Abstract
Extracellular vesicles (EVs) play an important role in cell-to-cell communication. Although there are different kinds of vesicles, each with their own secretion and capture biology, all of them carry a cargo of proteins, lipids, metabolites and nucleic acids. They act as vehicles for exchange of biological materials and signals and are involved in the regulation of various physiological processes. Liver is an essential organ containing different cell populations fulfilling various functions, which need to be strictly controlled and coordinated. There are a few articles showing the role of liver-derived EVs. On the basis of them, we present here a hypothesis of the implication of such vesicles in the physiology of the liver. Different liver cell types, including hepatocytes, cholangiocytes and stellate cells, secrete and capture EVs and interact with them. Liver injury changes the abundance and cargo of EVs; these changes are likely to be important for the outcome of stress response. Although a substantial effort has been put into the characterization of EVs in isolated populations, it is only recently that some more comprehensive information has begun to appear. In this article, we hypothesize about the role of EVs in liver microenvironment and their possible function using published data from both hepatic and non-hepatic systems.
Liver is a multifunctional organ involved in important metabolic functions, synthesis of blood components and storage of lipids and retinol Citation1. It is also responsible for the disposal of bacterial products taken up from the gut and detoxification of xenobiotics and drugs. To accomplish these tasks, the liver employs not just hepatocytes, but also other non-parenchymal immune and non-immune cells. The resident liver tissue macrophages (Kupffer cells), natural killer (NK) cells, T cells and B cells are all the members of the hepatic immune system. These cell types strictly regulate the immune system in liver and are important mediators in inflammation Citation2. Among non-immune cells, the hepatic stellate cells, also known as Ito cells, are involved in angiogenesis Citation3 and fibrosis processes. The parenchyma of liver consists mostly of hepatocytes, which are polarized cells with important roles in detoxification, production and clearance of blood components, and in the formation of bile.
All these cellular populations, with their diverse physiological processes, have to be strictly coordinated to perform their functions properly. Recently, the role of secreted extracellular vesicles in the exchange of proteins, nucleic acids and lipids has awakened some considerable interest in the field of intercellular signalling Citation4. Two main groups of secreted vesicles have been identified: endosome-derived vesicles named exosomes and plasma membrane-shed vesicles called ectosomes or microparticles Citation5. Here we refer to both as extracellular vesicles (EVs), following the nomenclature adopted by the International Society of Extracellular Vesicles (http://www.isevmeeting.org). However, we also provide the type of EVs, if the information is available.
There have been just few studies focusing on the role of circulating liver-derived EVs, or the possible effects that non-hepatic EVs have on liver. Supported by those published articles, and extrapolating information obtained from non-liver systems, we offer here a collection of possible scenarios where EVs may be important for liver functionality.
Several reports (Citation6–Citation8) have identified and characterized the role of EVs in intercellular communications; 2 fundamental mechanisms have been proposed. First, the vesicle membranes can interact with receptors of the targeted cell, triggering signal pathways. Some of the EV membrane proteins cannot be identified on the surface of the donor cell, and activate a signalling path different to that employed during direct cell contact Citation9. Second, EVs can be internalized Citation4, and their cargo, including proteins and various types of nucleic acids (such as mRNA and miRNA), can be then released Citation7 Citation10.
Remarkably, the composition of EVs depends on the particular cell state Citation11; their extracellular localization makes them an ideal research target in the field of biomarker discovery Citation12 Citation13. Identification of molecular markers for early detection and prognosis of liver conditions is one of the main tasks in hepatology. Currently, diagnosis of liver diseases relies mainly on histological examination of liver biopsies. However, liver biopsy is invasive and its practical applications are limited by sampling errors, low diagnostic accuracy and hazard to the patient Citation14 Citation15. Biopsy is often used late in the disease progress and the lesions might be irreversible in many cases. These concerns have been fuelling the search for novel non-invasive markers for the diseases associated with liver injury, such as steatosis, hepatitis, fibrosis, apoptosis, necrosis and cancer cell proliferation. The isolation and characterization of liver-derived EVs in blood samples could shed some new light on various liver physiological processes Citation5. Our aim here is to hypothesize about the role of EVs and their components in the hepatic environment, both in health and disease ( and ).
