings. It should be noted that fabrics impregnated with a concentration of 25 ppm and even lower have also caused significant loss in the cell counts (viabilities). It was not similar to that observed in the first group of tests discussed above. This was considered as a safe concentration according to the cell proliferation test results (). Note that in those tests we have followed the cell proliferation for about 48 h. There were cell deaths in the medium, but also cells were growing which overcame the cell number decrease due to toxicity. This second group of tests seems more reliable (gives direct cell death because of cytotoxicity) and is rather classical to exhibit cell toxicity of the materials. Only the fabrics loaded with nanoparticles using nanoemulsions with an AgNP concentration of 6.25 ppm exhibited low cytotoxicity (assumed safe), but they were antibacterial enough.
Figure 6. Fibroblast cell viability after incubation with: (A) AgNPs nanoemulsions with different concentrations (6.25–200 ppm); (B) PCF loaded with AgNPs; and (C) PVF loaded with AgNPs. “0” shows the case where only medium or fabrics (no AgNPs) were used.
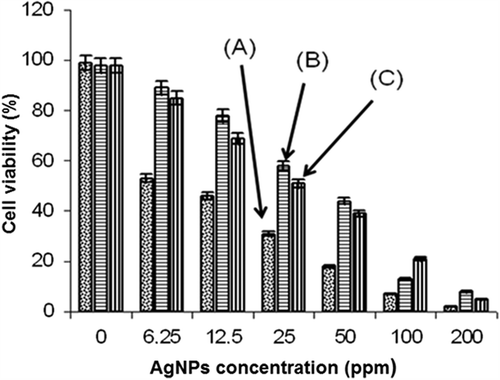
In the third test group, L929 fibroblast cells were incubated with AgNPs nanoemulsions and fabrics impregnated with them in the classical 48-well plates, and the apoptotic and necrotic cells were counted after staining with two fluorescent dyes (i.e., Hoechst and propidium iodide) using a fluorescence microscopy. shows typical micrographs which demonstrate both healthy (blue stained with the Hoechst dye) and necrotic (red stained with the propidium iodide – indicated also with arrows) cells. The percentages of the necrotic cells observed after exposing the fibroblasts to AgNPs nanoemulsions or fabrics carrying AgNPs are given in . As seen here there was severe necrosis due to cytotoxicities of the materials used, especially with high concentrations of AgNPs, as observed also in the second group of cytotoxicity tests discussed above. Much less necrotic cells – in the acceptable levels – were observed in the AgNPs on the fabrics, especially with the ones prepared with 6.25 ppm.
Figure 7. Representative fluorescence microscopy images showing the necrotic L929 cells after interaction with: (A) only medium or the plain fabrics (no AgNPs); (B) AgNPs nanoemulsions; (C) PCF loaded with AgNPs; and (D) PVF loaded with AgNPs. The healthy cells look all blue (stained with the Hoechst 33342 dye) – means no necrotic effect. The red color (stained with propidium iodate) demonstrates the necrotic cells (indicated also with arrows). Magnifications are 200x and the scale bar is 40 μ.
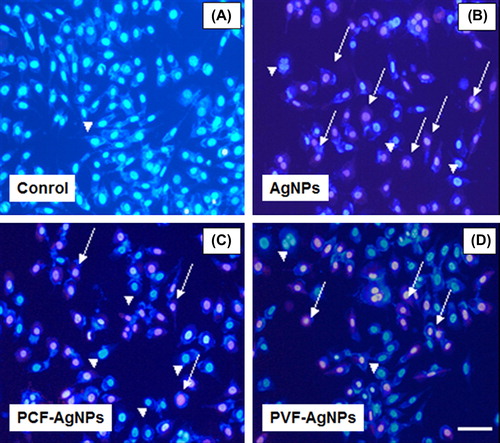
Table II. Necrotic cell percentages obtained with AgNPs and PCF and PVF containing different amounts of AgNPs.
Conclusions
Our results demonstrated that AgNPs in free form are powerful antibacterial agents but also highly toxic, even in the adsorbed form on the fabrics. As the main conclusion, we could say that only the fabrics loaded from nanoemulsions with 6.25 ppm AgNPs concentrations could be assumed safe, and also luckily antibacterial enough.
