Abstract
Exogenous insulin and EGFP genes were transduced into bone marrow mesenchymal stem cells of beagle dogs using the retroviral vector pMSCV to prepare β-like cells. These cells were autotransplanted into the liver of diabetic dogs through hepatic arterial intervention, and physiological indices before and after transplantation were monitored. Autotransplantation of β-like cells significantly improved blood sugar, insulin levels, and body mass of diabetic dogs (P < 0.01) continuously for over 80 weeks. Since the liver function remained normal and no tumors formed, this method was determined to be reliable and safe. Intrahepatic autotransplantation of β-like cells had long-term, reliable, and safe therapeutic effects on diabetic dogs.
Introduction
Diabetes is a metabolic syndrome typified by rising blood sugar level. Injection of exogenous insulin, which cannot simulate the secretion of the endogenous one, easily leads to fluctuation of blood sugar level and even hypoglycemia (CitationJun 2010; Citationvan Dieren et al. 2010). Although patients with severe islet cell damages can be subjected to pancreas transplantation, the donors are limited and there may be immunological rejection (CitationCandiello et al. 2011). Therefore, new therapies for diabetes are still needed. Bone marrow mesenchymal stem cells (BMSCs), as bone marrow-derived adult stem cells with multi-differentiation potential, are readily accessible and amplifiable in vitro, without involving ethical issues (CitationProckop 1997; CitationPittenger et al. 1999). BMSC populations generally show low immunogenic properties, with none or low expression of CD34, CD45, and CD14 on the surface, and expressing CD105, CD44, CD49, and CD106 at different levels (CitationPhinney 2007; CitationYechoor and Chan, 2010). Besides being differentiated into insulin-secreting cells through induction (CitationXie et al. 2009), BMSCs also secrete various cytokines as well as promote repair and regeneration of islets (CitationHess et al. 2003; CitationBi et al. 2007). Meanwhile, BMSCs can form new blood vessels, increase pancreatic blood supply, and facilitate islet repair (CitationLeroux et al. 2010). Accordingly, they are promising in treating diabetes.
β-like cells prepared from BMSCs can circumvent the lack of donors and immunological rejection during transplantation for the treatment of diabetes by allowing cellular autotransplantation (CitationHager et al. 2005; CitationPrescott et al. 2013). However, the long-term outcomes after transplantation, and the answer to whether transplants have detrimental effects or tumorigenicity, remain unclear. In this study, we evaluated the effects of intrahepatic autotransplantation of β-like cells on diabetic beagle dogs as well as the long-term outcomes and safety, providing valuable evidence for further clinical use.
Experimental
Materials
Adult beagle dogs aged 9–12 months (8–12 kg) were provided by the experimental animal center of Nantong University. This study has been approved by the Ethics Committee for Animal Use of the First Affiliated Hospital of Medical College of Xi'an Jiaotong University, and all experiments were performed according to “Guidance Suggestions for the Care and Use of Laboratory Animals (2006)” issued by the Ministry of Science and Technology of the People's Republic of China.
Plasmids pMSCV, pIRES (internal ribosome entry site), and pEGFP were purchased from Clontech Laboratories, Inc. (Japan). Restriction endonucleases EcoR I and Bgl II were bought from TaKaRa Biotechnology (Dalian) Co., Ltd. Primers were synthesized by Generay Biotech (Shanghai) Co., Ltd. Other reagents were analytically pure.
Construction of co-expression vector pMSCV-Ins-EGFP and preparation of virus solution
Primers 5′-CCCTCACTCCTTCTCTAGGCGCCGGAATTCGTAAG-3′ and 5′-AGCG CCTCCCCTACCCGGTAGAATTAGATCTTTAC-3′ were designed, into the 5′- and 3′-ends of which restriction endonucleases EcoR I and Bgl II were introduced to amplify the gene fragments Ins, IRES, ivs, and EGFP by using pMSCV-Ins, pIRES, and pEGFP-N1 as the templates. The reaction system (50 μl in total) consisted of: 5 μl of 10 × buffer, 2 μl of 25 mmol/L MgSO4, 1–100 ng template, 20 pmol of primer 1, 20 pmol of primer 2, 0.2 mmol/L dNTPs, 1.25–2.5 U of taq polymerase, and ddH2O. The PCR reaction conditions were: 2 min of pre-denaturation at 95C, 30 s of denaturation at 94°C, 30 s of annealing at 55°C, 50 s of extension at 72°C, 30 cycles, and 5 min of extension at 72°C. The amplified fragments were ligated into the vector to construct pMSCV-Ins-EGFP and pMSCV-EGFP vectors. Virus solution containing Ins-EGFP and EGFP genes was obtained after PT67 cell packaging.
