Abstract
Cell encapsulation has been investigated as a bioproduction system in the biomedical and pharmaceutical fields. We encaps-ulated human induced pluripotent stem (hiPS) cells in duplex microcapsules prepared from an alginate derivative possessing phenolic hydroxyl moieties, in a single-step procedure based on two competing enzymatic reactions catalyzed by horseradish peroxidase (HRP) and catalase. The encapsulated cells maintained 91.4% viability and proliferated to fill the microcapsules following 19 days of culture. Encapsulated hiPS cells showed pluripotency comparable to that of unencapsulated cells during the cultures, as demonstrated by the expression of the SSEA-4 marker.
Introduction
Cell encapsulation in spherical hydrogels has been studied for decades as a tool for protection of cells from the external environment while still permitting oxygen, nutrient, and secretory molecule transport (CitationChang and Poznansky 1968). The microencapsulation of stem cells during proliferation and differentiation offers numerous advantages. Specific microenvironments can be designed to protect cells against hydrodynamic shear forces, modulate stem cell phenotypes, and prevent aggregation during proliferation in suspension culture (CitationVelasco et al. 2012, CitationZhang et al. 2013). The general structures of microcapsules have two categories, namely microparticles with a solid core and microcapsules with a liquid core. Cells in solid core microparticles are more physically constrained and therefore form multiple small spheroids as they proliferate. Cells in liquid core microcapsules can move and grow with less restriction, and can proliferate and aggregate as a single large spheroid (CitationHuang et al. 2012). Generally, liquid core microcapsules are prepared by liquefaction of an inner microparticle coated with a polymer envelope such as poly-L-lysine or chitosan, to accelerate proliferation and aggregate formation (CitationWang et al. 2006, CitationZhang et al. 2013). However, the conventional method for producing cell-enclosing microcapsules with a liquid core requires several steps of coating and liquefying that may stress cells. We previously proposed a new single-step procedure for the production of cell-enclosing microcapsules with a hollow core structure, using competing reactions catalyzed by horseradish peroxidase (HRP) and catalase (CitationAshida et al. 2013). These microcapsules are prepared from an alginate derivative possessing phenolic hydroxyl moieties (Alg-Ph). In the current study, we extended this process to the encapsulation of human induced pluripotent stem (hiPS) cells. We also evaluated the viability, proliferative capacity, and pluripotency of hiPS cells in these microcapsules.
Materials and methods
Materials
Human iPS cells 201B7 (CitationTakahashi et al. 2007) were provided by RIKEN BRC (Ibaragi, Japan) through the national bio-resource project of MEXT, and maintained at 37°C in ReproStem medium (ReproCELL Inc., Kanagawa, Japan) supplemented with 5 ng/ml basic fibroblast growth factor (bFGF: ReproCELL) on mitomycin C-treated mouse STO fibroblast cells, under a humidified atmosphere containing 5% CO2. Mitomycin C-treated STO cells were seeded at approximately 2.9 × 104 cells/cm2 in Dulbecco's modified Eagle medium (Nissui Pharmaceutical, Tokyo, Japan) supplemented with 10% (v/v) fetal bovine serum (Biofill, Victoria, Australia), 75 μg/ml penicillin, and 50 μg/ml streptomycin on culture dishes pre-coated with 0.1% (w/v) gelatin. Human iPS cells were passaged as small clumps through a 40 μm cell strainer every 3−4 days using phosphate-buffered saline (PBS) containing 0.25% (w/v) trypsin (Sigma-Aldrich, St. Louis, MO, USA), 0.1% (w/v) collagenase IV (Invitrogen, Carlsbad, CA, USA), 20% (v/v) knockout serum replacement (Invitrogen), and 1 mM CaCl2.
Sodium alginate with a high guluronic acid content (I-1G, MW 70 000) was obtained from Kimica (Tokyo, Japan). Alginate lyase from Flavobacterium sp. was purchased from Sigma-Aldrich. Tyramine hydrochloride and liquid paraffin were products obtained from Kanto Chemical (Tokyo, Japan). Lecithin from soybeans, HRP (210 units/mg), catalase from bovine liver (1.0 × 104 units/ml), H2O2 aqueous solution (30%, w/w), and N-hydroxysulfosuccinimide were purchased from Wako Pure Chemical Industries (Osaka, Japan). Water-soluble carbodiimide hydrochloride was obtained from Peptide Institute (Osaka, Japan). Alg-Ph was prepared based on previously reported methods, through conjugation with tyramine (CitationSakai and Kawakami 2007). The Ph content in Alg-Ph was 2.9 × 10−4 mol-Ph/g-Alg-Ph. This polymer was used after sterilization with 70% (v/v) ethanol, followed by vacuum drying.
