Abstract
The biosynthesis of nanoparticles has received attention because of the development of economic and environmentally friendly technology for the synthesis of nanoparticles. The study develops a convenient method for the green synthesis of silver and gold nanoparticles by utilizing fresh root extract of the four-year old Panax ginseng plant, and evaluated the antimicrobial applications of silver nanoparticles against pathogenic microorganisms. P. ginseng is a well-known herbal medicinal plant, and its active ingredients are mainly ginsenosides. The fresh root of the 4 year old P. ginseng plant has been explored for the synthesis of silver and gold nanoparticles without the use of any additional reducing and capping agents. The reduction of silver nitrate led to the formation of silver nanoparticles within 2 h of reaction at 80°C. The gold nanoparticles were also successfully synthesized by the reduction of auric acid at 80°C, within 5 min of reaction. The biosynthesized gold and silver nanoparticles were characterized by techniques using various instruments, viz. ultraviolet-visible spectroscopy (UV-Vis spectroscopy), field emission transmission electron microscopy (FE-TEM), energy dispersive X-ray analysis (EDX), elemental mapping, and X-ray diffraction (XRD). In addition, the silver nanoparticles have shown antimicrobial potential against Bacillus anthracis, Vibrio parahaemolyticus, Staphylococcus aureus, Escherichia coli, and Bacillus cereus.
Introduction
Nanotechnology has developed as a modern field of research with potential effects in the electrical, chemical, and biological fields. Metal nanoparticles are considered important, owing to their unique particle size and shape-dependent physiochemical and biological properties (CitationSathishkumar et al. 2009). Currently, there is a growing need to develop the eco-friendly techniques for nanoparticle synthesis which avoid the use of hazardous chemicals and solvents, in order to evade the adverse effects and to increase the application of nanoparticles in the medical and environmental platforms. The exploitation of various biological species for the biosynthesis, including microorganisms and plant materials, does not involve any hazardous chemicals, solvents or energy resources, and is thus considered an eco-friendly technology. In addition, the biological methodologies are advantageous over chemical and physical methodologies, as they are economical, ecofriendly, and avoid any physical or chemical barriers. Several plants have been studied for the synthesis of nanoparticles, and the rate of synthesis of nanoparticles by living resources is comparable to the rates obtained using chemical or physical methods. In addition, when compared to microorganisms as well, the biological synthesis by plants is quite fast (CitationElavazhagan and Arunachalam 2011, CitationMubarakAli et al. 2011, CitationArunachalam and Annamalai 2013). In continuing the same approach for the synthesis of nanoparticles by green technology, we develop here a rapid and economical biological method for the synthesis of silver and gold nanoparticles using fresh root extract of Panax ginseng.
P. ginseng is a slow-growing perennial herb. For a very long time, the plant has been cultivated and used as a traditional medicine in Korea, China and Japan (CitationRadad et al. 2006). P. ginseng is mostly found in Korea, northeastern China, and far-eastern Siberia (CitationWen and Zimmer 1996, CitationHu 1976). The major active components of the plant are ginsenosides. More than 40 ginsenosides have been reported for their pharmacological activity. The pharmacological effects of ginsenosides involve their anti-cancer, anti-diabetic, immunomodulatory, anti-inflammatory, radioprotective, antioxidant, anti-apoptotic anti-amnestic, and anti-stress properties (CitationKim et al. 2014). Moreover, research has proposed that some of the active components of P. ginseng also exert beneficial effects on disorders of the central nervous system, aging, and neurodegenerative diseases (CitationRadad et al. 2006). In addition, the ginsenoside polymer conjugates was reported for better efficacies (CitationMathiyalagan et al. 2014). The root of the P. ginseng plant has been the main component used for medicinal purposes, due to its ginsenoside composition (CitationShi et al. 2007, CitationKim et al. 2015). Thus, in our study, we focused on the fresh root of the P. ginseng plant for the biosynthesis of silver and gold nanoparticles.