Preparation of GFP-labeled β-like cells
Red bone marrow (5 ml) was drawn from adult beagle dogs aged 9–12 months, from which BMSCs were obtained with the cell adhesion method (CitationLu et al. 2009) and transfected with the virus solution containing Ins-EGFP and EGFP genes. As a result, exogenous Ins and EGFP genes were expressed therein and EGFP-labeled β-like cells were prepared. Transcription of Ins and EGFP genes was detected by RT-PCR. Ins expression was detected by anti-insulin immunohistochemistry, and EGFP expression was observed under a fluorescent microscope.
Intrahepatic autotransplantation of β-like cells
Under anesthesia, the dogs were intravenously injected with 50 mg/kg of alloxan and 30 mg/kg of streptozotocin. The model of diabetes was considered successfully established if the blood sugar level was ≥ 11.1 mmol/L 48 h after injection and remained thereafter for two weeks. The dogs were divided into the following five groups (n = 8). G1: The diabetic group transplanted with β-like cells expressing Ins and EGFP genes; G2: the diabetic group transplanted with BMSCs expressing the EGFP gene; G3: the diabetic control group transplanted with BMSCs only; G4: the diabetic group without cell transplantation; G5: the normal control group. Cell transplantation was performed two weeks after establishment of the diabetic model. Under anesthesia and guidance of digital subtraction angiography, the hepatic artery was catheterized through the femoral artery to infuse 5 × 107 cell suspensions for the groups G1–G3, and PBS for the groups G4 and G5.
Observation of long-term outcomes after transplantation
Detection of blood sugar level and body mass changes: Fasting blood sugar level and body mass were measured in the morning once daily after transplantation and then twice weekly when the level stabilized. The results, at the same time as on the day of transplantation and every four weeks after transplantation, were plotted and compared. Detection of serum insulin level: After fasting overnight, about 2 ml of venous blood was drawn at the time points vide supra to detect insulin level with an insulin radioimmunoassay kit. Intravenous glucose tolerance test (IGTT): The physiological indices of the dogs gradually leveled off 20 weeks after transplantation. After 10–15 h of fasting, 50 ml of 50% glucose solution was intravenously injected within 3 min, and the blood sugar level was measured 6 times every 20 min, with the end of injection as the starting point.
Observation of safety after transplantation
Monitoring of liver function before and after transplantation: Fasting venous blood (2 ml) was drawn in the morning at the time points vide supra to detect the levels of alanine aminotransferase (ALT), aspartate aminotransferase (AST), and γ-glutamyl transpeptidase (γ-GT). Observation of liver pathological morphology: Liver samples were collected 80 weeks after transplantation, cryopreserved, cut into sections, subjected to immunofluorescence staining (indirect method, with rabbit anti-insulin antibody as the primary body and goat anti-rabbit antibody as the secondary antibody), and observed under a fluorescent microscope, aiming to locate the transplanted cells in the liver, to detect the expression of insulin in liver tissues, and to find out whether there were tumors. Spiral CT examination: CT scanning was conducted every 4 weeks after transplantation to observe the formation of tumors.
Statistical analysis
All data were expressed as ± s and analyzed by Stata7.0. Inter-group comparisons were performed with the t-test. A value of P < 0.05 was considered statistically significant.
