Abstract
Background
It is a common medical practice to characterise an infection based on the causative agent and to adopt therapeutic and prevention strategies targeting the agent itself. However, from an epidemiological perspective, exposure to a microbe can be harmless to a host as a result of low-level exposure or due to host immune response, with opportunistic infection only occurring as a result of changes in the host, pathogen, or surrounding environment.
Methods
We have attempted to review systematically the key host, pathogen, and environmental factors that may significantly impact clinical outcomes of exposure to a pathogen, using Pseudomonas aeruginosa eye infection as a case study.
Results and discussion
Extended contact lens wearing and compromised hygiene may predispose users to microbial keratitis, which can be a severe and vision-threatening infection. P. aeruginosa has a wide array of virulence-associated genes and sensing systems to initiate and maintain cell populations at the corneal surface and beyond. We have adapted the well-known concept of the epidemiological triangle in combination with the classic risk assessment framework (hazard identification, characterisation, and exposure) to develop a conceptual pathway-based model that demonstrates the overlapping relationships between the host, the pathogen, and the environment; and to illustrate the key events in P. aeruginosa eye infection.
Conclusion
This strategy differs from traditional approaches that consider potential risk factors in isolation, and hopefully will aid the identification of data and models to inform preventive and therapeutic measures in addition to risk assessment. Furthermore, this may facilitate the identification of knowledge gaps to direct research in areas of greatest impact to avert or mitigate adverse outcomes of infection.
Infectious diseases are one of the most common public health issues and contribute significantly to loss of quality-adjusted life-years in those affected (Citation1). Their impacts range from minor, requiring no clinical intervention, to long-term disease, disability, or death. Infections may be local or systemic; primary or secondary; with acute, chronic, or latent presentation; they may also arise through the introduction of foreign bodies in otherwise healthy individuals. Infections may also be classified as sporadic, endemic, epidemic, or pandemic, and be communicable or non-communicable between individuals. As a consequence, a number of different health-related disciplines may be involved in their prevention and/or treatment.
Health professionals usually focus on specific segments of the infection, for example, the agent, the host, or the environment which together constitute the widely acknowledged epidemiological triangle which must converge to result in an adverse clinical outcome for the individual (Citation2). It is our view that a more integrated and holistic framework of the infection process is warranted potentially to provide a more effective means of tackling infection prevention and treatment. Here, we propose a framework that incorporates elements of the recently described International Life Sciences Institute Key Events Dose–Response Framework (Citation3), which has been applied as a case study to microbiological hazards (Citation4).
We propose a proof-of-concept model for the identification of key events in the infection process of the opportunist bacterium, Pseudomonas aeruginosa, specifically in the context of eye infections. This organism is the primary cause of microbial keratitis (corneal infection) in users of extended-wear contact lenses and is usually associated with specific lens types as well as compromised hygiene that may lead to contamination of lens wash solutions (Citation5). We have used this infection model as a case study, primarily because the aetiological agent is an opportunist, and therefore, susceptibility of the host is key in establishing infection. In addition, there is good supportive scientific literature in this area. It is our hope that the model will give a better understanding of the infection process in microbial keratitis, and potentially identify key target areas for preventive or therapeutic control measures, as well as inform the development of risk assessment strategies. Indeed, we have adopted the basic concepts of the classic risk assessment framework (exposure, hazard identification, and characterisation) to structure the proposed model (Citation6).
The pathway-based concept describes the process in a sequential form (). It starts from the initial exposure of a host to an infectious agent, taking into account the number of microorganisms that manage to bind to specific mucosal receptors (the molecular initiating event) that will lead to a number of chain reactions (pathway of adverse outcome) and to the overall result, which is the clinically adverse outcome. It is worth noting that there are two processes mapped acting in opposite directions. The first process reflects the deleterious effects that the pathogen will have on the host, while the second describes the defences of the host used to inhibit or neutralise its progress. This concept is also mapped under the following classical empirical exponential dose–response model1
Fig. 1. Pathway-based conceptual model of the infection process. We have used the following colour code to map the different components of the epidemiological triangle, colour coding the adverse outcome (intersection) separately: orange: exposure (environment and interactions); blue: the invasion process by the microorganism; red: host defences based on time of occurrence; and green: adverse outcome (level of infection).
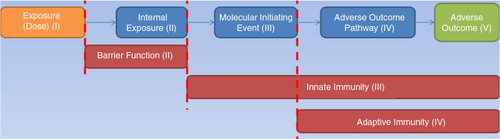
where ‘p’ is the probability of a specific endpoint (infection, illness, or death) as a function of two parameters: 1) parameter ‘d’ represents the dose to which the host is exposed while, 2) parameter ‘k’ is an indicator of the probability of a single organism surviving within the host. The exponential dose–response model assumes that one organism is capable of causing infection if it arrives at an appropriate site. More complex but still empirical models, are described in (Citation7) which target the variability of the agent and the host, and sometimes the effect of the environment. Although these models take into account some or most of these principles and are in general agreement with the proposed concept, our aim is to assist in moving away from such empirical models towards developing more mechanistic approaches that open and explore the ‘black box’ of infection.