Silver and gold nanoparticles are presently under intensive study due to their attractive physiochemical and biological properties, and their potential applications in the development of new technologies for applications with a medical background, like optoelectronic devices, biological sensors, and drug delivery systems, and also as catalysts (CitationMajdalawieh et al. 2014). For a long time, silver has been used for the treatment of medical ailments due to its antimicrobial properties, to treat many microbial infections (CitationDar et al. 2013, CitationKhan et al. 2013). The use of silver in nanoparticle form, as compared to its ionic form, appears to have reduced cellular toxicity and enhanced antibacterial efficacy, due to the formation of free radicals. Silver nanoparticles have been found to have direct antimicrobial and anti-inflammatory effects, and are mostly applied in the medical industry, to prevent infection in burns and open wounds (CitationDar et al. 2013, CitationGarg et al. 2014). Gold nanoparticles are well known for their unique and tunable physiochemical properties and their biomedical applications (CitationKhalil et al. 2012, CitationMubarakAli et al. 2011).
Owing to the wide-ranging applications of silver and gold nanoparticles in diverse areas of science and technology, different methods have been proposed for their synthesis. In our study, P. ginseng root has been explored for the biological synthesis of silver and gold nanoparticles. The synthesized silver and gold nanoparticles were characterized using ultraviolet-visible spectroscopy (UV-Vis), field emission transmission electron microscopy (FE-TEM), energy dispersive X-ray analysis (EDX), elemental mapping, and X-ray diffraction (XRD). The synthesized nanoparticles have been explored for their biological activity as antimicrobial agents. Previously, red ginseng has been studied for the biosynthesis of gold nanoparticles (CitationLeonard et al. 2011). P. ginseng (dried powder) has also been reported for the green synthesis of silver nanoparticles in an ecofriendly manner (CitationVimalanathan et al. 2013). However, there has been no report for the utilization of fresh root extract of four-year old fresh P. ginseng for the biological synthesis of silver and gold nanoparticles. Thus, the present study demonstrates the potential of fresh root extract of P. ginseng for the ability to synthesize silver and gold nanoparticles in an ecofriendly and facile manner.
Materials and methods
Materials
Silver nitrate (AgNO3) and Gold (III) chloride trihydrate (HAuCl4·3H2O) were purchased from Sigma-Aldrich Chemicals, USA. All the media were purchased from Difco, MB cells, Seoul, Republic of Korea. Fresh P. ginseng roots were collected from the four-year old P. ginseng plants from Gochang, Republic of Korea. The pathogenic bacterial strains used were Bacillus anthracis [NCTC 10340], Vibrio parahaemolyticus [ATCC 33844], Staphylococcus aureus [ATCC 6538], Escherichia coli [ATCC 10798], and Bacillus cereus [ATCC 14579]. The bacterial strains were cultured in nutrient agar media at 37°C and preserved at − 70°C in glycerol stock vials for further study. All antibiotics used were purchased from Oxoid Ltd., England: vancomycin (VA30) 30 μg/disk, rifampicin (RD5) 5 μg/disk, oleandomycin (OL15) 15 μg/disk, penicillin G (P10) 10 μg/disk, novobiocin (NV30) 30 μg/disk, and lincomycin (MY15) 15 μg/disk.
Preparation of root extract
P. ginseng root (25 g) was cleaned and washed with double-distilled water several times to remove any dust or suspended particles. Further, the roots were cut into small pieces and ground using a mortar and pestle. Then, the ground mixture of ginseng root was boiled for 30 min in 100 ml of sterile water, so that root components leached out into the water. The collected extract was then filtered using Whatman filter paper, and was centrifuged at 10 000 rpm for 10 min, to remove any suspended material. The total volume of filtrate was maintained at 100 ml with sterile water at the end of the process, and stored at 4°C for further use. This 100 ml of stock filtrate was used further for the synthesis of silver and gold nanoparticles.