Results
Successful construction of pMSCV-Ins-EGFP and preparation of virus solution
By using PCR amplification, ivs, Ins, IRES and EGFP fragments were obtained from pMSCV-Ins, pIRES, and pEGFP vectors respectively (), which were subjected to double digestion with EcoR I and Bgl II and then ligated to pMSCV to construct pMSCV-Ins-IRES-EGFP and pMSCV-IRES-EGFP retroviral vectors. The identification results of double digestion are shown in . As suggested by the bands of IRES-EGFP and Ins-IRES-EGFP after digestion ( and ), the construction was indeed successful. Infectious viruses were prepared from PT67 cell-packaged reconstructed vectors, the titers of which were determined as (4∼6)× 106 cfu/ml.
Figure 1. Construction of pMSCV-Ins-EGFP. (A) PCR fragments; 1: ivs (230 bp); 2: Ins (333 bp); 3: IRES (656 bp); 4: EGFP (754 bp); (B) identification results of EcoR I/Bgl II double digestion; 1: Ins-IRES- EGFP; 2: IRES-EGFP; 3: linear MSCV fragment (6.6 kb); (C) IRES-EGFP after digestion; (D) Ins-IRES-EGFP fragment after digestion.
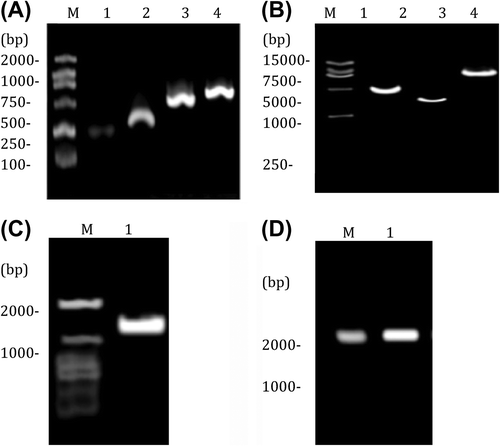
Preparation of GFP-labeled β-like cells
BMSCs isolated from beagle dogs had uniform morphology and high proliferative capacity after in vitro amplification (). GFP-labeled β-like cells were obtained after co-transfection of BMSCs with Ins and EGFP genes (). Immunohistochemical staining (DAB method) showed that the cytoplasm was stained brownish yellow (), indicating that insulin was abundantly expressed. RT-PCR results showed that exogenous Ins and EGFP were transcribed in BMSCs that had been transfected for 7, 14, and 28 days, with clear bands ().
Figure 2. Preparation of GFP-labeled β-like cells. (A) Morphology of BMSCs under an inverted fluorescent microscope (× 40); (B) EGFP expression in β-like cells after stable transfection (green fluorescence excited at 488 nm, × 40); (C) results of immunohistochemical staining of β-like cells (× 40); D: RT-PCR results of β-like cells, 7, 14, and 28 days after stable transfection.

Therapeutic effects of β-like cells on diabetes
The blood sugar levels, body mass measurements, serum insulin levels before and after intrahepatic autotransplantation, as well as IGTT results are shown in . Obviously, the G1 group had significantly better outcomes than those of the G2–G4 groups (P < 0.05), which, however, still failed to be comparable to those of G5 group (P < 0.05).
Safety after transplantation
The liver tissue section of the G1 group showed evident green fluorescence after immunohistochemical staining (), while red fluorescence was observed in the same region after insulin immunohistochemical staining (), suggesting that insulin was highly expressed. and exhibit the cell nuclear staining and merged fluorescence image respectively. Moreover, HE staining of liver sections ( and ) displayed that after transplantation, hepatocytes were arranged orderly, with clear lobular structure and without apparent fibrosis or tumor formation. CT scanning also detected no tumors (). Since the levels of ALT, AST, and γ-GT fluctuated within the normal ranges (P > 0.05) (), intrahepatic autotransplantation of β-like cells barely affected liver function.
Figure 4. Liver morphology after transplantation. (A) Expression of GFP gene in cells under the fluorescent microscope (× 20); (B) abundant insulin expression in the cytoplasm of the same region (× 20); (C) cell nuclear staining (× 20); (D) merged image (× 20); (E) results of HE staining of normal liver tissue section (× 40); (F) results of HE staining of liver after transplantation (× 40); (G) CT scanning results (tumors were not detected).
