Abstract
Increasing the size of hemoglobin (Hb) by polymerization offers the benefits of reduced renal clearance and increased duration in the vascular circulation. With this goal, diaspirin cross-linked hemoglobin (DCLHb) was modified in order to keep one thiol group on the surface and then polymerized with 1,6-bismaleimic-hexane (1,6-BMH) to increase the molecular weight. The HPLC results indicated that approximate 20% dimers to tetramers of DCLHb desired were achieved after the polymerization. It was also demonstrated that the oxygen-carrying capacity of the products was similar to natural heme. The present study is expected to improve the efficacy of the DCLHb as an oxygen therapeutic agent.
Introduction
The primary biological function of natural red blood cells (RBCs) is to transport hemoglobin-bound oxygen and carbon dioxide to and from tissues, respectively. Hemoglobin, by which in the RBCs oxygen is carried, is a tetramer of two α- and two β-polypeptide chains, each bound to an iron-containing heme group capable of binding one oxygen molecule. The molecular weight (MW) of hemoglobin is about 64,500, whose subunits are noncovalently associated through van der Waals forces, hydrogen bonds, and salt bridges.
Administering a cell-free suspension of hemoglobin as oxygen carrier has numerous advantages such as a reduced need for compatibility testing, and the ability to sterilize by ultrafiltration or low heat (Squires Citation2002). However, unmodified cell-free human hemoglobin is not suitable for use as an oxygen-delivering therapeutic agent. First, due to the lack of 2,3-diphosphoglycerate (2,3-DPG), the oxygen affinity of extracellular human hemoglobin is too high to deliver oxygen to tissues effectively (Christa et al. Citation2013). Then, extracellular hemoglobin is prone to dissociate into α–β dimers in the circulation; high concentrations of these dimers overwhelm the haptoglobin scavenging system and accumulate in the tubules of the kidney, where they are nephrotoxic (Buehler et al. Citation2010, Chang Citation2006, Haney et al. Citation2005, Pimenova et al. Citation2009). Furthermore, extracellular hemoglobin results in vasoconstriction and hypertension by NO, inhibiting endothelial cell-relaxing factors (Rioux et al. Citation1995). Finally, extracellular hemoglobin can alter the blood volume and change the colloidal osmotic pressure of the blood.
In order to improve the safety and efficacy of hemoglobin, the tetrameric hemoglobin has been intramolecular cross-linked between the dimeric subunits of the native hemoglobin (Gao et al. Citation2013). Hence the duration time in the vascular circulation increases and the renal toxicity reduces (Bolin and DeVenuto Citation1983, Labrude et al. Citation1986). For example, bis-(3,5-dibromosalicyl)fumarate (DBBF; Chatterjee et al. Citation1986), a cross-linking reagent, which creates cross-links between the α and β chains to form diaspirin cross-linked hemoglobin (DCLHb), showed an increase in circulation time up to 12 h compared to <6 h for untreated hemoglobin (Squires Citation2002). In addition, bifunctional cross-linking reagent such as glutaraldehyde has also been used to create polyhemoglobin molecules (Bian et al. Citation2013, Sehgal et al. Citation1983). However, it is hard to control polymer MW and consistent quality of the products. In other words, the polymerized products were mixtures of hemoglobin, oligomers of hemoglobin, and polymers of hemoglobin having a spectrum of sizes and MW. If a product in a specific size range was desired, it had to be separated from the bulk product mixture by chromatographic or ultrafiltration methods. The separation of such product mixtures into the various size ranges on a commercial scale is technically difficult, time consuming, and costly.
Although DCLHb and other cross-linked hemoglobins were successful in overcoming most of the shortcomings of cell-free hemoglobin, the full potential of extracellular hemoglobin solutions as an oxygen-delivering therapeutic agent could not be met by tetrameric hemoglobin solutions. A primary drawback of chemically modified tetrameric hemoglobins, such as DCLHb, was the limited duration of the hemoglobin in the circulatory system (Buehler et al. Citation2010, Haney et al. Citation2005, Pimenova et al. Citation2009). In general, larger hemoglobins are retained longer in the vascular system, but those polyhemoglobin molecules that are too large are prone to aggregation and/or precipitation. Therefore, polymerization of DCLHb was directed to obtain a polymerized DCLHb (polyDCLHb) product having hemoglobin molecules with molecular mass in the range of 130–260 kDa.