Fig. 1. Liver cells are able to release and capture EVs. The small circles represent EVs released from different cells. Green corresponds to cholangiocytes, red to blood-circulating EVs released from other tissues, yellow to stellate cells, violet to tumoral cells, pink to hepatocytes and brown to immune cells. For references, see text and .
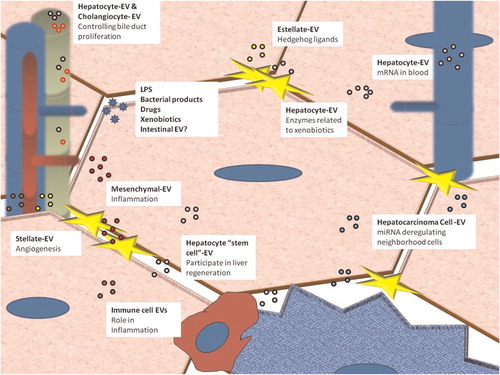
Table I. EV-secreting liver cell types; different studies show the properties and relevance of the EVs’ cargo for each cell type
EVs related to drug metabolism
Previously, we have reported that non-tumoral liver-derived cell lines such as MLP29 and primary cultured hepatocytes are able to secrete EVs Citation16. A comprehensive proteomic study of EVs released by primary hepatocytes has identified several members of cytochrome P450, uridinediphosphate-glucuronosyl-transferase (UGT) and glutathione S-transferase (GST) protein families Citation17. The proteomics data were validated on fractions obtained by employing a sucrose density gradient thereby demonstrating the vesicle-associated nature of the proteins. The presence of hepatocyte-derived EVs in the bloodstream is indirectly supported by the fact that EVs purified from plasma contain the enzyme carbamoyl phosphate synthetase 1, which is highly expressed in the liver and to a lesser extent in the small intestine tissue Citation17 Citation18.
Our proteomic analysis of hepatocyte-derived EVs indicates that these vesicles contain several members of the P450, UGT and GST drug-metabolizing protein families. If hepatocyte-derived EVs would be released to the blood stream, it can be hypothesized that EVs might be involved in detoxification of drugs and endogenous toxic substances Citation13. Supporting this hypothesis, it has been already described that tumour cells employ EV-mediated transport to develop and confer chemotherapy resistance. Hence, P-glycoprotein is a transmembrane protein involved in multidrug resistance that is transferred via EVs from drug-resistant cancer cells to drug-sensitive cells Citation19. Moreover, cisplatin-resistant cell lines release this anti-tumoral drug and its transporters by using an EV-dependent mechanism, transferring the survival ability to acceptor cells (Citation20–Citation22).
Circulating liver-specific mRNA associated with EVs
Hepatocyte-derived EVs contain mRNA Citation23, and liver-specific mRNAs associated with EVs are present in the bloodstream Citation24. Blood samples obtained after galactosamine-induced liver damage in animal models show increased levels of liver-specific mRNAs associated with EVs and cell debris. For detecting liver damage, amplification and quantification of these nucleic acids in blood samples have proved to be more sensitive than traditional transaminase activity quantification Citation24. As the activity of transaminase enzymes increases in some other conditions, such as muscle injury Citation25 Citation26, mRNA quantification methods are also more specific Citation24. Assessment of circulating albumin mRNA levels as a measure of liver damage has been used in a chronic and mild fibrosis model, and has also been shown to be more sensitive than transaminase activity measurements Citation27. However, that study has not determined whether the albumin mRNA was associated with EVs.
In general, the role of bloodstream mRNAs is still unclear. Since the first descriptions of mRNA associated to EVs, functional transfer of EV-associated RNA to the acceptor cells has been shown Citation10. However, to prove unequivocally that the mRNA present in the EVs finally is translated into a functional protein by the acceptor cells, it has required plasmid overexpression of green fluorescence protein (GFP) mRNA in EV-producing cells and resulting GFP expression in the acceptor cell Citation28. Furthermore, it has been demonstrated that bone marrow cells co-cultured with liver cells, separated by a cell-impermeable membrane, express mRNA for albumin Citation29 Citation30. These data suggest that EVs both deliver mRNA to bone marrow cells and mediate the transcription of tissue-specific mRNA although more investigations in this area are clearly needed.