In this paper, we review the known risk factors and stages in the infectious process of P. aeruginosa in the eye grouped by key events () and attempt to demonstrate the value of examining this process in a holistic framework as opposed to each factor in isolation. We propose that such an approach could form the basis of a generic model applicable to other infectious processes. Hereinafter, this paper follows the categorisation of key events presented in to describe the proposed framework.
Table 1 Stages in infectious process and predisposing risk factors
Exposure (dose)
Exposure refers to the number of microorganisms that reach the host before any further action occurs. In terms of a product contaminated with P. aeruginosa, the dose in its simplest form can be calculated by the number of bacteria per ml of product multiplied by the quantity of product used. This, however, is not always a simple calculation, due to different consumer habits during product use and whether the product is intended to be used as a leave-on or rinse-off agent. Exposure may also arise through contact with other sources such as the use of other products (e.g. soaps) contaminated with the organism. In terms of contact lenses, an estimation has to be made of how many microorganisms are transferred from the contaminated wash solution to the lens itself in order to estimate the level of exposure of the eye to the microbe. A number of factors with regard to the product (environment) or the user (host) can affect the presence of the pathogen on the lens and the level of exposure of the host. Because of this complexity, the safety/risk of the host is often assessed using a worst-case scenario following a binomial approach (‘yes/no’ type of response), which serves to safeguard both products and consumers by being overly conservative.
Conventional contact lenses fall into two basic categories with regard to material and composition: soft lenses and rigid gas-permeable lenses. Socio-economic factors or personal choice may affect the type of lens worn by a consumer. Contact lenses are worn by about 5% of the general public in developed countries and soft hydrogel lenses account for about two-thirds of the market (Citation8). Soft lenses are classified by the US Food and Drug Administration as being materials of high (>50%) or low (<50%) water content. Two main types – conventional hydrogel or silicone gel (several varieties) – are retailed. Lenses are also categorised for daily wear, or extended wear (6–30 nights) depending on the lens material (www.medscape.com/view article/773026).
A systematic review of the literature prior to 2011 by Dutta et al. (Citation9) reported that the incidence of microbial keratitis associated with contact lens wear varied from 12 to 66% depending on the study design and location. The annualised incidence of keratitis ranged from 9.2 to 20.9 per 10,000 individuals for overnight wear of lenses and 2.2 to 3.5 for daily wear. Based on a similar denominator of contact lens wearers, a prospective questionnaire study in Australia (Citation5) found an annual incidence of 1.2 cases per 10,000 of contact lens related microbial keratitis, and among a subset of culture proven infections the highest rates were found for daily wearers of silicone hydrogel lenses (11.9/10,000), overnight wear soft silicone (19.5), and overnight wear silicone hydrogel (25.4) lenses. Lowest rates were observed for daily wear soft (1.9) and daily disposable lenses (2.0). Loss of vision occurred in 0.6/10,000 wearers.
A number of independent risk factors have been identified for microbial keratitis but extended – particularly overnight – contact lens wear, tropical climate environment, and poor storage case hygiene remain the most commonly cited (Citation10). Significantly, dense biofilms of microbial communities growing in contaminated contact lens storage cases in the presence of disinfectants in an experimental setting have been demonstrated; these biofilms have proved difficult to remove by standard lens care practice such as rinsing in hot water or in multipurpose solution containing disinfectants (Citation11). Nevertheless, the contribution of user hygiene to the risk of developing keratitis is the subject of some debate. Various aspects of good user practice such as hand washing, frequency of disinfection, replacement rather than ‘topping up’ of disinfectant, and efficacy of the disinfectant have all been cited in several epidemiological studies and together or individually contribute to risk reduction (Citation12). Extended contact lens wear has been shown to cause migration of Langerhans cells into the central cornea (Citation13). These are key sentinel cells of the cornea which recognise, process, and present antigens to the immune system. This leads to cytokine release and further influx of inflammatory cells, particularly CD4+ Th1 cells which maintain a neutrophil infiltrate in Pseudomonas keratitis (Citation14).
P. aeruginosa is a common contaminant of contact lens wash solutions partly due to its innate and acquired resistance to contact lens biocides (Citation15). The composition of these solutions is complex and varies with manufacturer but chlorhexidine and benzalkonium chloride are universally used for rigid gas-permeable lenses. Hydrogel lenses are most widely used today and disinfectant solutions of polyaminopropyl biguanide in a borate base, and polyquaternium-1 in a citrate base, among others, have been used as preservatives. However, susceptibility to disinfectants is not uniform for all strains and some may express full resistance to agents and even multiply above the initial challenge inoculum over 24–48 h exposure to the disinfectant (Citation15). More significantly, this latter study found that resistance of strains was correlated with acute cytotoxic activity on corneal epithelial cells in culture and linked to the presence of a regulatory cytotoxicity gene, suggesting that the disinfectant may select for contamination with cytotoxic strains. Resistance to quaternary ammonium compounds also appears to be a function of the surface hydrophobicity of a strain as electrostatic repulsion between bacterial surface cations and the antimicrobial agent impede the entry of the latter into the cell (Citation16).