Synthesis of silver and gold nanoparticles
For the synthesis of silver nanoparticles, 5 ml from the 100 ml of ginseng root stock filtrate was mixed with 25 ml of sterile water. Then, the silver nitrate was added, with a final concentration of 1 mM in the reaction mixture. The reaction mixture was then kept at 80°C for 2 h. The color change was observed continuously, which indicated the formation of silver nanoparticles in the reaction mixture. For the collection and purification of silver nanoparticles, the reaction mixture was first centrifuged at 2000 rpm for 10 min, to remove any other unwanted components. Then, the silver nanoparticles were obtained by centrifugation at 16,000 rpm for 20 min, and the particles were further purified by repeated centrifugation followed by dispersion of the pellet in sterile water thrice, to remove the water-soluble biomolecules such as proteins and secondary metabolites (CitationGarg et al. 2014). This product was used for further characterization and confirmation of silver nanoparticle synthesis. Similarly, the methodology was repeated for gold nanoparticle synthesis using Gold (III) chloride trihydrate, at 0.1 mM final concentration at 80°C for 5 min. After 5 min, the products were collected and purified for further characterization. The silver and gold nanoparticles were collected by centrifugation and several times of washing with sterile water, and were finally kept for overnight air-drying and obtained in powder form. This product obtained was used for the characterization of the synthesized nanoparticles.
Characterization of silver and gold nanoparticles
To verify the reduction of silver and gold ions, the respective reaction mixture was scanned in the range of 300–800 nm in a UV-Vis spectrophotometer (2100 Pro, Amersham, Biosciences Corp. USA). The size, shape, morphology and distribution of the nanoparticles were analyzed using Ultrospec (FE-TEM), EDX, and elemental mapping with a JEM-2100F (JEOL) instrument operated at 200 kV. The sample was prepared by placing a drop of collected nanoparticles on a carbon-coated copper grid, and subsequently dried in an oven at 60°C, before being transferred to the microscope. The XRD analyses were performed on an X-ray diffractometer, D8 Advance, (Bruker), Germany, operated at 40 kV, 40 mA, with CuKα radiation, at a scanning rate of 6°/min, and a step size of 0.02, over the 2θ range of 20–80°.
The stability of nanoparticles was observed before and after the addition of sodium hydroxide (NaOH) to the nanoparticle solution. The effect of the pH change on the stability of the nanoparticles was studied, in the pH range of 3–12.
Analysis of antimicrobial activity of silver and gold nanoparticles
The antimicrobial activity of nanoparticles against pathogenic microorganisms such as B. anthracis, V. parahaemolyticus, S. enterica, S. aureus, E. coli, and B. cereus was measured on Muller-Hinton agar (MHA) plates using the disc diffusion method. In this assay, the MHA medium plates were spread evenly with 100 μL of an overnight log-culture of test organisms, and each standard antibiotic disc of vancomycin, rifampicin, oleandomycin, penicillin, novobiocin, and lincomycin was maintained as control. The sterile paper disc was further impregnated with 50 μL (100 mg/L) of freshly prepared and partially purified silver and gold nanoparticle solutions, and was placed onto the agar plates. Then, the plates were incubated at 37°C for 24 h. After the incubation period, the zones of inhibition around silver and gold nanoparticles were measured, and compared with the zone of inhibition of each antibiotic disc.