Although it has been suggested that the role of EVs is to protect RNA from RNase activity, the available data are controversial. The first description of RNA in exosomes has shown that mRNA is protected against RNase activity Citation10. However, RNase treatment of EVs preparations has also been employed as a proof that the EV-dependent phenotype is mediated by an RNA component Citation23 Citation28. One possible interpretation of these results is that different EVs confer different degree of protection. However, it is also plausible that certain RNAs might be located outside the vesicle lumen as associated membrane complexes. It is important to note that these studies employed different doses of RNase and very different times of treatment; it is also not clear whether fresh or frozen samples were used.
EV pathway for virus propagation
Hepatitis C virus (HCV) is a major cause of chronic liver disease, with about 170 million people infected worldwide Citation31. The HCV uses its envelope glycoproteins E1 and E2 to bind specific receptors such as CD81, claudin-1 and the class B member I scavenger receptor in the host cells Citation32 Citation33. HCV entry involves an additional clathrin-mediated post-internalization step and delivery into early endosomes Citation34. However, it is not clear how the assembled HCV virion is released from the cytoplasm. Extensive research on human immunodeficiency virus (HIV) has shown that the ESCRT (endosomal sorting complex required for transport) machinery plays a role in virion assembly Citation35. This machinery is also fundamental in the synthesis and sorting of exosomal cargo Citation36 Citation37. In HCV-infected patients, viral RNA is associated with EVs in plasma Citation38. In addition, the HRS protein, involved in the autophagic pathway Citation39, is required for exosome secretion, and plays an important role in HCV release (Citation38–Citation40). Improving our knowledge of EVs’ involvement in viral propagation could be useful in the development of new therapies against viral infections.
EVs in regeneration and differentiation
Liver is involved in detoxification of xenobiotics and noxious endobiotics, and therefore continuously exposed to injuries; tissue regeneration is an important defensive mechanism maintaining the viability of this organ. In acute liver injury, hepatic regeneration is not unlike a physiological wound-healing process, bringing about transient and reversible changes in the extracellular matrix of the organ and in the proliferative capacity of the hepatocytes Citation41 Citation42.
When administered in vivo, EVs derived from a subpopulation of human pluripotent resident liver cells accelerate the morphological and functional recovery of liver in hepatectomized rats Citation23. This effect is lost when EVs are treated with RNase, suggesting that RNA is involved in the process Citation23. Stem cells also use a horizontal transfer of mRNA to redirect the behaviour of differentiated cells Citation28 Citation43, and EVs participate in the cross-talk between stem and differentiated cells Citation44.
Protein cargo also plays an important role in response to EVs. Cholangiocytes and myofibroblastic hepatic stellate cells release EVs containing active hedgehog ligands in response to platelet-derived growth factor Citation45. In the acceptor cells, these EVs activate hedgehog signals that might stimulate angiogenesis Citation45.
EVs and cholangiocytes
Recently, it has been reported that cholangiocytes can secrete EVs Citation45. Moreover, transmission electron microscopy observations show that EVs present in the bile duct interact with the primary cilia of cholangiocytes Citation46. In experimental models of bile duct-ligated rats, biliary exosomes have been detected in vivo Citation45.
Biliary EVs secreted by cholangiocytes take part in the inactivation of ERK kinase signalling Citation47. This pathway is associated with the inhibition of cholangiocyte proliferation. Interestingly, a decrease in the ratio of phospho-ERK to total ERK correlates with the activation of miR-15a transcription in cholangiocytes treated with EVs. Inhibition of this miRNA also enhances cholangiocyte proliferation Citation48. However, the specific composition of cholangiocyte-derived EVs that mediate this effect remains unknown.
EVs in liver inflammation
In developed countries, non-alcoholic fatty liver disease is emerging as a major global liver disorder with the background of an increasing prevalence of obesity and type 2 diabetes Citation49. Non-alcoholic fatty liver disorder encompasses a spectrum of diseases from simple steatosis through steatohepatitis to fibrosis, and ultimately cirrhosis and hepatocellular carcinoma. Hepatocellular carcinoma is the fifth most common cancer worldwide and the third most common cause of cancer mortality Citation50.