Some evidence suggests that although markedly strain dependant, the increased cell surface hydrophobicity of P. aeruginosa contributes to its significantly greater adhesion to soft lens types (Citation17, Citation18). These lenses also vary in their surface hydrophobicity. Maximal adhesion of P. aeruginosa in stationary phase culture to worn lenses was achieved with approximately 1×109 cfu/ml (Citation19) and adherence occurred as rapidly as 5 min after exposure and peaked at 2–3 h (Citation20). Biofilm – glycocalyx – formation on hydrogel lenses was reported to occur after 30 min with an inoculum of approximately 107 organisms and form a mature biofilm within 24 h (Citation21). Furthermore, recent evidence from an experimental mouse corneal infection model with P. aeruginosa has demonstrated that although the bacteria are in a planktonic mode of growth in the early stages of infection, the early stages of biofilm formation and slight opacity were evident on the corneal surface after 24 h of exposure to 1×108 cfu/ml of the challenge strain (Citation22). These biofilms develop into three dimensional structures which vary in density depending on the substrate material of the contact lens and achieve maturity within 72 h with cell aggregates and microcolonies embedded in an extracellular polymer on all lens materials (Citation23). The organisation of the biofilm results in gradients of oxygen, pH, and nutrients and facilitates intercell communication (quorum sensing) and gene transfer between cells which together enhance the virulence properties of the bacteria.
Several studies have linked the degree of adherence of P. aeruginosa and other bacteria to water content of contact lenses but this is not consistently supported in the literature (Citation9). Other factors such as pH and electrolyte concentration may also influence adherence of the species to lens materials (Citation24).
There is some evidence to suggest that adaptation of some P. aeruginosa strains to environmental water may be a key factor in their association with keratitis. The great majority of keratitis isolates (71%) in a large series from UK patients were grouped together in a core genotype cluster which remained stable over time and was related to a clonal complex more commonly associated with environmental water sources (Citation25). It is arguable that the adaptation and high prevalence of the clonal cluster in environmental waters could be a key factor that results in higher exposure and consequently higher association of the clone with keratitis. On the other hand, a specialised population of P. aeruginosa from the natural aqueous environment which seems to have a propensity to cause corneal infection may be positively selected by contact lens wearers. It remains unknown whether these strain populations result in more severe infections than strains outside of the core cluster. If so, then the identification of the strain genotype of P. aeruginosa isolates from keratitis or contact lens solutions may be informative of the likely course and outcome of the infection.
Internal exposure/barrier function impairment
For most opportunist species whether an organism remains a benign colonist of a body site or progresses to establish an infection in that site depends largely on the balance of the host defences and on the armoury of pathogenicity factors at the organism's disposal. Skin and mucous membranes present an anatomical barrier against invasion and infection of the host by the microbe. Several properties including the presence of fatty acids, low pH, antimicrobial peptides, and enzymes all contribute to this process (Citation26). The ocular surface is exposed to the external environment and is open to atmospheric pollutants, multiple antigens, and microbes. To establish an ocular infection P. aeruginosa needs to traverse the ocular barrier in order to bind with relevant receptor targets to reach its pathogenic potential. In the case of contact-lens-associated keratitis, planktonic and biofilm-derived bacteria on the lens come into intimate contact with the corneal surface.
For Pseudomonas keratitis to develop, both microbial virulence characteristics and the physiological impact of contact lens wear on the cornea come into play (Citation26). A number of host factors may contribute to increased susceptibility to infection and include inhibition of normal corneal epithelial cell shedding, thinning of the epithelia, ocular trauma, and increased bacterial binding to specific receptors on exposed corneal membranes, among others as detailed by Stapleton and Carnt (Citation10). P. aeruginosa is able to take advantage of the hypoxia and local trauma of the cornea and intrude to the host. A combination of secreted virulence factors and host immune and inflammatory responses contribute to host tissue damage and to the elimination of the bacteria.
Molecular initiating event/innate immunity
When the pathogen manages to pass the ocular barrier, it is exposed to the innate immunity mechanisms of the host. Binding to the host receptors is considered as the first step of a series of biological events, which dictate the pathogenic potential of the microorganism.
To establish a population of P. aeruginosa cells on an abiotic or biotic surface, the flagella of planktonic cells provide the ‘swimming’ mobility necessary to bring them into close contact with the surface. Polar positioned pili (Type IV pili) promote cell movement through a form of ‘twitching’ motility by tethering the bacteria to a specific cell surface, which on retraction, effectively drags the bacterial cell forward. Motility may be influenced by the viscosity of the surrounding medium as indicated by the recent observation of increased surface motility in the presence of mucin (Citation27). This is distinct from ‘swarming’ motility on semi-solid surfaces. Both these mechanisms are also implicated in Pseudomonas biofilm formation. As with some other Gram-negative bacteria, P. aeruginosa is able to form fimbriae on the bacterial cell surface using a system called the chaperone/usher pathway (CUP). The CUP proteins are structurally similar to immunoglobulins and escort the intracellular subunits of the fimbrial proteins to an usher protein complex, which facilitates the translocation and assembly of these subunits across the outer membrane. At least three different CUP (A, B, and C) systems have been identified in the P. aeruginosa reference strain PAO1, and CUP A is required for the successful formation of biofilm on abiotic surfaces (Citation28) through aggregation of the cells in microcolonies (Citation29).