Encapsulation of hiPS cells
The microcapsules enclosing the hiPS cells were prepared from a solution containing 2.5% (w/v) Alg-Ph, 100 units/ml HRP, 9.1 × 104 units/ml catalase, and 2–3 × 106 cells/ml. The solution was extruded from a 26-gauge needle at 0.075 ml/min for 4 min into a flow of liquid paraffin containing H2O2 and 5% (w/w) lecithin. After 4 min of standing, the microcapsules were rinsed several times with ReproFF2 medium (ReproCELL) and incubated in ReproFF2 medium containing 5 ng/ml bFGF and 10 μM ROCK inhibitor Y27632 (Wako Pure Chemical Industries) at 37°C. The proliferation of encapsulated hiPS cells was examined in a series of 6 culture runs performed for 19 days and the data were recorded from 3 independent runs. The medium was replaced every other day during each culture run. The viability of cells just after encapsulation was estimated by a trypan blue exclusion assay, after recovering the cells by soaking the microcapsules in cell culture medium containing 0.2 mg/ml alginate lyase. The growth of cells within the microcapsules was assessed in terms of mitochondrial activity of cells per 100 microcapsules. The increased amount of water-soluble formazan dye derived from tetrazolium salt was measured with a colorimetric assay kit (Cell Counting Kit-8, Dojindo, Kumamoto, Japan). The absorbance (ABS) at 450 nm was determined using a spectrophotometer after 2 h of incubation of medium containing a hundred of the cell-enclosing microcapsules with the reagent at 37°C.
Cell viability staining
Living and dead cells were detected by staining with calcein AM and propidium iodide (PI), with indications of green and red colors, respectively. Cell-enclosing microcapsules were incubated in a solution containing 2 μM calcein AM and 0.5 μM PI for 30 min, and then washed with PBS. Stained cells were observed using a confocal laser scanning microscope (Model FV-300; Olympus, Tokyo, Japan).
Flow cytometric analysis
Cell aggregates harvested from Alg-Ph microcapsules were dissociated by treatment with StemPro Accutase (Invitrogen). Anti-SSEA-4 antibody conjugated with PerCP-Cy™5.5 (BD Bioscience, CA, USA) was used to assess the pluripotency of encapsulated or unencapsulated hiPS cells with a BD Accuri C6 cytometer (BD Bioscience).
Results and discussion
We prepared hiPS cells enclosed within duplex microcapsules by extruding an Alg-Ph solution containing enzymes of both HRP and catalase into liquid paraffin containing H2O2. The average diameter of microcapsules obtained was 150 ± 19 μm. To evaluate the toxicity of the encapsulation process, we determined the viability of enclosed cells immediately after encapsulation. The viability of cells recovered from the microcapsules by soaking in a solution containing alginate lyase was 91.4 ± 3.2% (n = 3), the value of which was comparable with that prior to encapsulation (93.6 ± 1.1%, n = 3).
Next, we evaluated the usefulness of the duplex structure induced by the enzymatic reactions for the growth of enclosed cells. shows the typical photograph of cells enclosed in the microcapsules immediately after encapsulation. The spherical size of cell aggregates within microcapsules increased with time to a diameter of 120 μm by day 19 after encapsulation (). As shown in , the mitochondrial activity increased with elapsed culture time, and a 33-fold increase in the activity was achieved after 16 days of cultivation, compared with that at day 2 (n = 3). A hollow core structure, such as that formed here, is believed to provide a favorable environment for cell growth, allowing cells to move with less restriction and proliferate to form a large spheroid (CitationConstantinidis et al. 1999, CitationHuang et al. 2012).
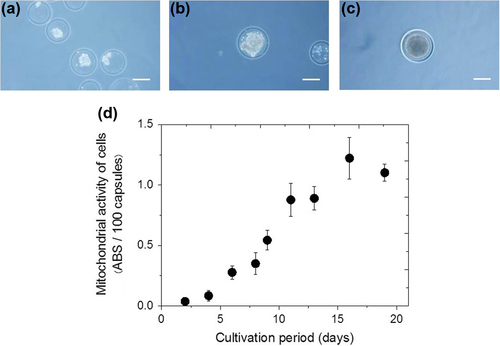
Large clumps of cells, especially those over 300 μm in diameter, experience increased cell death because the inner regions of the cell mass have limited access to nutrients and become necrotic (CitationKim et al. 1998, CitationValamehr et al. 2008). Therefore, we assessed the viability of cells within the microcapsules. Living and dead cells were detected by staining with calcein AM (green) and PI (red), respectively. As seen in , living cells existed dominantly in the microcapsules at 10 and 19 days of cultivation. Red colored signals from dead cells were never recognized at both dates ().