The role of EVs in inflammation is ambiguous because EVs can elicit either inflammation Citation51, or immunosuppressive effects Citation52 Citation53. In shock patients, circulating EVs increase oxidative stress in liver Citation54. Notably, hepatocyte death induced by chronic oxidative stress and inflammation triggers a potent regenerative response in attempt to restore the hepatic parenchyma Citation41. It has been shown that certain EVs can act as antigen vehicle Citation55. In cases of liver injury there is a large number of circulating EVs Citation24 Citation56 that contain liver-specific enzymes Citation57. Therefore, the possible involvement of EVs in autoimmune hepatitis should not be rejected Citation17. Aberrant activation of innate immune signalling may trigger “harmful inflammation” that contributes to sepsis, chronic inflammation, autoimmune diseases, tissue and organ injuries, fibrosis and carcinogenesis Citation2. Disruption of the intestinal epithelial barrier results in a leaky gut, which causes bacterial translocation and the appearance of bacterial products, such as lipopolysaccharides and toxins, in the liver Citation2 Citation58 Citation59. A number of obesity-related factors have been proposed as stimuli that activate the Toll-like receptors (TLR) pathway Citation60. In a fatty-liver rat model caused by high fatty diet, EVs isolated from peripheral blood were able to activate CD11b+ cells with subsequent homing to and accumulation of the cells in the liver Citation61.
EVs’ miRNA and cancer
Tumoral cells undergo deregulation of miRNAs expression Citation62 and are known to release miRNAs associated with EVs Citation12 Citation63 Citation64. An exhaustive catalogue of miRNAs present in EVs released by hepatic cancer cells has pointed towards TAK1 protein as one of the central targets of tumour-derived EV miRNA Citation65. TAK1 is an essential inhibitor of hepatocarcinogenesis, and the lack of TAK1 in vivo is associated with the spontaneous development of hepatocellular carcinoma as a result of aberrant responses to inflammatory and stress signalling Citation66 Citation67. TAK1 can also have a direct effect on cancer progression through repression of the telomerase reverse transcriptase gene Citation68. The aberrant expression of specific miRNAs in tumour cells and the ability of miRNAs to modulate multiple oncogenic or tumour suppressor genes make them well suited for such a role Citation65.
The objective is the network
shows a comprehensive summary of the possible roles of EVs secreted by liver cells. However, EVs derived from certain cell types have not been described; there is no data on hepatic sinusoidal cells, which play an important role in the cross-talk and paracrine regulation of lymphocytes and hepatocytes Citation69 Citation70. Further research will be required for in vivo isolation and characterization of other liver-derived EVs.
The appropriate regulation of the components present in any microenvironment is crucial to the stress response. As we have mentioned before, obesity causes leucocyte accumulation Citation71. Leucocyte EVs might trigger a response that activates immune cells which in turn rise the levels of interleukins Citation61. Oxidative stress induces hepatocyte damage Citation41 and the amount of liver-derived EVs probably increases Citation24 Citation56. EVs derived from liver resident pluripotent cells could induce regenerative signals Citation23. Hypothetically, liver-derived EVs might be captured by antigen-presenting cells Citation55 in the bone marrow and perhaps trigger an autoimmune response causing severe liver damage and inflammation. Steatohepatitis and fibrosis favour hepatocarcinogenesis and, in a final step of our hypothetical model, tumoural cells would release miRNA-containing EVs that deregulate the neighbouring cells Citation65 Citation66.
Maintaining appropriate signal balance may be crucial for the resolution of liver injuries. In a multicellular environment, EVs from different cell types, carrying different cargos, might interact with parenchymal cells. It is likely that liver dysfunction affects the composition of EVs’ cargo, making those vesicles invaluable in the damage assessment using plasma analysis. From another point of view, the ability of liver cells to uptake circulating EVs makes them a silver bullet in gene and miRNA therapy. The challenge is to decode the EVs’ network and find the tools to control it.
Conflict of interest and funding
The authors have not received any funding or benefits from industry or elsewhere to conduct this study.
Acknowledgements
We thank Drs So Young Lee, Esperanza Gonzalez and Ewa Gubb for a critical reading of the manuscript. This work was supported by grants from “Fondo de Investigaciones Sanitarias” (Institute of Health Carlos III, 06/0621 and PS09/00526 to J.M.F.P.) and Program “Ramon y Cajal” of Spanish Health Ministry (to J.M.F.P). “Centro de Investigación Biomédica en Red en el Área temática de Enfermedades Hepáticas y Digestivas” (CIBERehd) is funded by “Fondo de Investigaciones Sanitarias” (Institute of Health Carlos III).