With this in mind, it is necessary to increase the molecular size of the resulting hemoglobin composition. Further modifications to the DCLHb have been developed to extend the circulatory duration of the hemoglobin. Moreover, whether the molecular mass could be controlled in a proper range is also of great importance for DCLHb to improve the efficiency. In the present study, DCLHb reacted first with bromoacetic acid to block the residual thiol groups of protein chains, and then an amino group on the surface of one DCLHb tetramer was converted into thiol group with 2-iminothiolane hydrochloride (2-IT); therefore, a thiol group was located on the DCLHb, which was called “DCLHb-SH” and could react with cross-linking reagent (). Next, the “DCHLb-SH” reacted with 1,6-bismaleimic-hexane (1,6-BMH, ) so that a specific intermolecular cross-linking could be formed between two converted thiol groups (Hai et al. Citation1998), aiming at an appropriate increase of molecular mass of hemoglobin (). The properties of the cross-linked DCLHb were characterized, and P50 (the O2 pressure at which Hb is half saturated) of the product was also evaluated. This research explores a novel approach to produce oligomers of DCLHb within a proper MW range by specific cross-linking, hence preventing the formation of high molecular weight (HMW) polymers.
Experimental
Reagents and instruments
Human umbilical cord blood was obtained from Tianjin Stem Cell Gene Engineering Company. Maleic anhydride, 1,6-diaminohexane, fumaryl chloride, and 2-IT were purchased from Aladdin Reagent Co. Ltd. (Shanghai, China) and used without further purification. Other chemicals used were of analytical grade, and the solutions were prepared with deionized water.
Spectrophotometry was conducted using SHIMADZU UV-2550, and analytical RP-HPLC was carried out with SHIMADZU CBM-10AVP. Multiangle laser light scattering (MALLS) was performed using Wyatt DAWN HELEOSII. The NMR test was performed by NanoBay 400 MHz (Avance™III HD, Bruker), and oxygen equilibrium measurements of samples were carried out using Hemox analyzer (TCS Scientific, PA)
Methods
Hemoglobin – Purified Hb from human placentas was prepared following the methods described by Walder et al. (Citation1994). The final product in the retentates was collected and stored at −80 °C.
Synthesis of 1,6-BMH – maleic anhydride (19.6 g, 0.2 mol) was combined with 100 mL of DMF. The mixture was stirred at RT until all the maleic anhydride dissolved in the solvent, then 1,6-diaminohexane (12 g, 0.1 mol) was added in one portion to the solution. The mixture was heated to 90 °C for 0.5 h under N2 and cooled. To this solution, 3 mg of nickel diacetate, 1 mL of triethylamine, and 40 mL of acetic hydride were added. The mixture was reacted for another 0.5 h at 90 °C under N2. After cooling, the resulted red–brown solution was poured into 800-mL ice water. The mixture was allowed to stand overnight and a dark brown solid was formed. The solid was collected by filtration and recrystallized twice with ethyl acetate. The final product was dried under vacuum to give 11.3 g (41%) of 1,6-BMH, brown crystals. mp: 139–140 °C. 1H NMR (CDCl3, ppm) δ 6.70 (s, 4H), 3.50 (t, 4H), 1.57 (m, 4H), 1.31 (m, 4H).
Synthesis of DCLHb – Purified Hb was intramolecularly modified by DBBF to form DCLHb, this preparation was carried out as described earlier (Walder et al. Citation1979).
Preparation of DCLHb-SH and polymerization of DCLHb-SH with 1,6-BMH – 16 mL of unpurified DCLHb solution (15.6 μmol, total content of Hb) was deoxygenated by successive vacuum/nitrogen cycles for 2 h, then cooled to 0 °C. A solution of bromoacetic acid (31.2 μmol) in 0.2-M phosphate-buffered saline (PBS) buffer (2 mL, pH 7.4) was introduced rapidly to the deoxy-DCLHb solution. The reaction mixture was maintained at 0 °C and stirred under nitrogen for 2 h to block the thiol groups of Hb. Then, 5-mg 2-IT was added to the solution and the reaction continued at 4 °C for 3 h. This procedure was carried out in order to convert amino groups into corresponding thiol groups. Next, 1,6-BMH (0.6 molar equivalent of Hb) was added. The solution was stirred at 5 °C under nitrogen for 3 h and the final polymerized product was concentrated to 6.4 ± 0.2 g per dL into sterile glass bottles, and then stored frozen at –20 °C for further characterization.