A variety of transmembrane proteins, the Toll-like receptors (TLRs), and Nod-like receptors recognise and interact with specific ligands on the bacterial surface. These activated molecules stimulate macrophages or mast cells leading to the synthesis and secretion of pro-inflammatory cytokines and recruitment of phagocytes which engulf and kill the organism by the release of toxic reactive oxygen species (Citation30). At least 13 different TLRs have been identified in man and mice (Citation31) but TLRs 2, 4, and 5 recognise, respectively, pilin protein (Citation32), lipopolysaccharide (LPS) (Citation33), and flagellin protein (Citation34).
The immune system of the ocular mucosa uses both innate and adaptive effector mechanisms present in the tissue and tear film (Citation35). These serve to destroy the invading pathogen and limit the harmful effects of the inflammation on the eye. Leucocytes, primarily lymphocytes, and lymphoid cells are normal non-inflammatory components of the ocular surface and express in the main CD8+ suppressor/cytotoxic T cells. Plasma cells produce IgA and secretory SIgA on the conjunctival surface and the tear film which is also rich in antibacterial proteins. Chief of these are lysozyme, which destroys the bacterial cell wall, lactoferrin which binds iron essential for bacterial growth, and lipocalin which scavenges bacterial products (Citation35).
Surfactant protein D has been shown to inhibit epithelial cell invasion by P. aeruginosa (Citation36). Several other antimicrobial and effector molecules have been identified in tear film including leucocyte protease inhibitor, interleukins, macrophage inhibitory proteins, and different TLRs. The reader is referred to an excellent discussion of the role of TLRs in the infectious process in the eye by Pearlman et al. (Citation37). The authors propose that both live bacteria and their products activate TLRs in the cornea leading to chemokine production and neutrophil recruitment. Although the latter cells are necessary for bacterial killing, they can also cause loss of clarity of the cornea and so targeting of the TLR pathway as a means of treating such infections may be effective. Furthermore, they suggest that given the role of P. aeruginosa type III secretion system (T3SS) in the induction of neutrophil apoptosis and promoting growth of the pathogen in the cornea, therapy could also be targeted at the exotoxins associated with this secretion system. The corneal mucosa also contains a specific subset of mucin glycoproteins which protect the eye from invading microbes and, in particular, bind to P. aeruginosa (Citation38). Recent work suggests that commonly used formulations of contact lens solution may destroy the mucin layer and contribute to increased infections of the cornea (Citation39).
Adverse outcome pathway/adaptive immunity
Despite being endowed with a considerable array of virulence-associated genes, most strains of P. aeruginosa have low intrinsic virulence for humans. Genes for several virulence factors (cell-associated and secreted) have been identified in P. aeruginosa located on plasmids and the chromosome. Examples, among others, are genes that encode adhesion molecules, motility, cell-to-cell communication, exotoxins, exopolysaccharide biosynthesis, secreted exoenzymes, phenazine pigment, and a variety of regulatory proteins (Citation40). The contribution of these and other factors to the establishment of P. aeruginosa infections varies with the strain type and the susceptibility of the individuals, but common features are the need for the organism to 1) adhere to the host tissue and multiply to significant numbers, 2) breach innate defences, 3) exert a cytotoxic effect, and 4) resist the action of adaptive immune response and antibacterials, ultimately to persist in a hostile environment.
The major virulence factors of twitching motility (type IV pili), motility (flagella), exoprotein secretion systems, and quorum sensing controlled exoproducts have all been implicated in the pathogenesis of P. aeruginosa keratitis but evidence suggests that some strain populations with specific virulence gene profiles are more likely to be recovered from keratitis than others (Citation41). Stewart et al. (Citation42) found in a large series of P. aeruginosa isolates from keratitis and other clinical sources that genes significantly more prevalent in keratitis strains included oriC (replication origin), exoU (exotoxin), and katN (non-heme catalase). ExoU and ExoS are part of a T3SS and, depending on their presence, strains may exhibit different physiological and pathogenic characteristics, such as protease production and biofilm formation (Citation43). Bacteria secrete a number of biologically active exoproteins through type secretion systems in response to their environment or to promote survival in the host. Many of these proteins are toxins or hydrolytic enzymes involved in pathogenesis. To date, six different classes of these systems have been described in bacteria and to our knowledge all but one, T4SS, has been identified in P. aeruginosa (Citation44). The T3SS is the most intensively studied in Gram-negative bacterial pathogenesis as it promotes the injection of toxins directly into the cytoplasm of host cells. The apparent selection of strains expressing ExoU indicates that cytotoxicity is a key factor in the establishment of Pseudomonas keratitis. It has recently been shown that an active phospholipase domain of ExoU is required for the traversal of corneal epithelia by P. aeruginosa (Citation45). The T2SS-dependent release of proteolytic enzymes plays a significant role in corneal damage and degradation of secretory immunoglobulin A in Pseudomonas keratitis (Citation46). Other enzymes secreted through T2SS include lipases and phospholipases, and alkaline phosphatase, all of which act on extracellular targets.