References
- Shirakami Y, Lee SA, Clugston RD, Blaner WS. Hepatic metabolism of retinoids and disease associations. 2012, 1821: 124-36. 10.3402/jev.v1i0.18825.
- Seki E, Brenner DA. Toll-like receptors and adaptor molecules in liver disease: update. 2008, 48: 322-35. 10.3402/jev.v1i0.18825.
- Moreira RK. Hepatic stellate cells and liver fibrosis. 2007, 131: 1728-34.
- Mathivanan S, Ji H, Simpson RJ. Exosomes: extracellular organelles important in intercellular communication. 2010, 73: 1907-20. 10.3402/jev.v1i0.18825.
- Falcon-Perez JM, Lu SC, Mato JM. Sub-proteome approach to the knowledge of liver. 2010, 4: 407-15. 10.3402/jev.v1i0.18825.
- Cocucci E, Racchetti G, Meldolesi J. Shedding microvesicles: artefacts no more. 2009, 19: 43-51. 10.3402/jev.v1i0.18825.
- Simons M, Raposo G. Exosomes – vesicular carriers for intercellular communication. 2009, 21: 575-81. 10.3402/jev.v1i0.18825.
- Bastida E, Ordinas A, Escolar G, Jamieson GA. Tissue factor in microvesicles shed from U87MG human glioblastoma cells induces coagulation, platelet aggregation, and thrombogenesis. 1984, 64: 177-84.
- Tan F, Zhang Y, Mi W, Wang J, Wei J, Cai Yet al.Enrichment of phosphopeptides by Fe3 + -immobilized magnetic nanoparticles for phosphoproteome analysis of the plasma membrane of mouse liver. 2008, 7: 1078-87. 10.3402/jev.v1i0.18825.
- Valadi H, Ekstrom K, Bossios A, Sjostrand M, Lee JJ, Lotvall JO. Exosome-mediated transfer of mRNAs and microRNAs is a novel mechanism of genetic exchange between cells. 2007, 9: 654-9. 10.3402/jev.v1i0.18825.
- Duijvesz D, Luider T, Bangma CH, Jenster G. Exosomes as biomarker treasure chests for prostate cancer. 2011, 59: 823-31. 10.3402/jev.v1i0.18825.
- Rabinowits G, Gercel-Taylor C, Day JM, Taylor DD, Kloecker GH. Exosomal microRNA: a diagnostic marker for lung cancer. 2009, 10: 42-6. 10.3402/jev.v1i0.18825.
- Conde-Vancells J, Rodriguez-Suarez E, Gonzalez E, Berisa A, Gil D, Embade Net al.Candidate biomarkers in exosome-like vesicles purified from rat and mouse urine samples. 2010, 4: 416-25. 10.3402/jev.v1i0.18825.
- Cadranel JF, Rufat P, Degos F. Practices of liver biopsy in France: results of a prospective nationwide survey. For the Group of Epidemiology of the French Association for the Study of the Liver (AFEF). 2000, 32: 477-81. 10.3402/jev.v1i0.18825.
- Ratziu V, Charlotte F, Heurtier A, Gombert S, Giral P, Bruckert Eet al.Sampling variability of liver biopsy in nonalcoholic fatty liver disease. 2005, 128: 1898-906. 10.3402/jev.v1i0.18825.
- Conde-Vancells J, Rodriguez-Suarez E, Embade N, Gil D, Matthiesen R, Valle Met al.Characterization and comprehensive proteome profiling of exosomes secreted by hepatocytes. 2008, 7: 5157-66. 10.3402/jev.v1i0.18825.
- Conde-Vancells J, Gonzalez E, Lu SC, Mato JM, Falcon-Perez JM. Overview of extracellular microvesicles in drug metabolism. 2010, 6: 543-54. 10.3402/jev.v1i0.18825.
- Butler SL, Dong H, Cardona D, Jia M, Zheng R, Zhu Het al.The antigen for Hep Par 1 antibody is the urea cycle enzyme carbamoyl phosphate synthetase 1. 2008, 88: 78-88. 10.3402/jev.v1i0.18825.