Results and discussion
Many plants and their extracts have been reported for the synthesis of nanoparticles and their biomedical applications (CitationElavazhagan and Arunachalam 2011, CitationMubarakAli et al. 2011, CitationArunachalam and Annamalai 2013). To our knowledge, this is first report for the utilization of fresh P. ginseng root extract for the biosynthesis of silver and gold nanoparticles. The synthesis of nanoparticles was monitored by the change in color of the reaction mixture. The reduction of silver ions was completed within 2 h of reaction at 80°C, and the reaction mixture completely turned from being white to being brown in color, corresponding to the surface plasmon vibrations of silver nanoparticles which resemble the synthesis of silver nanoparticles in the reaction mixture (CitationGarg et al. 2014). On the other hand, no color change was observed in a control that contained the same ratio of root extract with sterile water which was kept under similar conditions. Similarly, for gold nanoparticles, the reaction mixture completely turned pink after a 5-min incubation period at 80°C, whereas no color change was observed in the control. The biological synthesis of silver and gold nanoparticles using root extract of Zingiber officinale (CitationVelmurugan et al. 2014) and Trianthema decandra (Aizoaceae) (CitationGeethalakshmi and Sarada 2012) have been reported earlier. The root extract of P. ginseng contains many heterocyclic compounds, including saponin, which play a major role in the synthesis of silver and gold nanoparticles (CitationGeethalakshmi and Sarada 2012). The presence of different ginsenosides and polysaccharides in the P. ginseng root must have influenced the formation of nanoparticles. Previously, gold nanoparticles were successfully synthesized using red ginseng. The study suggested that various phytochemicals within the plant effectively reduce silver salts to produce silver nanoparticles, and that the saponin glycosides provide additional stability by providing a coating on the silver nanoparticles (CitationLeonard et al. 2011). In addition, silver nanoparticle synthesis has been carried out using the dried root of P. ginseng in an ecofriendly manner (CitationVimalanathan et al. 2013). However, this is the first report for the facile synthesis of silver and gold nanoparticles using four-year old fresh P. ginseng root extract.
The reduction of silver and gold ions after exposure to the P. ginseng root extract was followed by UV-Vis spectroscopy. The UV-Vis spectrophotometric analysis of the final reaction mixture showed maximum absorption peaks at 412 nm and 534 nm, which are the characteristic peaks for silver () and gold nanoparticles (), respectively. Studies indicate that the absorbance in this range corresponds to the formation of silver and gold nanoparticles in the reaction mixture (CitationGarg et al. 2014, CitationGeethalakshmi and Sarada 2012). When the particles were stored at 20°C, the UV-Vis spectra of the nanoparticle solutions remained unchanged over several months, indicating that the nanoparticle size distribution was stable in water.
Figure 1. UV-Vis spectra of the reaction mixture containing silver nanoparticles (a) and gold nanoparticles (b), respectively.
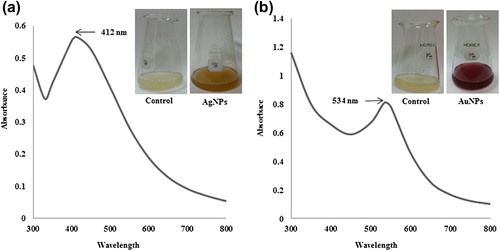
In previously reported studies of the biological synthesis of silver and gold nanoparticles by bacteria (CitationDas et al. 2014) and fungi (CitationBirla et al. 2013), the time required for synthesis ranged from one to several days. This is one of the limitations of the biosynthetic procedure that must be addressed if the methodology is to be compared with physical and chemical methods. The decrease in reaction time from some days to a few hours and minutes was observed when using the fresh root extract of P. ginseng. This represents a major and significant step toward achieving the goal of ecofriendly, cheap, and rapid synthesis of silver and gold nanoparticles.
The FE-TEM results give a clear indication regarding the shape and size of the nanoparticles (CitationArunachalam and Annamalai 2013). The silver nanoparticles synthesized in our study measured 10–30 nm, and were spherical ( and ). The gold nanoparticles formed were spherical and measured 10–40 nm ( and ). Previous reports showed that the gold nanoparticles synthesized by red ginseng root were spherical and measured 2–40 nm, while silver nanoparticles formed by dried P. ginseng root were in the range of 100 nm (CitationLeonard et al. 2011, CitationVimalanathan et al. 2013). Moreover, the biosynthesized silver and gold nanoparticles were uniform and monodisperse in nature. () showed the fringe spacing of 0.23 nm of spherical silver and its corresponding selected-area electron diffraction (SEAD) and FFT images ( and ) respectively. The diffraction pattern in the form of a ring indicates the crystalline nature of the synthesized particles. The rings arise due to reflections from the (111), (200), (220), and (311) lattice planes of FCC silver, respectively. () shows the fringe spacing of 0.24 nm of spherical gold, and its corresponding SEAD and FFT images ( and ) respectively. The rings in the electron diffraction pattern resemble the (111), (200), (220), and (311) reflections of the crystalline gold nanoparticles (CitationVerma et al. 2010).