Adverse outcome (infection)
The final event of the proposed framework is the adverse outcome. The ‘endpoint’ (response) discussed in this paper is infection, which is generally defined as a condition in which a pathogen invades and can actively multiply within the body of the host. Both subclinical and clinically apparent infections are possible (i.e. depending on the manifestation of symptoms). As presented in , there can be four possible outcomes resulting from infection as an adverse outcome to the proposed framework, namely: resolved, mild, moderate, and severe.
Discussion
Fleiszig and Evans (Citation47) proposed a four-step model of the pathogenesis of P. aeruginosa keratitis in contact lens wearers with an undamaged cornea. First, the physical presence of the lens interferes with clearance of the bacteria which in turn bind and form biofilm on the lens to promote their survival from where they interact and adhere to corneal epithelia. Second, those bacteria that evade the TLRs and resist local defensin antimicrobials traverse the multilayered epithelia through breakdown of cell-to-cell junctions and extracellular matrices. Third, the bacteria cross the physical barrier of the epithelial basement membrane, whose small pore sizes normally exclude invading organisms, through production of general and specific proteases which degrade the matrix glycoproteins (Citation48). Finally, the bacteria enter the corneal stroma and initiate inflammatory and immune response cascades, which produce the visible pathology.
Our proposed framework incorporates and expands the model of Fleiszig and Evans (Citation47) by including the exposure assessment, which details the primary exposure of the individual to the organism, and provides additional information pertinent to characterising the output of the infection as resolved, mild, moderate, or severe. Therefore, where Fleiszig and Evans (Citation47) model solely the events in pathogenesis, we have attempted to account for the effect of these events on the adverse outcome. The addition of other parameters renders our proposed framework rather more generic than specific to pathogenesis and extends its adaptability to other organisms and infection types.
The use of contact lenses is clearly a dominant factor as P. aeruginosa keratitis is rare in non-users. Poor lens hygiene and extended wear are clearly contributory factors to increased susceptibility to infection.
However, it appears that the presence of the organism on the corneal surface alone is not sufficient for infection to occur and several factors are necessary for the bacteria to penetrate the cornea and expose the internal subepithelial cells. The numbers of organisms that traverse the ocular barrier are considered representative of the ‘internal exposure’, as they are able to bind to cells and express their cytotoxic potential. This process also applies to the skin where sweat and sebum production, abnormalities in rate of corneocyte replacement, thinning of the skin layers, or hypoxia, all contribute as risk factors compromising skin barrier function (Citation49).
The molecular initiating event in the case of keratitis is the binding of the bacteria to surface receptors of the subepithelial cells mediated through various structures and surface expressed molecules. Once adherent to the stroma, cytotoxic molecules are orchestrated through quorum sensing and exoprotein secretion systems. Although these factors are recognised by the innate immune system, the relative number of microbial versus innate immunity molecules (competitive binding) is the main determinant of the outcome of the infectious process. If only a small number of P. aeruginosa traverse the ocular barrier, it is likely that innate immune factors will be sufficient to inhibit the infectious process leading to a resolved or mild adverse outcome.
Determination of the minimum number of P. aeruginosa cells required to initiate infection is complicated because of its opportunist nature whereby some degree of predisposition of the host is necessary. It has been established in rabbit corneal infection models that the minimum infectious dose for keratitis is of the order of 100–1,000 P. aeruginosa if injected directly into the stroma (Citation50). If the ocular barrier is breached and/or innate immunity is dysfunctional, then according to the theory of the single-hit model (Citation51), a single microorganism could reach its target and cause an infection. Recent evidence, using omics data from HIV infections, has demonstrated that the infection arises from a single variant, or a few viral particles (Citation52). In reality, barriers function efficiently to impede the spread of bacteria and viruses and reduce the numbers reaching the target, thus a higher infectious dose may be required to cause an adverse outcome. However, as populations of P. aeruginosa adhering to experimentally contaminated contact lenses can reach numbers of 107–109 cfu/ml on the lens surface within 45 min (Citation20, Citation21), the bacterial load could far exceed the ‘minimum infectious dose’ of 100–1,000 cfu as established from animal studies (Citation50, Citation53) and thus overwhelm the ocular barrier function and host defences.