- Bebawy M, Combes V, Lee E, Jaiswal R, Gong J, Bonhoure Aet al.Membrane microparticles mediate transfer of P-glycoprotein to drug sensitive cancer cells. 2009, 23: 1643-9. 10.3402/jev.v1i0.18825.
- Safaei R, Larson BJ, Cheng TC, Gibson MA, Otani S, Naerdemann Wet al.Abnormal lysosomal trafficking and enhanced exosomal export of cisplatin in drug-resistant human ovarian carcinoma cells. 2005, 4: 1595-604. 10.3402/jev.v1i0.18825.
- Shedden K, Xie XT, Chandaroy P, Chang YT, Rosania GR. Expulsion of small molecules in vesicles shed by cancer cells: association with gene expression and chemosensitivity profiles. 2003, 63: 4331-7.
- Fleissner F, Goerzig Y, Haverich A, Thum T. Microvesicles as novel biomarkers and therapeutic targets in transplantation medicine. 2012, 12: 289-97. 10.3402/jev.v1i0.18825.
- Herrera MB, Fonsato V, Gatti S, Deregibus MC, Sordi A, Cantarella Det al.Human liver stem cell-derived microvesicles accelerate hepatic regeneration in hepatectomized rats. 2010, 14: 1605-18. 10.3402/jev.v1i0.18825.
- Wetmore BA, Brees DJ, Singh R, Watkins PB, Andersen ME, Loy Jet al.Quantitative analyses and transcriptomic profiling of circulating messenger RNAs as biomarkers of rat liver injury. 2010, 51: 2127-39. 10.3402/jev.v1i0.18825.
- Nathwani RA, Pais S, Reynolds TB, Kaplowitz N. Serum alanine aminotransferase in skeletal muscle diseases. 2005, 41: 380-2. 10.3402/jev.v1i0.18825.
- Nosaka K. Changes in serum enzyme activities after injection of bupivacaine into rat tibialis anterior. 1996, 81: 876-84.
- Kudo Y, Ochi T, Shimada H, Ogawa S, Shinjo K. Utility of plasma circulating mRNA as a marker to detect hepatic injury. 2008, 70: 993-5. 10.3402/jev.v1i0.18825.
- Deregibus MC, Cantaluppi V, Calogero R, Lo Iacono M, Tetta C, Biancone Let al.Endothelial progenitor cell derived microvesicles activate an angiogenic program in endothelial cells by a horizontal transfer of mRNA. 2007, 110: 2440-8. 10.3402/jev.v1i0.18825.
- Jang YY, Collector MI, Baylin SB, Diehl AM, Sharkis SJ. Hematopoietic stem cells convert into liver cells within days without fusion. 2004, 6: 532-9. 10.3402/jev.v1i0.18825.
- Aliotta JM, Pereira M, Johnson KW, de Paz N, Dooner MS, Puente Net al.Microvesicle entry into marrow cells mediates tissue-specific changes in mRNA by direct delivery of mRNA and induction of transcription. 2010, 38: 233-45. 10.3402/jev.v1i0.18825.
- Asselah T, Benhamou Y, Marcellin P. Protease and polymerase inhibitors for the treatment of hepatitis C. 2009, 29Suppl 157-67. 10.3402/jev.v1i0.18825.
- Evans MJ, von Hahn T, Tscherne DM, Syder AJ, Panis M, Wolk Bet al.Claudin-1 is a hepatitis C virus co-receptor required for a late step in entry. 2007, 446: 801-5. 10.3402/jev.v1i0.18825.
- Murray CL, Jones CT, Rice CM. Architects of assembly: roles of Flaviviridae non-structural proteins in virion morphogenesis. 2008, 6: 699-708. 10.3402/jev.v1i0.18825.
- Burlone ME, Budkowska A. Hepatitis C virus cell entry: role of lipoproteins and cellular receptors. 2009, 90Pt 51055-70. 10.3402/jev.v1i0.18825.
- Chen BJ, Lamb RA. Mechanisms for enveloped virus budding: can some viruses do without an ESCRT?. 2008, 372: 221-32. 10.3402/jev.v1i0.18825.