Figure 2. TEM image of spherically-shaped silver nanoparticles at 10 nm (a) and 20 nm (b). Corresponding TEM image of gold nanoparticles at 10 nm (c) and 20 nm (d).
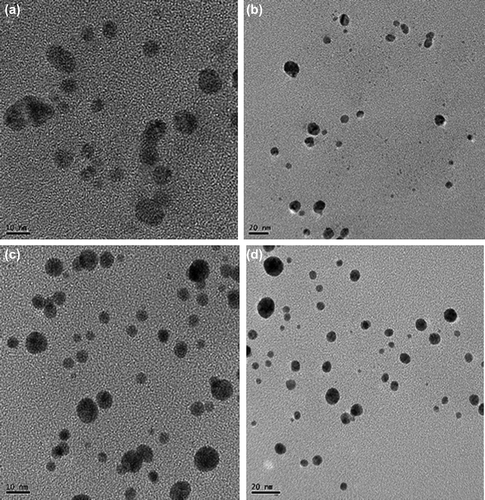
Figure 3. FE-TEM image of spherically-shaped silver nanoparticles showing fringe spacing of 0.23 nm (a), and their corresponding SAED (b) and FFT images (c). FE-TEM image of spherically-shaped gold nanoparticles showing fringe spacing of 0.24 nm (d), and its corresponding SAED (e) and FFT images (f).
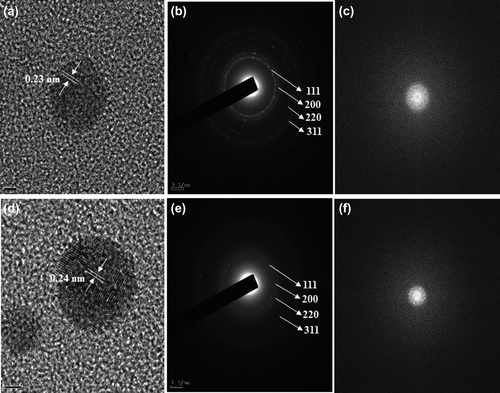
In the EDX analysis of silver nanoparticles, strong signals were observed from the silver atoms in the nanoparticles at 3 keV, which is a characteristic peak of metallic silver nanocrystals (). The results were in line with previous reports of EDX spectra of silver nanoparticles (CitationGeethalakshmi and Sarada 2012). Other signals originated from carbon and copper, which is because of the TEM grid. Further, biosynthesized silver nanoparticles were also characterized by elemental mapping. The elemental mapping results revealed that in the electron micrograph region of synthesized product (), the distribution of silver nanoparticles was 54.73% (). For gold nanoparticles, in the EDX spectrum, the strong peaks were observed at 2.2 keV () (CitationGeethalakshmi and Sarada 2012). In elemental mapping results, the maximum deposition of elements (52.16%) corresponds to the gold element ( and ).
Figure 4. EDX spectrum of silver nanoparticles (a), elemental mapping results indicate the distribution of elements, the TEM micrograph of silver nanoparticle pellet solution (b), and silver; green (c), respectively. The EDX spectrum of gold nanoparticles (d), TEM micrograph of gold nanoparticle pellet solution (e), and gold; green (f), correspondingly.