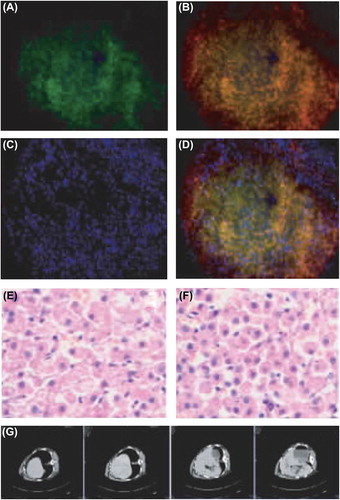
Discussion
Diabetes has become the second most dangerous chronic disease threatening human health, after malignant tumors (Citationvan Dieren et al. 2010), which cannot be cured by traditional drugs. Although pancreatic islet transplantation is fairly promising, its application in clinical practice is limited owing to insufficient donor cells and transplant rejection. BMSCs, on the other hand, are advantageous owing to easy availability of samples, rich resources, and great multi-differentiation potential (CitationYechoor and Chan 2010). Donor insufficiency and immune rejection can be circumvented by using BMSCs as seed cells to prepare β-like cells that allow autotransplantation to treat diabetes.
Global researchers have paid particular attention to the continuous and stable expressions of exogenous genes. Retroviral vector MSCV enhances the transcription activation of viral vector MMLV and decreases the transcription inhibition, thus managing to transfect cells with complex functions (e.g., hematopoietic stem cells, embryonic stem cells, and BMSCs) and to elevate the stable expression efficiencies of exogenous genes in BMSCs (CitationMayrhofer et al. 2008; CitationTolmachov 2010). IRES allows translation of two open reading frames from one mRNA and that of different proteins from the same transcript, and GFP-labeled β-like cells can be prepared by ligating the Ins gene to the EGFP gene with IRES.
The liver has rich vascular networks, with blood supplied by the portal vein and the hepatic artery, and oxygen supplied by the hepatic artery. As to blood sugar regulation, the liver synthesizes and decomposes glycogen, as well as accommodates insulin to first exert effects after entering the bloodstream. After being intrahepatically transplanted, β-like cells can secrete insulin that immediately works, so these cells are eligible for treating diabetes (CitationCalafiore et al. 2014). Since the life span of beagle dogs ranges between those of human and murine, they have patterns of disease onset and clinical response similar to those of humans, and have been widely employed to study long-course diseases. In addition, the success rates of establishing diabetic dog models are commonly high (CitationStarkey et al. 2005; CitationSwitonski et al. 2004). In the meantime, the physiological functions of beagle dogs are mildly affected when a small amount of bone marrow is collected to extract BMSCs. Thereby motivated, we herein prepared β-like cells that co-expressed Ins and EGFP through gene transfection by using BMSCs as seed cells, and then autotransplanted them into the liver of diabetic beagle dogs through hepatic arterial intervention under the guidance of digital subtraction angiography. Afterwards, the location of these autotransplanted cells in the liver was observed and the long-term effects on diabetes were assessed by tracing EGFP.
The body mass of diabetic beagle dogs stopped decreasing 7 days after intrahepatic autotransplantation of β-like cells, gradually increased, and remained stable thereafter. Diabetes was relieved for over 80 weeks, which was, however, still worse than in normal dogs. Moreover, exogenous insulin was only secreted in the serum of dogs receiving autotransplantation, so the β-like cells had long-term, stable effects on diabetic dogs. Notably, the β-like cells were not tumorigenic or adverse to the liver function, as evidenced by detection of liver physiological functions and pathological morphology as well as CT scanning. Hence, the β-like cells prepared from BMSCs by Ins gene transfection had high reliability and biosafety.
Although the β-like cells we prepared herein were effective and safe, they were inferior to mature β cells in the negative results of glucose-stimulating insulin secretion. Therefore, the mechanism for the regulatory effects of β cells on blood sugar level should be clarified to prepare β-like cells remarkably resembling mature β-cells, with higher output, paving the way for clinical treatment of diabetes.