- Stoorvogel W, Kleijmeer MJ, Geuze HJ, Raposo G. The biogenesis and functions of exosomes. 2002, 3: 321-30. 10.3402/jev.v1i0.18825.
- Hanson PI, Shim S, Merrill SA. Cell biology of the ESCRT machinery. 2009, 21: 568-74. 10.3402/jev.v1i0.18825.
- Masciopinto F, Giovani C, Campagnoli S, Galli-Stampino L, Colombatto P, Brunetto Met al.Association of hepatitis C virus envelope proteins with exosomes. 2004, 34: 2834-42. 10.3402/jev.v1i0.18825.
- Tamai K, Tanaka N, Nara A, Yamamoto A, Nakagawa I, Yoshimori Tet al.Role of Hrs in maturation of autophagosomes in mammalian cells. 2007, 360: 721-7. 10.3402/jev.v1i0.18825.
- Tamai K, Shiina M, Tanaka N, Nakano T, Yamamoto A, Kondo Yet al.Regulation of hepatitis C virus secretion by the Hrs-dependent exosomal pathway. 2012, 422: 377-85. 10.3402/jev.v1i0.18825.
- Fausto N, Campbell JS, Riehle KJ. Liver regeneration. 2006, 432 Suppl 1S45-53. 10.3402/jev.v1i0.18825.
- Muddu AK, Guha IN, Elsharkawy AM, Mann DA. Resolving fibrosis in the diseased liver: translating the scientific promise to the clinic. 2007, 39: 695-714. 10.3402/jev.v1i0.18825.
- Ratajczak J, Miekus K, Kucia M, Zhang J, Reca R, Dvorak Pet al.Embryonic stem cell-derived microvesicles reprogram hematopoietic progenitors: evidence for horizontal transfer of mRNA and protein delivery. 2006, 20: 847-56. 10.3402/jev.v1i0.18825.
- Quesenberry PJ, Aliotta JM. The paradoxical dynamism of marrow stem cells: considerations of stem cells, niches, and microvesicles. 2008, 4: 137-47. 10.3402/jev.v1i0.18825.
- Witek RP, Yang L, Liu R, Jung Y, Omenetti A, Syn WKet al.Liver cell-derived microparticles activate hedgehog signaling and alter gene expression in hepatic endothelial cells. 2009, 136: 320-30.e2. 10.3402/jev.v1i0.18825.
- Huang BQ, Masyuk TV, Muff MA, Tietz PS, Masyuk AI, Larusso NF. Isolation and characterization of cholangiocyte primary cilia. 2006, 291: G500-9. 10.3402/jev.v1i0.18825.
- Masyuk AI, Huang BQ, Ward CJ, Gradilone SA, Banales JM, Masyuk TVet al.Biliary exosomes influence cholangiocyte regulatory mechanisms and proliferation through interaction with primary cilia. 2010, 299: G990-9. 10.3402/jev.v1i0.18825.
- Lee SO, Masyuk T, Splinter P, Banales JM, Masyuk A, Stroope Aet al.MicroRNA15a modulates expression of the cell-cycle regulator Cdc25A and affects hepatic cystogenesis in a rat model of polycystic kidney disease. 2008, 118: 3714-24. 10.3402/jev.v1i0.18825.
- Friedman SL. Liver fibrosis – from bench to bedside. 2003, 38Suppl 1S38-53. 10.3402/jev.v1i0.18825.
- Gines P, Cardenas A, Arroyo V, Rodes J. Management of cirrhosis and ascites. 2004, 350: 1646-54. 10.3402/jev.v1i0.18825.
- Thery C, Zitvogel L, Amigorena S. Exosomes: composition, biogenesis and function. 2002, 2: 569-79.
- Thery C, Duban L, Segura E, Veron P, Lantz O, Amigorena S. Indirect activation of naive CD4+ T cells by dendritic cell-derived exosomes. 2002, 3: 1156-62. 10.3402/jev.v1i0.18825.
- Sadallah S, Eken C, Schifferli JA. Ectosomes as modulators of inflammation and immunity. 2011, 163: 26-32. 10.3402/jev.v1i0.18825.