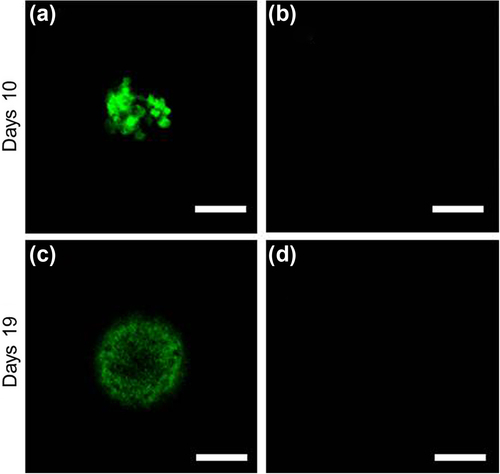
Lastly, we assessed the pluripotency of encapsulated hiPS cells by measuring the levels of extracellular SSEA-4 protein during 3 culture runs. As typically seen in , SSEA-4 protein was expressed in almost all cell aggregates at days 9 and 19; the proportions of SSEA-4-positive cells at days 9 and 19 were estimated at 93.8 ± 1.8% (n = 3) and 91.1 ± 2.4% (n = 3), respectively. The proportion of SSEA-4-positive cells was 89.9 ± 8.2% (n = 3) at day 9 during cultures of unencapsulated cells performed under similar conditions (in the case of unencapsulated cells, the cultures could not last beyond 9 days, owing to cell aggregate dissociation). These results suggest that the pluripotency of encapsulated hiPS cells was comparable to that of unencapsulated cells during the cultures. Collectively, our results demonstrated that Alg-Ph microcapsules produced using HRP- and catalase-catalyzed reactions can effectively give a proliferation space to hiPS cells.
Conclusion
We successfully encapsulated hiPS cells in Alg-Ph microcapsules prepared using a single-step procedure employing two enzymatic reactions. Cells could be enclosed within microcapsules without significant loss in cell viability. Encapsulated cells proliferated and filled the cavities during 19 days of cultivation. Moreover, hiPS cells cultivated within the microcapsules maintained pluripotency at levels comparable to those of unencapsulated cells. These results demonstrate the feasibility of using Alg-Ph microcapsules obtained through the HRP- and catalase-catalyzed reactions for the multiplication of hiPS cells.
Declaration of interest
The authors report no declarations of interest. The authors alone are responsible for the content and writing of the paper.This work was supported by the MEXT/JSPS KAKENHI Grant (No. 24360340) from the Ministry of Education, Culture, Sports, Science and Technology of Japan, and by a Grant-in-Aid for Young Scientists from the Multidisciplinary Research Laboratory System for Future Developments of the Graduate School of Engineering Science, Osaka University.
References
- Ashida T, Sakai S, Taya M. 2013. Competing two enzymatic reactions realizing one-step preparation of cell-enclosing duplex microcapsules. Biotechnol Prog. 29:1528–1534.
- Chang TMS, Poznansky MJ. 1968. Semipermeable microcapsules containing catalase for enzyme replacement in acatalsaemic mice. Nature. 218:242–245.
- Constantinidis I, Rask I, Long RC Jr, Sambanis A. 1999. Effects of alginate composition on the metabolic, secretory, and growth characteristics of entrapped βTC3 mouse insulinoma cells. Biomaterials. 20:2019–2027.
- Huang X, Zhang X, Wang X, Wang C, Tang B. 2012. Microenvironment of alginate-based microcapsules for cell culture and tissue engineering. J Biosci Bioeng. 114:1–8.
- Kim SK, Yu SH, Son JH, Hübner H, Buchholz R. 1998. Calculations on O2 transfer in capsules with animal cells for the determination of maximum capsule size without O2 limitation. Biotechnol Lett. 20:549–552.
- Sakai S, Kawakami K. 2007. Synthesis and characterization of both ionically and enzymatically crosslinkable alginate. Acta Biomater. 3:495–501.
- Takahashi K, Tanabe K, Ohnuki M, Narita M, Ichisaka T, Tomoda K, Yamanaka S. 2007. Induction of pluripotent stem cells from adult human fibroblasts by defined factors. Cell. 131:861–872.
- Valamehr B, Jonas SJ, Polleux J, Qiao R, Guo S, Gschweng EH, et al. 2008. Hydrophobic surfaces for enhanced differentiation of embryonic stem cell-derived embryoid bodies. Proc Natl Acad Sci USA. 105:14459–14464.
- Velasco D, Tumarkin E, Kumacheva E. 2012. Microfluidic encapsulation of cells in polymer microgels. Small. 8:1633–1642.
- Wang X, Wang W, Ma J, Guo X, Yu X, Ma X. 2006. Proliferation and differentiation of mouse embryonic stem cells in APA microcapsule: a model for studying the interaction between stem cells and their niche. Biotechnol Prog. 22:791–800.
- Zhang W, Zhao S, Rao W, Snyder J, Choi JK, Wang J, et al. 2013. Novel core-shell microcapsule for encapsulation and 3D culture of embryonic stem cells. J Mater Chem B Mater Biol Med. 7:1002–1009.