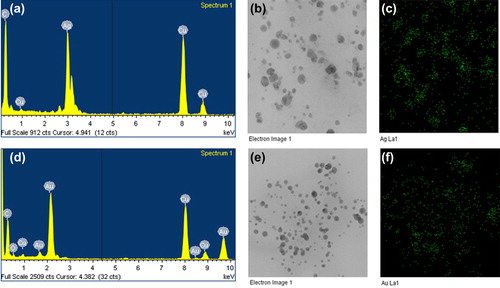
To investigate the purity of silver and gold nanoparticles, XRD analysis was done. The XRD results confirmed the purity of the synthesized silver and gold nanoparticles ( and ), respectively. The peaks in the XRD pattern corresponding to the (111), (200), (220) and (311) planes, confirmed the nanoparticles’ crystalline nature in the FCC structure, which was also confirmed by bright circular spots in the SAED and clear lattice fringes in the high-resolution TEM image. The XRD analysis showed diffraction peaks corresponding to the FCC structure and the crystallinity of silver and gold nanoparticles (CitationGarg et al. 2014, CitationVerma et al. 2010).
The pH of silver and gold nanoparticles synthesized using fresh root extract of P. ginseng was stable, with no major shift in absorbance when 0.1 M NaOH was added. On studying the effect of pH, a range from 3–12 resulted in no major change in the absorbance, which confirmed the stability of the silver and gold nanoparticles. In addition, even after 3 weeks of synthesis, there was no observable variation in the UV-Vis spectrum of the reaction mixture, which indicated the stable nature of the nanoparticles. The P. ginseng root is known to have many ginsenosides, including Rb2, Rb3, Rd, Rg1, Rc, Re, and Rb1. These components of the P. ginseng root may play an important role in the rapid synthesis and stabilization of synthesized nanoparticles (CitationKim et al. 2014, CitationShi et al. 2007, CitationVimalanathan et al. 2013). The stability of the nanoparticles over a wide range of pH levels implies the possibility of using P. ginseng-mediated nanoparticles in drug delivery applications.
Many studies have shown the antimicrobial activity of silver nanoparticles against various pathogenic and multidrug-resistant microorganisms (CitationDar et al. 2013, CitationGeethalakshmi and Sarada 2012, CitationLara et al. 2010). In our study, the antimicrobial activities of synthesized silver nanoparticles, as compared to standard antibiotics, have been shown in (). The strains E. coli and V. parahaemolyticus are completely resistant to the antibiotics, but showed a zone of inhibition when treated with silver nanoparticles. The other pathogenic strains, B. anthracis, B. cereus and S. aureus, showed sensitivity to both standard antibiotics and silver nanoparticles. The silver nanoparticles showed a maximum antimicrobial activity against S. aureus, followed by B. anthracis, B. cereus, E. coli, and V. parahaemolyticus. In the case of gold nanoparticles, no activity was observed at the same concentration. Thus, the biological approach used for the synthesis of silver and gold nanoparticles is highly eco-friendly and possesses distinct advantages such as stability and monodispersity, as compared to the more traditional physiochemical methods which often involve the use of hazardous chemicals.
Figure 6. Antimicrobial activity of nanoparticles in comparison with antibiotics against Escherichia coli [ATCC 10798], Bacillus cereus [ATCC 14579], Staphylococcus aureus [ATCC 6538], Vibrio parahaemolyticus [ATCC 33844], and Bacillus anthracis [NCTC 10340]. Note: MY15; lincomycin- 15 μg/disc, OL15; oleandomycin-15 μg/disc, NV30; novobiocin-30 μg/disc, VA30; vancomycin-30 μg/disc, P10; penicillin G-10 μg/disc, RD5; rifampicin-5 μg/disc, silver nanoparticles; silver nanoparticles-50 μL (100 mg/L).