- Mastronardi ML, Mostefai HA, Meziani F, Martinez MC, Asfar P, Andriantsitohaina R. Circulating microparticles from septic shock patients exert differential tissue expression of enzymes related to inflammation and oxidative stress. 2011, 39: 1739-48. 10.3402/jev.v1i0.18825.
- Wolfers J, Lozier A, Raposo G, Regnault A, Thery C, Masurier Cet al.Tumor-derived exosomes are a source of shared tumor rejection antigens for CTL cross-priming. 2001, 7: 297-303. 10.3402/jev.v1i0.18825.
- Brodsky SV, Facciuto ME, Heydt D, Chen J, Islam HK, Kajstura Met al.Dynamics of circulating microparticles in liver transplant patients. 2008, 17: 261-8.
- Locke JA, Fazli L, Adomat H, Smyl J, Weins K, Lubik AAet al.A novel communication role for CYP17A1 in the progression of castration-resistant prostate cancer. 2009, 69: 928-37. 10.3402/jev.v1i0.18825.
- Crispe IN. The liver as a lymphoid organ. 2009, 27: 147-63. 10.3402/jev.v1i0.18825.
- Pradere JP, Troeger JS, Dapito DH, Mencin AA, Schwabe RF. Toll-like receptor 4 and hepatic fibrogenesis. 2010, 30: 232-44. 10.3402/jev.v1i0.18825.
- Vitseva OI, Tanriverdi K, Tchkonia TT, Kirkland JL, McDonnell ME, Apovian CMet al.Inducible Toll-like receptor and NF-kappaB regulatory pathway expression in human adipose tissue. 2008, 16: 932-7. 10.3402/jev.v1i0.18825.
- Deng ZB, Liu Y, Liu C, Xiang X, Wang J, Cheng Zet al.Immature myeloid cells induced by a high-fat diet contribute to liver inflammation. 2009, 50: 1412-20. 10.3402/jev.v1i0.18825.
- Croce CM. Causes and consequences of microRNA dysregulation in cancer. 2009, 10: 704-14. 10.3402/jev.v1i0.18825.
- Taylor DD, Gercel-Taylor C. MicroRNA signatures of tumor-derived exosomes as diagnostic biomarkers of ovarian cancer. 2008, 110: 13-21. 10.3402/jev.v1i0.18825.
- Skog J, Wurdinger T, van Rijn S, Meijer DH, Gainche L, Sena-Esteves Met al.Glioblastoma microvesicles transport RNA and proteins that promote tumour growth and provide diagnostic biomarkers. 2008, 10: 1470-6. 10.3402/jev.v1i0.18825.
- Kogure T, Lin WL, Yan IK, Braconi C, Patel T. Intercellular nanovesicle-mediated microRNA transfer: a mechanism of environmental modulation of hepatocellular cancer cell growth. 2011, 54: 1237-48. 10.3402/jev.v1i0.18825.
- Inokuchi S, Aoyama T, Miura K, Osterreicher CH, Kodama Y, Miyai Ket al.Disruption of TAK1 in hepatocytes causes hepatic injury, inflammation, fibrosis, and carcinogenesis. 2010, 107: 844-9. 10.3402/jev.v1i0.18825.
- Malato Y, Willenbring H. The MAP3K TAK1: a chock block to liver cancer formation. 2010, 52: 1506-9. 10.3402/jev.v1i0.18825.
- Fujiki T, Miura T, Maura M, Shiraishi H, Nishimura S, Imada Yet al.TAK1 represses transcription of the human telomerase reverse transcriptase gene. 2007, 26: 5258-66. 10.3402/jev.v1i0.18825.
- Shetty S, Lalor PF, Adams DH. Lymphocyte recruitment to the liver: molecular insights into the pathogenesis of liver injury and hepatitis. 2008, 254: 136-46. 10.3402/jev.v1i0.18825.
- Edwards S, Lalor PF, Nash GB, Rainger GE, Adams DH. Lymphocyte traffic through sinusoidal endothelial cells is regulated by hepatocytes. 2005, 41: 451-9. 10.3402/jev.v1i0.18825.
- van der Poorten D, Milner KL, Hui J, Hodge A, Trenell MI, Kench JGet al.Visceral fat: a key mediator of steatohepatitis in metabolic liver disease. 2008, 48: 449-57. 10.3402/jev.v1i0.18825.