One of the most important issues for impregnation of AgNPs onto fabrics, especially at production scale, is the process itself. We have tested several methods for impregnation of AgNPs onto the fabrics in our preliminary studies (Moghtader Citation2012), and found that “dipping” is the simplest and effective method, which is easily adaptable (scale up) to industrial processes – in nonwoven textile production lines – without incurring high expenditure and operation costs.
Stabilization is another important issue. AgNPs release during use is certainly not desirable as it may cause high toxicity and also environmental contaminations. Heat stabilization after AgNPs adsorption is an easy and effective approach that we have applied here. The type of AgNPs (size, shape and surface chemistries), physical form, surface chemistry and structure of fabrics may be equally important as indicated in this study.
As mentioned above, quite a number of studies have been accumulated in the literature about bactericidal AgNPs formulations/materials; there are also comparisons about the effectiveness of different systems. However, we believe that it is not correct to do that because AgNPs are synthesized and used very differently, and the preparation of materials loaded with AgNPs and formulations and structures of the final products (such as creams, dressings, etc.) are very different: bacteria and their species are not usually described well and different; and the test methods and conditions are different. This means that the performance data are not comparable. Only the results of different materials could be compared as we did in this study. Standardization of – at least – performance test methods is strongly needed before the market gets filled with these successful antibacterial materials carrying AgNPs but with a high risk of cytotoxicity as demonstrated in this study, and environmental contaminations reported by others (Faunce and Watal Citation2010).
Acknowledgements
Prof. Erhan Pişkin is supported by Turkish Academy of Sciences as a full member. We would like to thank both Prof. Hadi Zareie, Izmir-Institute of High Technology, Izmir, for personally taking the SEM micrographs, and Anadolu University, Eskisehir, for allowing us to use their SEM facilities.
Declaration of interest
The authors report no declarations of interest. The authors alone are responsible for the content and writing of the paper.
References
- Ales P, Milan K, Renata V, Robert P, Jana S, Vladimir K. 2009. Antifungal activity of silver nanoparticles against Candida Spp. Biomaterials. 30:6333–6340.
- Castellano JJ, Shafii SM, Ko F, Donate G, Wright TE, Mannari RJ, et al. 2007. Comparative evaluation of silver-containing antimicrobial fabrics and drugs. Int Wound J. 4:114–122.
- Chaloupka K, Malam Y, Seifalian AM. 2010. Nanosilver as a new generation of nanoproduct in biomedical applications. Trends Biotechnol. 28: 580–588.
- Durán N, Marcato PD, De Souza GIH, Alves OL, Esposito E. 2007. Antibacterial effect of silver nanoparticles produced by fungal process on textile fabrics and their effluent treatment. J Biomed Nanotechnol. 3:203–208.
- El-Rafiea MH, Shaheena TI, Mohamed AA, Hebeish A. 2012. Bio-synthesis and applications of silver nanoparticles onto cotton fabrics. Carbohydrate Polym, 90: 915–920.
- El-Shishtawy RM, Asiri AM, Abdelwahed AAM, Al-Otaibi MM. 2011. In situ production of silver nanoparticle on cotton fabric and its antimicrobial evaluation. Cellulose. 18:75–82.
- Faunce T, Watal A. 2010. Nanosilver and global public health: international regulatory issues. Nanomedicine. 5:617–632.
- Feng QL, Wu J, Chen GQ, Cui FZ, Kim TN, Kim JO. 2000. A mechanistic study of the antibacterial effect of silver ions on Escherichia coli and Staphylococcus aureus. J Biomed Mater. 52:662–668.
- Gong P, Li H, He X, Wang K, Hu J, Tan W, et al. 2007. Preparation and antibacterial activity of Fe3o4@Ag nanoparticles. Nanotechnology. 18:604–11.
- Guzman MG, Dille J, Godet S. 2008. Synthesis of silver nanoparticles by chemical reduction method and their antibacterial activity. World Acad Sci Eng Technol. 43:357–364.
- Jun J, Yuan-Yuan D, Shao-hai W, Shao-feng Z, Zhong-yi W. 2007. Preparation and characterization of antibacterial silver-containing nanofibers for wound dressing applications. J US-China Med Sci. 4:52–54.
- Kim JS, Kuk E, Yu KN, Kim JH, Park SJ, Lee HJ. 2007. Antimicrobial effects of silver nanoparticles. Nanomed Nanotechnol Biol Med. 3:95–101.
- Klasen HJ. 2000. A historical review of the use of silver in the treatment of burns. Part I earlyuses. Burns. 30:1–9.