Sodium dodecyl sulfate-polyacrylamide gel electrophoresis (SDS-PAGE) was performed based on the method of Laemmli (Citation1970).
UV-Vis wavelength scanning – UV-Vis full wavelength scanning of the Hb solution; DCLHb-SH solution and the polyDCLHb solution were recorded in 200–700 nm range.
Size exclusion chromatography-MALLS (SEC-MALLS).
Molecular size and polydispersity were determined for each Hb fraction by SEC coupled to MALLS. SEC-MALLS data were acquired and analyzed with Astra version 5.1 software (Wyatt Technology Corp., Santa Barbara, CA, USA). Samples were injected in 25-μL volume and separated on a SuperdexTM 200 Increase 10/300 GL column (300 × 10 mm), eluted in 0.1-M PBS, pH 7.4, at 0.5 mL/min. The sample passed through a Wyatt DAWN HELEOSII laser photometer for MALLS. The detector was operated at a wavelength of 658 nm. All SEC-MALLS measurements were made at ambient temperature.
HPLC analysis
The samples were loaded on a SuperdexTM 200 Increase 10/300 GL column (300 × 10 mm). The columns were equilibrated and eluted with 1M MgCl2 at the flow rate of 0.5 mL/min and an excitation wavelength of 280 nm. All volumes of the samples were 20 μL.
Oxygen affinity
Oxygen binding curve of native Hb was measured using a Hemox analyzer at 37 °C in PBS, pH 7.4. PolyDCLHb was measured on identical conditions, with 2-IT (2 mmol/L) and cross-linking agent (1 mmol/L) added. Triplicate measurements for each sample were performed to obtain the average value of P50, which was obtained from the oxygen equilibrium curves.
Results and discussion
SDS-PAGE
The SDS-PAGE analysis of purified Hb, DCLHb, DCLHb-SH, and polyDCLHb is shown in . Purified Hb migrated as a single band at 16 kDa, while unpurified DCLHb migrated as two primary bands at 16 (non-cross-linked Hb chains) and 32 kDa (Buehler et al. Citation2006). Finally, polyDCLHb migrated as a more complex mixture of species; there was a new band which indicated that a further intermolecular cross-linking was successful.
UV-Vis spectrum
Native Hb was used as a contrast. It is evident from that the absorption intensity of Hb before and after modification is not changed obviously. As we know, the absorption peak of heme is in the 415 nm. This demonstrated that there is no change in the spatial conformation around heme. Meanwhile, the absorption peaks of DCLHb-SH and polyDCLHb, which are at 540 nm and 577 nm, respectively (Hirsch et al. Citation1999), showed a good oxygenation state. This means that the modification of DCLHb has no obvious effects on the function of carrying oxygen.
Light scattering of studies
The SEC-MALLS spectrum of the solution of polyDCLHb is shown in . The MWs of main components and their corresponding peak of time are summarized in . Among these peaks, Peak 3 (from 20 min to 21.5 min) represents the tetramers of modified Hb. It was notable that there appeared a peak from18 min to 20 min (Peak 2), whose MW was ∼130.7 kDa. This peak indicates that there is a formation of polyDCLHb, which is cross-linked by two modified DCLHbs and one 1,6-BMH, so that the specific polymerization of DCLHb is feasible. Meanwhile, Peak 4 (from 21.5 min to 22.5 min) suggests the dissociation of unintramolecular Hb into dimers.
Table 1. MW distribution of polyDCLHb by SEC-MALLS.