Declaration of interest: The authors report no conflicts of interest. The authors alone are responsible for the content and writing of the paper.
References
- Bi B, Schmitt R, Israilova M, Nishio H, Cantley LG. 2007. Stromal cells protect against acute tubular injury via an endocrine effect. J Am Soc Nephrol. 18:2486–2496.
- Calafiore R, Montanucci P, Basta G. 2014. Stem cells for pancreatic beta-cell replacement in diabetes mellitus: actual perspectives. Curr Opin Organ Transplant. 19:162–168.
- Candiello JE, Jaramillo M, Goh SK, Banerjee I. 2011. Role of substrates in diabetes therapy: stem cell differentiation and islet transplantation. Crit Rev Biomed Eng. 39:535–555.
- Hager DN, Krishnan JA, Hayden DL, Brower RG, Network ACT. 2005. Tidal volume reduction in patients with acute lung injury when plateau pressures are not high. Am J Respir Crit Care Med. 172: 1241–1245.
- Hess D, Li L, Martin M, Sakano S, Hill D, Strutt B, et al. 2003. Bone marrow-derived stem cells initiate pancreatic regeneration. Nat Biotechnol. 21:763–770.
- Jun HS. 2010. Cell replacement and regeneration therapy for diabetes. Korean Diabetes J. 34:77–83.
- Leroux L, Descamps B, Tojais NF, Séguy B, Oses P, Moreau C, et al. 2010. Hypoxia preconditioned mesenchymal stem cells improve vascular and skeletal muscle fiber regeneration after ischemia through a Wnt4-dependent pathway. Mol Ther. 18:1545–1552.
- Lu YH, Zhu MY, Chen J, Wang ZW, Qian HX. 2009. [The Study of Nesting and Therapy of Bone-marrowed Murine Mesenchymal Stem Cells of Diabetic Mice]. Suzhou Univ J Med Sci. 29:436–438, 445.
- Mayrhofer P, Blaesen M, Schleef M, Jechlinger W. 2008. Minicircle-DNA production by site specific recombination and protein-DNA interaction chromatography. J Gene Med. 10:1253–1269.
- Phinney DG. 2007. Biochemical heterogeneity of mesenchymal stem cell populations: clues to their therapeutic efficacy. Cell Cycle. 6:2884–2889.
- Pittenger MF, Mackay AM, Beck SC, Jaiswal RK, Douglas R, Mosca JD, et al. 1999. Multilineage potential of adult human mesenchymal stem cells. Science. 284:143–147.
- Prescott HC, Brower RG, Cooke CR, Phillips G, O’Brien JM. 2013. Factors associated with elevated plateau pressure in patients with acute lung injury receiving lower tidal volume ventilation. Crit Care Med. 41:756–764.
- Prockop DJ. 1997. Marrow stromal cells as stem cells for nonhematopoietic tissues. Science. 276:71–74.
- Starkey MP, Scase TJ, Mellersh CS, Murphy S. 2005. Dogs really are man's best friend–canine genomics has applications in veterinary and human medicine! Brief Funct Genomic Proteomic. 4:112–128.
- Switonski M, Szczerbal I, Nowacka J. 2004. The dog genome map and its use in mammalian comparative genomics. J Appl Genet. 45:195–214.
- Tolmachov OE. 2010. Tightly-wound miniknot vectors for gene therapy: a potential improvement over supercoiled minicircle DNA. Med Hypotheses. 74:702–704.
- van Dieren S, Beulens JW, van der Schouw YT, Grobbee DE, Neal B. 2010. The global burden of diabetes and its complications: an emerging pandemic. Eur J Cardiovasc Prev Rehabil. 17 Suppl 1:S3–8.
- Xie QP, Huang H, Xu B, Dong X, Gao SL, Zhang B, et al. 2009. Human bone marrow mesenchymal stem cells differentiate into insulin-producing cells upon microenvironmental manipulation in vitro. Differentiation. 77:483–491.
- Yechoor V, Chan L. 2010. Minireview: beta-cell replacement therapy for diabetes in the 21 st century: manipulation of cell fate by directed differentiation. Mol Endocrinol. 24:1501–1511.