- Landsdown ABG. 2002. Silver and its antibacterial properties and mechanism of action. J Wound Care. 11:125–38.
- Lee HY, Park HK, Lee YM, Kim K, Park SB. 2007. A practical procedure for producing silver nanocoated fabric and its antibacterial evaluation for biomedical applications. Chem Commun. 35:2959–2961.
- Livermore DH. 2004. The need for new antibiotics. Clin Microbiol Infec. 10:1–9.
- Moghtader F. 2012. Production of nanowoven dressing materials loaded with silver nanoparticles and investigation of their antibacterial properties, M.Sc. Thesis, Hacettepe University, Institute of Graduate Studies, Nanotechnology and Nanomedicine Division, 2012 (Abstract in English is available).
- Morones JR, Elechiguerra JL, Camacho A, Ramirez JT. 2005. The bactericidal effect of silver nanoparticles. Nanotechnology. 16:2346–2353.
- Osório I, Igreja R, Franco R, Cortez J. 2012. Incorporation of silver nanoparticles on textile materials by an aqueous procedure. Mater Lett. 75:200–203.
- Pal S, Tak K, Myong SJ. 2007. Does the antibacterial activity of silver nanoparticles depend on the shape of the nanoparticles?A study of the gram-negative bacterium Escherichia Coli. Appl Environ Microbiol. 73:1712–1720.
- Panacek A, Kvitek L, Prucek R, Kolar M, Vecerova R, Pizurova N. 2006. Silver colloid nanoparticles: synthesis, characterization, and their antibacterial activity. J Phys Chem. 110:16248–16253.
- Perelshtein I, Applerot G, Perkas N, Guibert G, Mikhailov S, Gedanken A. 2008. Sonochemical coating of silvernanoparticles on textile fabrics (nylon, polyester and cotton) and their antibacterial activity. Nanotechnol. 19:77–83.
- Petrus E.M, Tinakumar S, Chai LC, Ubong A, Tunung R, Elexson N, et al. 2011. A study on the minimum inhibitory concentration and minimum bactericidal concentration of nano colloidal silver on food-borne pathogens. Intl Food Res J. 18:55–66.
- Pivec T, Persin Z, Hribernik S, Maver T, Kolar M, Stana-Kleinschek K. 2012. Binding silver nano-particles onto viscose Non-woven using different commercial Sol-gel procedures Mtaec9.46:75–80.
- Rai M, Yadav A, Gade A. 2009. Silver nanoparticles as a new generation of antimicrobials. Biotechnol Adv. 27:76183.
- Ravindra S, Mohan YM, Reddy NN, Raju KM. 2010. Fabrication of antibacterial cotton fibres loaded with silver nanoparticles via “Green Approach”. Coll Surf A Physicochem Eng Aspects. 367: 31–40.
- Richard JW, Spencer BA, Mccoy LF, Carina E, Washington J, Edgar P. 2002. Acticoat versus silverlon: the truth. J Burns Surg Wound Care. 1:111–120.
- Ruparelia JP, Chattejee Ak, Duttagupta Sp, Mukherji S. 2008. Strain specifity In antimicrobial activity of silver and copper nanoparticels. Acta Biomaterialia. 4:707–716.
- Shahrokh S, Emtiazi G. 2009. Toxicity and unusual biological behavior of nanosilver on gram positive and negative bacteria assayed by microtiter-plate. Eur J Biol Sci. 1:28–31.
- Shrivastava S, Bera T, Roy A, Singh G, Ramachandrarao P, Dash D. 2007. Characterization of enhanced antibacterial effects of novel silver nanoparticles. Nanotechnology. 18:103–12.
- Sondi I, Salopek-Sondi B. 2007. Silver nanoparticles as antimicrobial agent: a case study on E.coli as a model for gram-negative bacteria. J Colloid Interface. 275:177–182.
- Song HY, Ko KK, Oh LH, Lee BT. 2006. Fabrication of silver nanoparticles and their antimicrobial mechanisms. Eur Cells Mater. 11:58–64.
- Venesa N, Ayala N, Humberto H. 2009. Silver nanoparticles toxicity and bacterial effect against methicillin-resistant Staphylococcus aureus. Nanobiotechnol. 5:2–9.
- Xu H, Shi X, Ma H, Lv Y, Zhang L, Mao Z. 2011. The preparation and antibacterial effects of dopa-cotton/AgNPs. Appl Surf Sci. 257:6799–