![Figure 6. Antimicrobial activity of nanoparticles in comparison with antibiotics against Escherichia coli [ATCC 10798], Bacillus cereus [ATCC 14579], Staphylococcus aureus [ATCC 6538], Vibrio parahaemolyticus [ATCC 33844], and Bacillus anthracis [NCTC 10340]. Note: MY15; lincomycin- 15 μg/disc, OL15; oleandomycin-15 μg/disc, NV30; novobiocin-30 μg/disc, VA30; vancomycin-30 μg/disc, P10; penicillin G-10 μg/disc, RD5; rifampicin-5 μg/disc, silver nanoparticles; silver nanoparticles-50 μL (100 mg/L).](/cms/asset/6bc57958-98a6-4b04-995f-158f96266de6/ianb_a_1011809_f0006_oc.jpg)
Conclusion
The use of natural and environmental resources provides a wide range of possibilities for the further development of biological and ecofriendly routes for nanoparticle synthesis. In our study, we utilized the root extract of P. ginseng, an herbal medicinal plant, which can reduce and stabilize nanoparticles. The root extract reduces the silver salts to silver nanoparticles, within 2 h of reaction. The gold nanoparticles were also successfully synthesized by the reduction of auric acid. This simple and rapid method offers numerous benefits over other physical and chemical methods, including safety, cost reduction, absence of toxic chemicals, as well as low energy and time consumption. Further, this process provides a wide range of potential applications of these nanoparticles on a biological platform. To our knowledge, this is first report where fresh root extract of P. ginseng was found to be suitable for this action. The silver and gold nanoparticles that have been synthesized are spherical in shape, measuring10–30 nm and 10–40 nm in size, respectively. Moreover, the silver nanoparticles exert an antimicrobial property. The results obtained from the evaluation of the physiochemical, biostability, and biological properties of nanoparticles obtained from P. ginseng root extract support its application in the medical background. This approach is quite simple and eco-friendly, and can be scaled up for large-scale production. In the future, selection of such medicinal plants with high therapeutic and pharmacologic significance will create a new standard for the synthesis of various nanoparticles.
Acknowledgments
This work was supported by a post-doctoral fellowship grant from the Kyung Hee University in 20120351.
Declaration of interest
The authors report no declarations of interest. The authors alone are responsible for the content and writing of the paper.
Highlights
Biosynthesis of silver and gold nanoparticles by the fresh roots of Panax ginseng.
Silver and gold nanoparticles synthesized within 2 h and 5 min, respectively.
Characterization by UV-Vis, TEM, EDX, elemental mapping, and XRD.
Silver and gold nanoparticles were spherical and measured 10–30 and 10–40 nm respectively.
Biological activity of synthesized nanoparticles.
References
- Arunachalam KD, Annamalai SK. 2013. Chrysopogon zizanioides aqueous extract mediated synthesis, characterization of crystalline silver and gold nanoparticles for biomedical applications. Int J Nanomedicine. 8:2375–2384.
- Birla SS, Gaikwad SC, Gade AK, Rai MK. 2013. Rapid synthesis of silver nanoparticles from Fusarium oxysporum by optimizing physicocultural conditions. Scientific World Journal. 2013:12.
- Dar MA, Ingle A, Rai M. 2013. Enhanced antimicrobial activity of silver nanoparticles synthesized by Cryphonectria sp. evaluated singly and in combination with antibiotics. Nanomedicine. 9: 105–110.
- Das V, Thomas R, Varghese R, Soniya EV, Mathew J, Radhakrishnan EK. 2014.Extracellular synthesis of silver nanoparticles by the Bacillus strain CS 11 isolated from industrialized area. 3 Biotech. 4:121–126.
- Elavazhagan T, Arunachalam K D. 2011. Memecylon edule leaf extract mediated green synthesis of silver and gold nanoparticles. Int J Nanomedicine. 6:1265–1278.
- Garg S, Chandra A, Mazumder A, Mazumder R. 2014. Green synthesis of silver nanoparticles using Arnebia nobilis root extract and wound healing potential of its hydrogel. Asian J Pharm. 8:95–101.
- Geethalakshmi R, Sarada DV. 2012. Gold and silver nanoparticles from Trianthema decandra: synthesis, characterization, and antimicrobial properties. Int J Nanomedicine. 7:5375–5384.