HPLC analysis of polymerization
The HPLC spectra of purified Hb, DCLHb, and polyDCLHb are shown in . Due to the dissociation of unintramolecular Hb, there appeared only a single peak at about 21.7 min, which indicated the dimers of Hb (). After the cross-linking with DBBF, the MWs of DCLHb were increased, as a result, the corresponding peak at 20.7 min in HPLC demonstrated the existence of DCLHb (). When 1,6-BMH was used to further cross-link the DCLHb, the polymerizaiton gave a product mixture having a HPLC profile consisting of three peaks (). These peaks were identified as “tetrimers” (DCLHb), polyDCLHb, and “HMWs” of DCLHb that eluted in the excluded volume of the column. Among these peaks, the one which was in the 18.7 min corresponded to the polyDCLHb, and from the area of the peak, it could be concluded that polyDCLHb was up to about 20% of the total products (). This result confirmed that it was feasible to increase and control the MWs of polyDCLHb within a specific range by intermolecular cross-linking.
Figure 6. HPLC of the products. (a) purified Hb, (b) DCLHb, and (c) polyDCLHb mobile phase 1 mol/L MgCl2.
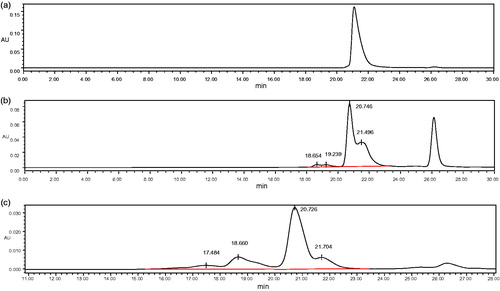
Table 2. The constituents and corresponding proportion of the chemical modified Hb.
Considering that the dosages of bromoacetic acid and 2-IT had influence on the control of MWs, we carried out a series of experiments in which the quantity of both reagents was varied to optimize the experimental condition. The results are listed in .
Table 3. The proportion of polyDCLHb in the polymerization of DCLHb with 1,6-BMH with varial quantity of bromoacetic acid and 2-IT.
When 3 molar equivalents of bromoacetic acid were used, the percentage of polyDCLHb in the total products was in the range of 14–15% (; Entry A1–A3). Nevertheless, using 2 molar equivalents of bromoacetic acid resulted in the increase of polyDCLHb up to the percentage of 17–18% (; Entry B1–B3). The increase of the desired polyDCLHb with less bromoacetic acid indicated that using more bromoacetic acid was adverse to the formation of polyDCLHb, which might hinder the conversion of amino group to thiol group. Hence the percentage of polyDCLHb decreased. In addition, the results of 3 molar equivalents of 2-IT were not as good as those of 2 molar equivalents of 2-IT. Excess of 2-IT might be expected to convert a small amount of polyDCLHb to “HMWs”, which consequently led to the light percentage decrease of polyDCLHb.
Oxygen affinity
The oxygen affinity (P50) (Benesch et al. Citation1982) of purified Hb and polyDCLHb are shown in and , respectively. Due to the lack of 2,3-DPG, purified Hb showed a high affinity of O2, whose P50 reduced to a lower value (10.88 mmHg) than native Hb (26 mmHg). However, after the cross-linking, the polyDCLHb showed a P50 value for 28.8 mmHg, which is similar to that of human blood (26 mmHg). The oxygen equilibrium curve indicated that polyDCLHb possessed favorable biological ability, which offers the benefits to carry and release O2.
Conclusion
The present study prepared a cross-linking agent, 1,6-BMH; then DCLHb was modified to produce DCLHb-SH and polymerized by 1,6-BMH to prepare polyDCLHb with proper MWs (∼130.7 kDa). Within this range of MWs, not only could polyDCLHb increase duration in the vascular circulation but also prevent aggregation/precipitation. Experimental data demonstrated the successful preparation of polyDCLHb and indicated its good oxygen affinity. This work put forward a new method to prepare chemical-modified polyDCLHb with proper MWs.
Disclosure statement
The authors report no declarations of interest. The authors alone are responsible for the content and writing of the paper.
Funding information
This work is supported by The National Science Foundation of China (No. 21202117/B020703) and The National High Technology Research and Development Program (“863” Program) of China (No. 2012AA021903).
References
- Benesch R, Benesch RE, Kwong S, Acharya AS, Manning JM. 1982. Labeling of hemoglobin with pyridoxal phosphate. J Biol Chem. 257:1320–1324.