In the context of risk assessment, the interactions between host and pathogen, influenced by environmental factors, present a challenge at the hazard characterisation step (i.e. dose–response assessment). In its simplest form, Equation (Citation1) describes this mathematically; the lower the value of k (probability of agent surviving the host defences), the higher the dose d required for the microorganism to cause an adverse outcome. Clearly, such an approach is a continuum, rather than a specific cut-off or ‘tipping point’ and this will vary with each individual according to their susceptibility, giving different probabilities of developing an infection. The concurrent circumstances and risk factors of the host such as ocular hygiene, type of contact lens, and pattern of wear, all contribute to infection risk at the time of exposure. Therefore, understanding the underlying biology of infection and the variability of interactions between pathogens and hosts offer a promising strategy to refine the hazard characterisation step in risk assessment.
Characterisation of the risk to the host is problematic as the degree of host response to the pathogen is highly variable. Preconditions in the host may reduce local defence mechanisms thus allowing relatively low bacterial numbers to invade and establish an infection. The adaptive immune response is the last line of defence and if activated, may prevent severe infection. Nevertheless, it should be borne in mind that overreaction of the immune system in response to the agent may increase inflammation and result in exacerbation of clinical symptoms.
The categories of adverse outcome as outlined earlier are dependent on the standpoint of the user of this framework as different protection goals can be set, for example, preventive measures should have targets of none or resolution of infection, whereas therapeutic measures will primarily impact on the reduction of infection severity. Epidemiological studies can help to identify the key factors of an infection and to propose appropriate control measures. However, on occasion, such measures may not impact directly on the pathogen per se, but serve to strengthen susceptible hosts or to reduce transmission in the environment, for example, hygiene and sanitation. A more structured approach to identify protection goals by identifying at-risk groups, for example, contact lens users, and adopting risk mitigation measures may be more effective to avoid or minimise unintentional exposure to microorganisms.
Conclusions
We propose a new conceptual pathway based model to describe the mechanism of infection that can be used by health professionals and risk assessors of contamination of personal care products used by the general public, using P. aeruginosa keratitis as a case study. By taking into account the host, agent, environment, and their interactions, it is possible to identify the key events in the process of infection and allow focus of effort on those that have a critical effect on the potential outcome. Despite the specificity of the case model to contact-lens-associated infection the model can theoretically be applied to other infections of differing severity. From a therapeutic perspective, it is expected that the investigator will focus more on the identification of the pathogen and ways to limit the development of the infection, whereas epidemiological investigations target the transmission of a disease, including the environment, and potential key host factors. From a safety/risk assessment perspective, the focus would be on evaluating the likelihood of adverse human health effects occurring, following exposure to the pathogen, and managing the risk by ensuring that products meet the required safety objectives by the use of relevant control measures, for example, non-favourable growth conditions or antimicrobials in lens solutions.
The proposed model is structured to allow the identification of knowledge gaps and highlight areas where further research is required to sketch a more complete picture of the infection process for a given scenario. It is hoped that this model will have the potential to focus research efforts and aid a better understanding of risk assessment and management of the infection process and associated outcomes.
Conflicts of interest and funding
The work was funded from an internal budget for Unilever staff members and Dr. Tyrone Pitt was contracted as an external consultant funded by Unilever.
References
- Sassi F. Calculating QALYs, comparing QALY and DALY calculations. Health Policy Plan. 2006; 21: 402–8.
- Last JM. A dictionary of epidemiology. 2001; New York: Oxford University Press. 196. 4th ed.
- Julien E, Boobis RA, Olin SS, The ILSI Research Foundation Threshold Working Group . The Key Events Dose–Response Framework: a cross-disciplinary mode-of-action based approach to examining dose-response and thresholds. Crit Rev Food Sci Nutr. 2009; 49: 682–9. [PubMed Abstract] [PubMed CentralFull Text].
- Buchanan LR, Havelaar HA, Smith AM, Whiting CR, Julien E. The Key Events Dose–Response Framework: its potential for application to foodborne pathogenic microorganisms. Crit Rev Food Sci Nutr. 2009; 49: 718–28.
- Stapleton F, Keah L, Edwards K, Naduvilath T, Dart JK, Brian G, etal. The incidence of contact lens related microbial keratitis in Australia. Ophthalmology. 2008; 115: 1665–72.
- Haas CN, Rode JB, Gerba CP. Quantitative microbial risk assessment. 2014; 2nd ed, New Jersey: John Wiley & Sons. 63–89.
- Haas CN. Microbial dose–response modeling: past, present & future. Environ Sci Technol. 2015; 49: 1245–59. doi: http://dx.doi.org/10.1021/es504422q [PubMed Abstract].
- Edwards K, Keay L, Naduvilath T, Stapleton F. A population survey of the penetrance of contact lens wear in Australia: rationale, methodology and results. Ophthalmol Epidemiol. 2009; 16: 275–80.
- Dutta D, Cole N, Willcox MD. Factors influencing bacterial adhesion to contact lenses. Mol Vis. 2012; 18: 14–21.
- Stapleton F, Carnt N. Contact-lens related microbial keratitis: how have epidemiology and genetics helped us with pathogenesis and prophylaxis. Eye. 2012; 26: 185–93.
- Vijay AK, Willcox M, Zhu H, Stapleton F. Contact lens storage case hygiene practice and storage case contamination. Eye Contact Lens. 2015; 41: 91–7.