- Hu SY. 1976. The genus Panax (Ginseng) in chinese medicine. Econ Bot. 30:11–28.
- Khalil MMH, Ismail EH, EL-Magdoub F. 2012. Biosynthesis of Au nanoparticles using olive leaf extract: 1st nano updates. Arabian J Chem. 5:431–437.
- Khan Z, Singh T, Hussain JI, Obaid AY, AL-Thabaiti SA, EL-Mossalamy EH. 2013. Starch-directed green synthesis, characterization and morphology of silver nanoparticles. Colloids Surf B Biointerfaces. 102:578–584.
- Kim K. 2015. Effect of ginseng and ginsenosides on melanogenesis and their mechanism of action. J Ginseng Res. 39:1–6.
- Kim YJ, Jeon JN, Jang MG, Oh JY, Kwon WS, Jung SK, Yang DC. 2014. Ginsenoside profiles and related gene expression during foliation in Panax ginseng Meyer. J Ginseng Res. 38:66–72.
- Lara H, Ayala-Núñez N, Ixtepan Turrent L, Rodrguez Padilla C. 2010. Bactericidal effect of silver nanoparticles against multidrug- resistant bacteria. World J Microbiol Biotechnol. 26:615–621.
- Leonard K, Ahmmad B, Okamura H, Kurawaki J. 2011. In situ green synthesis of biocompatible ginseng capped gold nanoparticles with remarkable stability. Colloids Surf B Biointerfaces. 82:391–396.
- Majdalawieh A, Kanan MC, EL-Kadri O, Kanan SM. 2014. Recent advances in gold and silver nanoparticles:synthesis and applications. J Nanosci Nanotechnol. 14:4757–4780.
- Mathiyalagan R, Subramaniyam S, Kim YJ, Kim YC, Yang DC. 2014. Ginsenoside compound K-bearing glycol chitosan conjugates: synthesis, physicochemical characterization, and in vitro biological studies. Carbohydr Polym. 112:359–366.
- MubarakAli D, Thajuddin N, Jeganathan K, Gunasekaran M. 2011. Plant extract mediated synthesis of silver and gold nanoparticles and its antibacterial activity against clinically isolated pathogens. Colloids Surf B Biointerfaces. 85:360–365.
- Radad K, Gille G, Liu L, Rausch WD. 2006. Use of ginseng in medicine with emphasis on neurodegenerative disorders. J Pharmacol Sci. 100:175–186.
- Sathishkumar M, Sneha K, Kwak IS, Mao J, Tripathy SJ, Yun YS. 2009. Phyto-crystallization of palladium through reduction process using Cinnamom zeylanicum bark extract. J Hazard Mater. 171: 400–404.
- Shi W, Wang Y, Li J, Zhang H, Ding L. 2007. Investigation of ginsenosides in different parts and ages of Panax ginseng. Food Chem. 102:664–668.
- Velmurugan P, Anbalagan K, Manosathyadevan M, Lee KJ, Cho M, Lee SM, et al. 2014. Green synthesis of silver and gold nanoparticles using Zingiber officinale root extract and antibacterial activity of silver nanoparticles against food pathogens. Bioprocess Biosyst Eng. 37:1935–1943.
- Verma V, Singh S, Solanki R, Prakash S. 2010. Biofabrication of anisotropic gold nanotriangles using extract of endophytic Aspergillus clavatus as a dual functional reductant and stabilizer. Nanoscale Res Lett. 6:1–7.
- Vimalanathan AB, Tyagi V, Rajesh A, Devanand P, Tyagi MG. 2013. Biosynthesis of silver nanoparticles using chinese white ginseng plant root panax ginseng. Asian J Biomed Pharm Sci. 34:1–6
- Wen J, Zimmer EA. 1996. Phylogeny and biogeography of Panax L. (the ginseng genus, araliaceae): inferences from ITS sequences of nuclear ribosomal DNA. Mol Phylogenet Evol. 6:167–177.