- Bian YZ, Gao W, Chang TMS. 2013. Lowering of elevated tissue PCO2 in a hemorrhagic shock rat model after reinfusion of a novel nanobiotechnological polyhemoglobin-superoxide dismutase-catalase-carbonic anhydrase that is an oxygen and a carbon dioxide carrier with enhanced antioxidant properties. Artif Cells Nanomed Biotechnol. 41:60–68.
- Bolin R, DeVenuto F. 1983. Hemoglobin solution as a blood substitute. Adv Blood Res. 122:1–7.
- Buehler PW, Boykins RA, Norris S, Alayash AI. 2006. Chemical characterization of diaspirin cross-linked hemoglobin polymerized with poly(ethylene glycol). Anal Chem. 78:4634–4641.
- Buehler PW, D’Agnillo F, Schaer DJ. 2010. Hemoglobin-based oxygen carriers: from mechanisms of toxicity and clearance to rational drug design. Trends Mol Med. 16:447–457.
- Chang TMS. 2006. Blood substitutes based on nanobiotechnology. Trends Biotechnol. 24:372–377.
- Chatterjee R, Welty EV, Walder RY, Pruitt SL, Roger PH, Arnone A, Walder JA. 1986. Isolation and characterization of a new hemoglobin derivative cross-linked between the alpha chains (lysine 99 alpha 1—lysine 99 alpha 2). J Biol Chem. 261:9929–9937.
- Christa LM, Lewis LT, Victor P, Anirban SG. 2013. Synthetic approaches to RBC mimicry and oxygen carrier systems. Biomacromolecules. 14:939–948.
- Gao W, Bian YZ, Chang TMS. 2013. Novel nanodimension artificial red blood cells that act as O2 and CO2 carrier with enhanced antioxidant activity: PLA-PEG nanoencapsulated polySFHb-superoxide dismutase-catalase-carbonic anhydrase. Artif Cells Nanomed Biotechnol. 41:232–239.
- Hai TT, Pereira DE, Nelson DJ. 1998. Synthesis of water-soluble, nonimmunogenic polyamide cross-linking agents. Bioconjug Chem. 9:645–654.
- Haney CR, Buehler PW, Gulati A. 2005. Synthesis and characterization of a novel DTPA polymerized hemoglobin based oxygen carrier. Biochim Biophys Acta. 1725:358–369.
- Hirsch RE, Juszczak LJ, Fataliev NA, Friedman JM, Nagel RL. 1999. Solution-active structural alterations in liganded hemoglobins C(β6 Glu →Lys) and S (β6 Glu →Val). J Biol Chem. 274:13777–13782.
- Labrude P, Chaillot B, Dellacherie E, Stoltz JF, Vigneron C. 1986. Hemoglobin solutions as oxygen carriers: ligands and other molecules interactions with hemoglobin. Adv Biosci. 54:161–172.
- Laemmli UK. 1970. Cleavage of structural proteins during the assembly of the head of bacteriophage T4. Nature. 227:680–685.
- Pimenova T, Pereira CP, Schaer DJ, Zenobi R. 2009. Characterization of high molecular weight multimeric states of human haptoglobin and hemoglobin-based oxygen carriers by high-mass MALDI MS. J Sep Sci. 32:1224–1230.
- Rioux F, Drapeau G, Marceau F. 1995. Recombinant human hemoglobin (rHb1.1) selectively inhibits vasorelaxation elicited by nitric oxide donors in rabbit isolated aortic rings. J Cardiovasc Pharmacol. 25:587–594.
- Sehgal LR, Rosen AL, Gould SA, Sehgal HL, Moss GS. 1983. Preparation and in vitro characteristics of polymerized pyridoxylated hemoglobin. Transfusion. 23:158–162.
- Squires JE. 2002. Artificial blood. Science. 295:1002–1005.
- Walder JA, Zaugg RH, Walder RY, Steele JM, Klotz IM. 1979. Diaspirins that cross-Link beta chains of hemoglobin: bis(3,5-dibromosalicyl) succinate and bis(3,5-dibromosalicyl) fumarate. Biochemistry. 18:4265–4270.
- Walder RY, Andracki ME, Walder JA. 1994. Preparation of intramolecularly cross-linked hemoglobins. Methods Enzymol. 231:274–280.