- Carnt N, Willcox MDP, Keay L, Flanagan J, Stapleton F. Pathogenesis of contact-lens associated microbial keratitis. Optom Vis Sci. 2010; 87: 612–14.
- Hazlett LD, McClellan SM, Hume SB, Dajcs JJ, O'Callaghan RJ, Willcox MD. Extended contact lens usage induces Langerhans cell migration into cornea. Exp Eye Res. 1999; 69: 575–7.
- Hazlett LD. Pathogenic mechanisms of P. aeruginosa keratitis: a review of the role of T cells, Langerhans cells, PMN, and cytokines. DNA Cell Biol. 2002; 21: 383–90.
- Lakkis C, Fleiszig SMJ. Resistance of Pseudomonas aeruginosa isolates to hydrogel contact lens disinfection correlates with cytotoxic activity. J Clin Microbiol. 2001; 39: 1477–86.
- Bruinsma GM, Rustema-Abbing M, van der Mei HC, Lakkis C, Busscher HJ. Resistance to a polyquaternium-1 care solution and isoelectric points of Pseudomonas aeruginosa strains. J Antimicrob Chemother. 2006; 57: 764–6.
- Bruinsma GM, van der Mei HC, Busscher HJ. Bacterial adhesion to surface hydrophilic and hydrophobic contact lenses. Biomaterials. 2001; 22: 3217–24.
- Henriques M, Sousa C, Lira M, Elisabete M, Oliveira R, Oliveira R, etal. Adhesion of Pseudomonas aeruginosa and Staphylococcus epidermidis to silicone-hydrogel contact lenses. Optom Vis Sci. 2005; 82: 446–50.
- Williams TJ, Schneider RP, Willcox MD. The effect of protein-coated contact lenses on the adhesion and viability of gram negative bacteria. Curr Eye Res. 2003; 27: 227–35.
- George M, Adhearn D, Pierce G, Gabriel M. Interactions of Pseudomonas aeruginosa and Staphylococcus epidermidis in adhesion to a hydrogel. Eye Contact Lens. 2003; 29: S105–9.
- Stapleton FDJ, Matheson M, Woodward E. Bacterial adherence and glycocalyx formation on unworn hydrogel lenses. J Br Contact Lens Assoc. 1993; 16: 113–16.
- Saraswathi P, Beuerman RW. Corneal biofilms: from planktonic to microcolony formation in an experimental keratitis infection with Pseudomonas aeruginosa. Ocul Surf. 2015; 13: 331–45. doi: http://dx.doi.org/10.1016/j.tos.2015.07.001 [PubMed Abstract].
- Randler C, Matthes R, McBain AJ, Giese B, Fraunholz M, Sietmann R, etal. A three-phase in-vitro system for studying Pseudomonas aeruginosa adhesion and biofilm formation upon hydrogel contact lenses. BMC Microbiol. 2010; 10: 282. doi: http://dx.doi.org/10.1186/1471-2180-10-282 [PubMed Abstract] [PubMed CentralFull Text].
- Miller MJ, Ahearn DG. Adherence of Pseudomonas aeruginosa to hydrophilic contact lenses and other substrata. J Clin Microbiol. 1987; 25: 1392–7. [PubMed Abstract] [PubMed CentralFull Text].
- Shankar J, Sueke H, Wiehlmann L, Horsburgh MJ, Tuft S, Neal TJ, etal. Genotypic analysis of UK keratitis-associated Pseudomonas aeruginosa suggests adaptation to environmental water as a key component in the development of eye infections. FEMS Microbiol Lett. 2012; 334: 79–86.
- Hornef MW, Wick MJ, Rhen M, Normark S. Bacterial strategies for overcoming host innate and adaptive immune responses. Nat Immunol. 2002; 3: 1033–40.
- Yeung AT, Parayno A, Hancock RE. Mucin promotes rapid surface motility in Pseudomonas aeruginosa. mBio. 2012; 3 pii: e00073–12. doi: http://dx.doi.org/10.1128/mBio.00073-12.
- Vallet I, Diggle SP, Stacey RE, Camara M, Ventre I, Lory S, etal. Biofilm formation in Pseudomonas aeruginosa: fimbrial cup gene clusters are controlled by the transcriptional regulator MvaT. J Bacteriol. 2004; 186: 2880–90.
- Sriramulu DD, Lünsdorf H, Lam JS, Römling U. Microcolony formation: a novel biofilm model of Pseudomonas aeruginosa for the cystic fibrosis lung. J Med Microbiol. 2005; 54: 667–76.
- Akira S, Uematsu S, Takeuchi O. Pathogen recognition and innate immunity. Cell. 2006; 124: 783–801.
- Kawasaki T, Kawai T. Toll-like receptor signalling pathways. Front Microbiol. 2014; 5: 461.
- Lorenz DA, Chemotti DC, Vandal K, Tessier PA. Toll-like receptor 2 represses nonpilus adhesin-induced signalling in acute infections with the Pseudomonas aeruginosa pilA mutant. Infect Immun. 2004; 72: 4561–9.
- Pier GB. Pseudomonas aeruginosa lipopolysaccharide: a major virulence factor, initiator of inflammation and target for effective immunity. Int J Med Microbiol. 2007; 297: 277–95.
- Feuillet V, Medjane S, Mondor, Demaria I, Pagni I, Galán JE, etal. Involvement of Toll-like receptor 5 in the recognition of flagellated bacteria, Proc Nat Acad Sci USA. 2006; 103: 12487–92. [PubMed Abstract].
- Knop E, Knop N. Niederkorn JY, Kaplan HJ. Anatomy and immunology of the ocular surface. Immune response and the eye. Chemical immunology and allergy . 2007; 92; Basel: Karger. 36–49.
- Ni M, Evans DJ, Hawgood S, Anders EM, Sack RA, Fleiszig SM. Surfactant protein D is present in human tear fluid and the cornea and inhibits epithelial cell invasion by Pseudomonas aeruginosa. Infect Immun. 2005; 73: 2147–56.
- Pearlman E, Sun Y, Roy S, Karmakar M, Hise AG, Szczothaa-Flynn L. Host defense at the ocular surface. Int Rev Immunol. 2013; 32: 4–18.
- McAuley JL, Linden SK, Png CW, King RM, Pennington HL, Gendler SJ, etal. MUC1 cell surface mucin is a critical element of the mucosal barrier to infection. J Clin Invest. 2007; 117: 2313–24.
- Gordon GM, Moradshabi N, Jeong S, Lane C, Fini ME. A novel mechanism of increased infections in contact lens wearers. Invest Ophthalmol Vis Sci. 2011; 52: 9188–94.
- Lyczak JB, Cannon CL, Pier GB. Establishment of Pseudomonas aeruginosa infection: lessons from a versatile opportunist. Microb Infect. 2000; 2: 1051–60.
- Lomholt JA, Poulsen K, Kilian M. Epidemic population structure of Pseudomonas aeruginosa: evidence for a clone that is pathogenic to the eye and that has a distinct combination of virulence factors. Infect Immun. 2001; 69: 6284–95.
- Stewart RMK, Wiehlmann L, Ashelford KE, Preston SJ, Frimmersdorf E, Campbell BJ, etal. Genetic characterization indicates that a specific subpopulation of Pseudomonas aeruginosa is associated with keratitis infections. J Clin Microbiol. 2011; 49: 993–1003.
- Choy HM, Stapleton F, Willcox PDM, Zhu H. Comparison of virulence factors in Pseudomonas aeruginosa strains isolated from contact lens- and non-contact lens-related keratitis. J Med Microbiol. 2008; 57: 1539–46.
- Bleves S, Viarre V, Salacha R, Michel GPF, Filloux A, Voulhoux R. Protein secretion systems in Pseudomonas aeruginosa: a wealth of pathogenic weapons. Int J Med Microbiol. 2010; 300: 534–43.
- Ramirez JC, Fleiszig SM, Sullivan AB, Tam C, Borazjani R, Evans DJ. Traversal of multilayered corneal epithelia by cytotoxic Pseudomonas aeruginosa requires the phospholiase domain of exoU. Invest Ophthalmol Vis Sci. 2012; 53: 448–53.
- Lomholt JA, Kilian M. Degradation of uniquely glycosylated secretory immunoglobulin A in tears from patients with Pseudomonas aeruginosa keratitis. Invest Ophthalmol Vis Sci. 2008; 49: 4939–44.
- Fleiszig SM, Evans DJ. Pathogenesis of contact lens-associated microbial keratitis. Optom Vis Sci. 2010; 87: 225–32.
- Alarcon I, Kwan L, Yu C, Evans DJ, Fleiszig SM. Role of corneal epithelial basement membrane in ocular defense against Pseudomonas aeruginosa. Infect Immun. 2009; 77: 3264–71.
- Wu DC, Chan WW, Metelitsa AI, Fiorillo L, Lin AN. Pseudomonas skin infection: clinical features, epidemiology, and management. Am J Clin Dermatol. 2011; 12: 157–69.
- Moreau JM, Conerly LL, Hume EB, Dajcs JJ, Girgis DO, Cannon BM. Effectiveness of mupirocin and polymyxin B in experimental Staphylococcus aureus, Pseudomonas aeruginosa, and Serratia marcescens keratitis. Cornea. 2002; 21: 807–11.
- Teunis PFM, Havelaar AH. The beta Poisson dose–response model is not a single-hit model. Risk Anal. 2000; 20: 513–20.
- Gale P, Hill A, Kelly L, Bassett J, McClure P, Le Marc Y, Soumpasis I. Applications of omics approaches to the development of microbiological risk assessment using RNA virus dose–response models as a case study. J Appl Microbiol. 2014; 117: 1537–48.
- Tam C, Mun JJ, Evans DJ, Fleizig SMJ. The impact of inoculation parameters on the pathogenesis of contact lens-related infectious keratitis. Invest Opthalmol Vis Sci. 2010; 51: 3100–6.